This month we’ll begin to examine brand new information on effects of lighting (both intensity and spectral quality) and how it affects coral coloration. In addition, we’ll piece together some information from various research papers, and parts of the coral pigmentation puzzle will begin to fall in place.
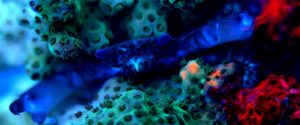
A coral crab jealously guards it cyan-colored Pocillopora damicornis host. Note the red fluorescence of the algae growing on the live rock to the right, and how the coral’s blue-green and the red fluorescence of the algae combine to make the coral appear orange. Photo by the author, 10-second exposure at f/32 using a black light as an excitation source.
It is very unusual for important research papers on coral coloration to appear simultaneously, yet this happened in the later portion of 2008. Together, results of efforts by researchers (Alieva et al., 2008, D’Angelo et al., 2008, Feldman et al., 2008, Gruber et al., 2008, Vogt et al., 2008), along with others’ (Oswald et al., 2007, Schlacher et al., 2007), offer fascinating glimpses into the mysterious world of coral coloration, with insights offered on types of pigments and how we, as hobbyists, can manipulate the environments of captive corals and induce coloration.
The goals of these articles are to enable the hobbyist to visually identify the color bandwidth of a particular pigment and, once identified, categorize the pigment and apply information provided on light intensity and light color to induce, or maintain, coral coloration.
We should note that a number of factors are involved when making corals colorful. Light, of course, is critical, but the importance of this parameter should not diminish the criticality of other co-factors such as water motion, pH, alkalinity, nutrient loadings, etc. However, it can not be denied that, when all other conditions are correct, light can literally make or break colorful pigmentation.
In situations where the pigment can gain or lose coloration, light energy can bend the shape of the pigment molecule (called the ‘hula twist’), sometimes resulting in coloration (photoconversion) or coloration loss (photobleaching). When the light energy falls below a certain threshold level (either intensity or spectral quality), the pigment molecule ‘relaxes’ to its normal state.
In some cases, light energy breaks the molecular bond(s) completely, leading to an irreversible change in apparent coloration.
This month, we’ll examine pigment clades, and effects of light quantity and quality on the blue-green (cyan) fluorescent pigments. But first, a review of terminology used in this and following articles is in order.
Glossary
The following terms will be used in this article:
- Absorbance:
- Ability of a solution or layer of a substance to retain light without reflection or transmission.
- Absorption:
- The process in which incident radiation is retained without reflection or transmission.
- Brightness:
- The intensity of a fluorescent emission. Extinction coefficient times Quantum Yield = Brightness.
- Clade:
- For our purposes in this article, a grouping of pigments based on similar features inherited from a common ancestor. Pigments from corals includes Clades A, B, C, and D. Clades can refer to living organisms as well (clades of Symbiodinium – zooxanthellae – are a good example.)
- Chromophore:
- The colorful portion of a pigment molecule. In some cases, chromophore refers to a granular packet containing many pigment molecules.
- Chromoprotein pigment:
- A non-fluorescent but colorful pigment. These pigments appear colorful because they reflect light. For example, a chromoprotein with a maximum absorption at 580nm might appear purple because it preferentially reflects blue and red wavelengths.
- Chromo-Red Pigment:
- A newly described type of pigment possessing characteristics of both chromoproteins and Ds-Red fluorescent proteins. Peak fluorescence is at 609nm (super red).
- Cyan Fluorescent Protein (CFP):
- Blue-green pigments with fluorescent emissions in the range of ~477-500nm. Cyan and green pigments share a similar chromophore structure. Cyan pigments are expressed at lower light levels than green, red or non-fluorescent pigments.
- Emission:
- That light which is fluoresced by a fluorescent pigment.
- Extinction Coefficient:
- The quantity of light absorbed by a protein under a specific set of circumstances.
- Excitation:
- That light absorbed by a fluorescent pigment. Some of the excitation light is fluoresced or emitted at a less energetic wavelength (color).
- Ds-Red type pigment:
- A type of red fluorescent pigment with a single primary emission bandwidth at 574-620nm. Originally found in the false coral Discosoma.
- Fluorescence:
- Absorption of radiation at one wavelength (or color) and emission at another wavelength (color). Absorption is also called excitation. Fluorescence ends very soon after the excitation source is removed (on the order of ~2-3 nanoseconds: Salih and Cox, 2006).
- Green Fluorescent Protein (GFP):
- Fluorescent pigments with emissions of 500-525nm.
- ‘Hula Twist’:
- A bending of a pigment molecule resulting in a change of apparent color. Molecular bonds are not broken; therefore the pigment can shift back and forth, with movements reminiscent of a hula dancer.
- Kaede-type pigment:
- A type of red fluorescent pigment with a characteristic primary emission at ~574-580nm and a secondary (shoulder) emission at ~630nm. Originally found in the stony coral Trachyphyllia geoffroyi, but common in corals of suborder Faviina.
- Kindling Protein:
- A protein capable of being converted from a non-fluorescent chromoprotein to a fluorescent protein. Sometimes called a ‘Kindling Fluorescent Protein’, or KFP.
- Quantum Yield:
- Amount of that energy absorbed which is fluoresced. If 100 photons are absorbed, and 50 are fluoresced, the quantum yield is 0.50.
- Photobleaching:
- Some pigments, such as Dronpa, loss fluorescence if exposed to strong light (in this case, initially appearing green and bleaching to a non-fluorescent state when exposed to blue-green light). Photobleaching can obviously cause drastic changes in apparent fluorescence. In cases where multiple pigments are involved, the loss of fluorescence (or energy transfer from a donor pigment to an acceptor pigment) could also result in dramatic shifts in apparent color.
- Photoconversion:
- A rearrangement of the chemical structure of a colorful protein by light. Depending upon the protein, photoconversion can increase or decrease fluorescence (in processes called photoactivation and photobleaching, respectively). Photoconversion can break proteins’ molecular bonds (as with Kaede and Eos fluorescent pigments) resulting in an irreversible color shift, or the molecule can be ‘twisted’ by light energy (a ‘hula twist’) where coloration reversal are possible depending upon the quality or quantity of light available. This process is known as photoswitching).
- Red Fluorescent Protein (RFP):
- Those pigments with an emission of ~570nm and above. Includes Ds-Red, Kaede and Chromo-Red pigments.
- Stokes Shift:
- The difference in the maximum wavelength of fluorescent pigment excitation light and the maximum wavelength of the fluoresced light (emission). For example, a pigment with an excitation wavelength of 508nm and an emission wavelength of 535nm would have a Stokes Shift of 27nm.
- Threshold or Coloration Threshold:
- The point at which pigment production is sufficient to make its fluorescence (or in the case of non-fluorescent chromoproteins, it absorption) visually apparent. The term threshold generally refers pigment production, although, in some cases, it could apply to a light level where a pigment disappears (as in the cases of photobleaching, or photoconversion).
- Yellow Fluorescent Protein:
- An uncommon group of fluorescent proteins with emissions in the 525-570 nm range (Alieva et al., 2008).
Types of Pigments
There are at least 9 described types of coral pigments. Note: Pigment types, such as green or red might not be structurally similar to another green or red pigment in a different clade – see below). These include:
- Cyan Fluorescent Proteins (CFP) – Cyan pigments are blue-green pigments with a maximum emission of up to ~500 nm. The chromophore structure of a cyan pigment is very similar to that of a green fluorescent pigment.
- Green Fluorescent Proteins (GFP) – This group, by far, is the most numerous of the fluorescent proteins. The structure of green fluorescent chromophores is very similar to that of cyan fluorescent chromophores.
- Yellow Fluorescent Proteins (YFP) – An unusual type of fluorescent protein with maximum emission in the yellow portion of the spectrum. Rare in its biological distribution, YFP is found in a zoanthid and some specimens of the stony coral Agaricia. Personally, I’ve noted yellow fluorescence in a very few stony corals (Porites specimens) here in Hawaii while on night dives using specialized equipment to observe such colorations (see www.nightsea.com for details on this equipment).
- Orange Fluorescent Protein (OFP) – I’ve included this protein ‘type’ in an attempt to avoid confusion. OFP is used to describe a pigment found in stony coral Lobophyllia hemprichii and its name suggests a rather unique sort of protein. In fact, OFP is simply a variant of the Kaede-type fluorescent proteins.
- Red Fluorescent Proteins (RFP) – A group of proteins including several different subtypes (Kaede, Ds-Red and Chromo-Red). Typically, fluorescent emission is in the range of ~580 nm to slightly over 600 nm.
- Dronpa – A green fluorescent protein that loses fluorescent when exposed to blue-green light (~490 nm) but returns when irradiated with violet light at ~400 nm.
- Kindling Proteins – A protein (notably from the anemone Anemonia sculata) that changes from a non-fluorescent pigment to one demonstrating fluorescence. This change is switchable/reversible and its state depends upon the spectral quality of light striking it.
- Chromo-Red Proteins – A new classification (Alieva et al., 2008) of a single fluorescent pigment found in the stony coral Echinophyllia. This chromo-red pigment has qualities of a non-fluorescent chromoprotein, but fluoresces at a maximum of 609 nm.
- Chromoproteins (CP) – This group of pigments is non-fluorescent, or has minimal fluorescence (where the quantum yield is essentially zero). Instead of relying upon fluorescence for coloration, these pigments instead absorb light most strongly in a relatively narrow portion of the visible spectrum. Most coral chromoproteins absorb light maximally at 560-593 nm. There are reports of anemones absorbing light at a maximum wavelength of 610nm, and a couple of reports of stony corals absorbing wavelengths in the 480-500nm range. Some chromoproteins are very similar in structure to the fluorescent Ds-Red proteins. In fact, genetic engineers have found that a single amino acid substitution in a protein can make the difference between non-fluorescence and fluorescence. Chromoproteins do not get much attention by researchers (relative to that of fluorescence proteins) and there are only about 40 described.
Pigment Clades
Examination of pigment clades might seem a fruitless endeavor, but, to the contrary, there is some interesting information to be garnered. Working on the hypothesis that pigment clades have evolved over the millennia and could have specific within-clade properties, we can possibly use cladal data, along with other information, to form loose categories as to how pigments respond to various stimuli, including light and perhaps other factors. It is interesting to note that some pigments seem to have evolved within certain groups of animals (Clade A in anemones, or Clade D’s Kaede-type pigments found mostly in stony corals of suborder Faviina).
Pigment Names
I’ve attempted to list as much of the shorthand names for coral pigments within the following Tables 1 through 4. It is becoming common for researchers to use a pigment’s given name (such as mcav4, Eos, or r1.2) without providing further information, and this information was included in the following Tables for my convenience, although any serious student not familiar with these names can also benefit.
There are currently 4 major pigment clades, with Clade C containing several subgroups. We begin our study with:
Clade A
Consists entirely (at present) of anemones (Actinaria genera) and contains green and red fluorescent variants, along with a number of non-fluorescent chromoproteins. No cyan or yellow fluorescent pigments are included. See Table 1. Evidence suggests that photoconversion is possible in at least some fluorescent pigments of Clade A, and a transition from a non-fluorescent state to one of fluorescence is possible in the ‘kindling’ protein from Anemonia sculata (that transforms from a non-fluorescent protein with maximum absorption at 562 nm to a fluorescence state with maximum emission at 595 nm upon exposure to strong light).
Species | Name | Type | Order | Excitation | Em/Abs | Clade |
---|---|---|---|---|---|---|
Anemonia equina | CP-597 | Chromoprotein | Actinaria | n/a | 597 | A |
Anemonia sculata | CP-568 | Chromoprotein | Actinaria | n/a | 568 | A |
Anemonia sculata | CP-575 | Chromoprotein | Actinaria | n/a | 575 | A |
Anemonia sculata | CP-595 | Chromoprotein (KPF1) | Actinaria | n/a | 595 | A |
Anemonia sculata | CP-562 | Chromoprotein | Actinaria | n/a | 562 | A |
Cnidopis japonica | CP-610 | Chromoprotein | Actinaria | n/a | 610 | A |
Condylactis gigantea | CP-571 | Chromoprotein | Actinaria | n/a | 571 | A |
Condylactis passiflora | CP-571 | Chromoprotein | Actinaria | n/a | 571 | A |
Heteractis crisperi | hmagnCP | Chromoprotein | Actinaria | n/a | 578 | A |
Rhizostoma anemone | CP-588 | Chromoprotein | Actinaria | n/a | 588 | A |
Entacmaea quadricolor | eqFP611 | Ds-Red | Actinaria | 559 | 611 | A |
Anemonia sculata | asFP499 | GFP | Actinaria | ? | 499 | A |
Condylactis gigantea | cgigGFP | GFP | Actinaria | 399/482 | 496 | A |
Heteractis crisperi | hcriGFP | GFP | Actinaria | 405/481 | 500 | A |
Clade B
Clade B is currently populated by scleractinian non-fluorescent chromoproteins (including those found in Acropora, Montipora, Pocillopora, etc.), three red fluorescent pigments (from the stony corals Porites, Montipora, and the false coralDiscosoma), along with one cyan fluorescent pigment. At present, Clade B does not contain any green or yellow fluorescent pigments. See Table 2. Photoconversion occurs in many Clade B pigments, but is not common in the cyan pigments discussed in this article.
Taxon | Pigment Name | Type of Pigment | Order/Suborder | Excitation | Emission | Clade |
---|---|---|---|---|---|---|
Discosoma striata | P-583 | CFP | Corallimorpharia | 443 | 483 | B |
Acanthastrea | CP-579.5 | Chromoprotein | Faviina | n/a | 579.5 | B |
Acropora | CP-500 | Chromoprotein | Astrocoeiina | n/a | 500 | B |
Acropora | CP-586 | Chromoprotein | Astrocoeiina | n/a | 586 | B |
Acropora aculeus | aacuCP | Chromoprotein | Astrocoeiina | n/a | 580 | B |
Acropora aculeus | CP-580 | Chromoprotein | Astrocoeiina | n/a | 580 | B |
Acropora digitifera | CP-578 | Chromoprotein | Astrocoeiina | n/a | 578 | B |
Acropora formosa | CP-588 | Chromoprotein | Astrocoeiina | n/a | 588 | B |
Acropora hyacinthus | ahyaCP | Chromoprotein | Astrocoeiina | n/a | 580 | B |
Acropora millepora | amilCP | Chromoprotein | Astrocoeiina | n/a | 588 | B |
Acropora nobilis | CP-579 | Chromoprotein | Astrocoeiina | n/a | 579 | B |
Acropora nobilis | CP-583.5 | Chromoprotein | Astrocoeiina | n/a | 583.5 | B |
Acropora nobilis | CP-591 | Chromoprotein | Astrocoeiina | n/a | 591 | B |
Caulastrea sp. | CP-591.5 | Chromoprotein | Faviina | n/a | 591.5 | B |
Galaxea fascicularis | gfasCP | Chromoprotein | Meandriina | n/a | 577 | B |
Goniopora djiboutiensis | gdjiCP | Chromoprotein | Fungiina | n/a | 583 | B |
Goniopora tenuidens | gten | Chromoprotein | Fungiina | n/a | 580 | B |
Millepora (hydrocoral) | CP-579 | Chromoprotein | Capitata | n/a | 579 | B |
Montipora efflorescens | meffCP | Chromoprotein | Astrocoeiina | n/a | 574 | B |
Montipora efflorescens | CP-590 | Chromoprotein | Astrocoeiina | n/a | 590 | B |
Montipora sp. | CP-579.5 | Chromoprotein | Astrocoeiina | n/a | 579.5 | B |
Montipora sp. | CP-584 | Chromoprotein | Astrocoeiina | n/a | 584 | B |
Montipora sp. | CP-592 | Chromoprotein | Astrocoeiina | n/a | 592 | B |
Pocillopora sp. | CP-480 | Chromoprotein | Astrocoeiina | n/a | 480 | B |
Pocillopora damicornis | CP-560 | Chromoprotein | Astrocoeiina | n/a | 560 | B |
Pocillopora sp. | CP-593 | Chromoprotein | Astrocoeiina | n/a | 593 | B |
Porites murrayensis | CP-579.5 | Chromoprotein | Faviina | n/a | 579.5 | B |
Seriatopora hystrix | CP-560 | Chromoprotein | Astrocoeiina | n/a | 560 | B |
Stylocoeniella sp. | stylCP | Chromoprotein | Astrocoeiina | n/a | 574 | B |
Stylophora pistillata | spisCP | Chromoprotein | Astrocoeiina | n/a | 560 | B |
Discosoma sp. | Ds-Red | Ds-Red | Corallimorpharia | 558 | 583 | B |
Montipora sp. | Keima-Red | Ds-Red | Astrocoeiina | 440 | 620 | B |
Porites porites | pPorRFP | Ds-Red | Faviina | 578 | 595 | B |
Clade C, including Subclades C1, C2, and C3
This clade is the most diverse of the 4 clades currently described, and includes some of the cyan, green, yellow and red fluorescent proteins plus one non-fluorescent chromoprotein (a ‘red’ pocilloporan) from the stony coral Stylophora pistillata. Green fluorescent proteins are mostly from Acropora and Montipora species but also several from orders/suborders Meandriina, Fungiina and Faviina. See Table 3.
Taxon Pigment Name Type of Pigment Order/Suborder Excitation Emission Clade
Taxon | Pigment Name | Type of Pigment | Order/Suborder | Excitation | Emission | Clade |
---|---|---|---|---|---|---|
Acropora millepora | amilCFP | CFP | Astrocoeiina | 441 | 489 | C2 |
Acropora nobilis | anobCFP1 | CFP | Astrocoeiina | 462 | 490 | C2 |
Acropora nobilis | anobCFP2 | CFP | Astrocoeiina | 477 | 495 | C2 |
Anemonia majano | P-486 | CFP | Actinaria | 458 | 486 | C2 |
Eusmilia fastigata | efasCFP | CFP | Meandriina | 466 | 490 | C1 |
Montipora efflorescens | meffCFP | CFP | Astrocoeiina | 467 | 492 | C3 |
Montipora millepora | mmilCFP | CFP | Astrocoeiina | 404 | 492 | C3 |
Psammocora sp. | psamCFP | CFP | Faviina | 404 | 492 | C3 |
Acropora aculeus | aacuGFP1 | GFP | Astrocoeiina | 478 | 502 | C2 |
Acropora aculeus | aacuGFP2 | GFP | Astrocoeiina | 502 | 513 | C2 |
Acropora eurostoma | AeurGFP | GFP | Astrocoeiina | 504 | 515 | C2 |
Acropora millepora | amilGFP | GFP | Astrocoeiina | 503 | 512 | C2 |
Acropora nobilis | anobGFP | GFP | Astrocoeiina | 502 | 511 | C2 |
Eusmilia fastigata | efasGFP | GFP | Meandriina | 496 | 507 | C1 |
Meandrites meandrina | mmeanGFP | GFP | Faviina | 487 | 515 | C1 |
Montipora efflorescens | meffGFP | GFP | Astrocoeiina | 492 | 506 | C3 |
Porites porites | pporGFP | GFP | Faviina | 495 | 507 | C3 |
Stylocoeniella sp. | stylGFP | GFP | Astrocoeiina | 485 | 500 | C2 |
Acropora millepora | amilRFP | Ds-Red | Astrocoeiina | 560 | 593 | C3 |
Fungia concinna | Kusabira-Orange | Ds-Red | Fungiina | 548 | 561 | C1 |
Montipora digitata | mdigFP572 | Ds-Red | Astrocoeiina | 556 | 572 | C3 |
Montipora efflorescens | meffRFP | Ds-Red | Astrocoeiina | 560 | 576 | C3 |
Zoanthid sp. | Zoan2RFP | Ds-Red | Zoanthidae | 506 | 574 | C3 |
Zoanthid sp. | Zoan2RFP | Ds-Red | Zoanthidae | 552 | 576 | C* |
Zoanthus sp. | zoanGFP | Ds-Red | Zoanthidae | 496 | 506 | C3 |
Zoanthid sp. | zoanYFP | YFP | Zoanthidea | 528 | 538 | C3 |
Clade D
All pigments within this clade are fluorescent to some extent, and includes Ds-Red and Kaede-type orange/red fluorescent proteins (see Glossary). Clade D contains pigments found in at least two soft corals (Clavularia and Dendronephthya species), false corals (Discosoma and Ricordea), and many stony corals. Photoconversion is possible in many Clade D pigments. One green fluorescent pigment, Dronpa, bleaches upon exposure to ‘strong’ blue-green light. Interestingly, the majority of these pigments are from the stony coral suborder Faviina. See Table 4.
Taxon | Pigment Name | Type of Pigment | Order/Suborder | Excitation | Emission | Clade |
---|---|---|---|---|---|---|
Clavularia sp. | P-484 | CFP | Alcyonaria | 456 | 484 | D |
Montastraea cavernosa | P-486 | CFP | Faviina | 440 | 486 | D |
Mycedium elephantotus | meleCFP | CFP | Faviina | 454 | 485 | D |
Scolymia cubensis | scubCFP1 | CFP | Faviina | ? | 483 | D |
Scolymia cubensis | scubCFP2 | CFP | Faviina | ? | 484 | D |
Montastrea cavernosa | CP-486 | CFP | Faviina | 440 | 486 | D |
Montastrea cavernosa | mcav5 | CFP | Faviina | 435 | 495 | D |
Montastrea cavernosa | r5 | CFP | Faviina | ? | 495 | D |
Montastrea cavernosa | g5.1 | CFP | Faviina | ? | 495 | D |
Montastrea cavernosa | g5.2 | CFP | Faviina | ? | 495 | D |
Discosoma sp. | dis2RFP | Ds-Red | Corallimorpharia | 558 | 593 | D |
Agaricia fragilis | afraGFP | GFP | Fungiina | 494 | 503 | D |
Catalaphyllia jardineri | cjar | GFP | Faviina | 509 | 517 | D |
Dendronephthya sp. | dendGFP | GFP | Alcyonaria | 494 | 508 | D |
Discosoma sp. | dis3GFP | GFP | Corallimorpharia | 503 | 512 | D |
Echinophyllia echinata | eechGFP1 | GFP | Faviina | 497 | 510 | D |
Echinophyllia echinata | eechGFP2 | GFP | Faviina | 506 | 520 | D |
Echinophyllia echinata | eechGFP3 | GFP | Faviina | 512 | 524 | D |
Favites abdita | fabdGFP | GFP | Faviina | 508 | 520 | D |
Galaxea fascicularis | gfasGFP | GFP | Meandriina | 492 | 506 | D |
Galaxea fascicularis | Azami-Green | GFP | Meandriina | 492 | 505 | D |
Lobophyllia hemprichii | Eos | GFP | Faviina | 506 | 516 | D |
Lobophyllia hemprichii | lhemOFP | GFP | Faviina | 507 | 517 | D |
Montastraea annularis | monannGFP | GFP | Faviina | ? | 510 | D |
Montastraea cavernosa | mcavRFP | GFP | Faviina | 504 | 517 | D |
Montastraea cavernosa | mcavGFP | GFP | Faviina | 506 | 516 | D |
Montastraea cavernosa | P-510 | GFP | Faviina | 440 | 510 | D |
Montastraea cavernosa | mcav2 | GFP | Faviina | 505±3 | 515±3 | D |
Montastraea cavernosa | mcav3 | GFP | Faviina | 505±3 | 515±3 | D |
Montastraea cavernosa | mcav4 | GFP | Faviina | 505±3 | 515±3 | D |
Montastraea cavernosa | mcav6 | GFP | Faviina | 495 | 507 | D |
Montastraea cavernosa | r2 | GFP | Faviina | ? | 522 | D |
Montastraea cavernosa | r3 | GFP | Faviina | ? | 514 | D |
Montastraea cavernosa | r4 | GFP | Faviina | ? | 519 | D |
Montastraea cavernosa | r7 | GFP | Faviina | ? | 505 | D |
Montastraea cavernosa | g1.2 | GFP | Faviina | ? | 518 | D |
Montastraea cavernosa | g4 | GFP | Faviina | ? | 518 | D |
Montastraea cavernosa | g6 | GFP | Faviina | ? | 507 | D |
Montastraea faveolata | monfavGFP1 | GFP | Faviina | 440 | 510-520 | D |
Pectiniidae | Dronpa | GFP | Faviina | 503 | 518 | D |
Platygyra lamellina | plamGFP | GFP | Faviina | 502 | 514 | D |
Ricordea florida | P-510 | GFP | Corallimorpharia | ? | 510 | D |
Ricordea florida | P-513 | GFP | Corallimorpharia | ? | 513 | D |
Ricordea florida | P-517 | GFP | Corallimorpharia | 506 | 517 | D |
Ricordea florida | rfloGFP | GFP | Corallimorpharia | 508 | 518 | D |
Ricordea florida | P-520 | GFP | Corallimorpharia | ? | 520 | D |
Ricordea florida | P-573 | GFP | Corallimorpharia | ? | 573 | D |
Ricordea florida | rfloRFP | GFP | Corallimorpharia | 506 | 574 | D |
Sarcophyton sp. | sarcGFP | GFP | Octocorallia | 483 | 500 | D |
Scolymia cubensis | scubGFP1 | GFP | Faviina | 497 | 506 | D |
Scolymia cubensis | scubGFP2 | GFP | Faviina | 497 | 506 | D |
Trachyphyllia geoffroyi | P-518 | GFP | Faviina | 508 | 518 | D |
Catalaphyllia jardineri | cjar | Kaede | Faviina | 573 | 582 | D |
Dendronephthya sp. | Dendra | Kaede | Alcyonaria | 557 | 575 | D |
Echinophyllia echinata | eechRFP | Kaede | Faviina | 574 | 582 | D |
Favia favus | P-582 | Kaede | Faviina | 583 | 582 | D |
Favia speciosa | P-582 | Kaede | Faviina | 422 | 582 | D |
Lobophyllia hemprichii | EOS | Kaede | Faviina | 571 | 581 | D |
Lobophyllia hemprichii | lhemOFP | Kaede | Faviina | 543 | 574 | D |
Montastraea cavernosa | P-575 | Kaede | Faviina | 525 | 575 | D |
Montastraea cavernosa | mcav1 | Kaede | Faviina | 508 | 582 | D |
Montastraea cavernosa | r1.1 | Kaede | Faviina | ? | 582 | D |
Montastraea cavernosa | r1.2 | Kaede | Faviina | ? | 582 | D |
Montastraea cavernosa | g1.1 | Kaede | Faviina | ? | 582 | D |
Montastraea cavernosa | mcavRFP | Kaede | Faviina | 573 | 582 | D |
Montastraea cavernosa | mcavRFP | Kaede | Faviina | 508 | 520/580 | D |
Mycedium elephantotus | meleRFP | Kaede | Faviina | 573 | 579 | D |
Plesiastrea verispora | P-574 | Kaede | Faviina | 560 | 574 | D |
Ricordea florida | rfloRFP | Kaede | Corallimorpharia | ? | 576 | D |
Scolymia cubensis | scubRFP | Kaede | Faviina | 570 | 578 | D |
Trachyphyllia geoffroyi | Kaede | Kaede | Faviina | 558 | 582 | D |
Experimental Evidence of Light Intensity and Quality on Expression of Pigmentation by the Coral Host

Figure 1. Approximate transmission qualities of three colored filters used by D’Angelo et al. (2008).
A recent paper (D’Angelo et al., 2008) reports the results of experiments designed to investigate the effects of light intensity and light quality on expression of fluorescent pigments and non-fluorescent chromoproteins by various corals. The small-polyped stony corals used in these experiments were Acropora millepora, Acropora pulchra, Hydnophora grandis, Montipora digitata and Seriatopora hystrix. It appears that typographical errors mislabeled some of the pigments, but it seems that at least 11 coral pigments were examined (4 cyan fluorescent pigments, 3 green fluorescent pigments, 1 orange fluorescent pigment and 1 red fluorescent pigment. Two non-fluorescent chromoproteins were also studied).
Expression of coral coloration was examined with varying photon flux densities (PAR values classified as ‘very low’ (80 µmol·m²·sec), ‘low’ (100 µmol·m²·sec) ‘moderate’ (400 µmol·m²·sec), and ‘high’ (700 µmol·m²·sec). Photoperiod was 12 hours. Metal halide lamps producing ~50% of its visible radiation in the blue portion of the spectrum were used. See the Discussion section below for comments concerning how these categories relate to those seen in aquaria.
The effects of spectral quality were estimated by using commercially-available filters to separate the light generated by a metal halide lamp into 3 general categories. See Figure 1 for the approximate transmission qualities of these filters.

Figure 2. An unidentified Acropora specimen demonstrating blue-green fluorescence. The pigment is most likely of Clade C2. Photo by the author.
Unfortunately, we do not know the exact type of metal halide lamps used. We are told it is an ‘Aqua Light’ but no information is provided other than they ’emit ~50% of the photons in the blue spectral region.’ The lamps’ brand, kelvin rating, etc. would be of great use since it would allow us to better estimate the quality of light used to illuminate the corals. I’m tempted to suggest the lamps were Ushio’s ‘AquaLite’ metal halide lamp series, but lack any evidence other than similar names. Since metal halide lamps produce ‘spiky’ spectra we might expect to see these filters transmitting generous portions of relatively narrow bandwidths, while transmitting lesser, if any, amounts at other wavelengths. An email inquiry to the primary investigator has remained unanswered.
When using the blue, green and red filters, these researchers standardized the photon flux density to 200 µmol·m²·sec, but be aware that the green filter transmitted blue light as well (estimated to be 25% of the total transmitted light. In other words, the green filter transmitted at least 50 µmol·m²·sec of blue light). The red filter transmitted red wavelengths almost exclusively.
The experimental results of D’Angelo et al. offer fascinating insights on the effects of light intensity and spectral qualities on coral pigmentation. Some pigments require relatively little light while others are not expressed until light intensity reached a certain threshold. At least one pigment examined decreased in concentration upon exposure to increasing light intensity. By the same token, production of coral pigments by the coral animal can be a response to different colors of light and each pigment class seems to have a different reaction to ‘colored’ light.
Cyan Fluorescent Pigments
Cyan (from the Greek work kanos meaning dark blue) pigments are, for our purposes, a group of pigments with a fluorescent emission of up to a wavelength of 500 nanometers.

Figure 3. The beautiful blue-shifted cyan fluorescence of Discosoma specimens. This pigment is likely of Clade B.
Species | Pigment Name | Type | Suborder | Excit. | Emission | Clade |
---|---|---|---|---|---|---|
Acropora millepora | amilCFP | CFP | Astrocoeiina | 441 | 489 | C2 |
Acropora millepora | amilFP484 | CFP | Astrocoeiina | 420 | 484 | ? |
Acropora millepora | amilFP497 | CFP | Astrocoeiina | 477 | 497 | ? |
Acropora nobilis | anobCFP1 | CFP | Astrocoeiina | 462 | 490 | C2 |
Acropora nobilis | anobCFP2 | CFP | Astrocoeiina | 477 | 495 | C2 |
Acropora pulchra | apulFP486 | CFP | Astrocoeiina | 420 | 483 | ? |
Anemonia majano | P-486 | CFP | Actinaria | 458 | 486 | C2 |
Clavularia sp. | P-484 | CFP | Alcyonaria | 456 | 484 | D |
Discosoma striata | P-483 | CFP | Corallimorpharia | 443 | 483 | B |
Eusmilia fastigata | efasCFP | CFP | Meandriina | 466 | 490 | C1 |
Hydnophora grandis | hgraFP492 | CFP | Faviina | 443 | 492 | ? |
Montastraea cavernosa | P-486 | CFP | Faviina | 440 | 486 | D |
Montipora digitata | mdigFP486 | CFP | Astrocoeiina | 470 | 486 | ? |
Montipora efflorescens | meffCFP | CFP | Astrocoeiina | 467 | 492 | C3 |
Montipora millepora | mmilCFP | CFP | Astrocoeiina | 404 | 492 | C3 |
Mycedium elephantotus | meleCFP | CFP | Faviina | 454 | 485 | D |
Pocillopora damicornis | P-499 | CFP | Astrocoeiina | 484 | 499 | C2 |
Psammocora sp. | psamCFP | CFP | Faviina | 404 | 492 | C3 |
Scolymia cubensis | scubCFP1 | CFP | Faviina | ? | 483 | D |
Scolymia cubensis | scubCFP2 | CFP | Faviina | ? | 484 | D |
Montastrea cavernosa | CP-486 | CFP | Faviina | 440 | 486 | D |
Montastrea cavernosa | mcav5 | CFP | Faviina | 435 | 495 | D |
Montastrea cavernosa | r5 | CFP | Faviina | ? | 495 | D |
Montastrea cavernosa | g5.1 | CFP | Faviina | ? | 495 | D |
Montastrea cavernosa | g5.2 | CFP | Faviina | ? | 495 | D |
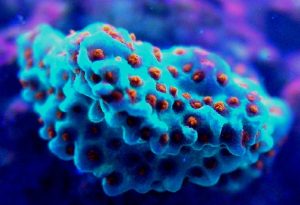
Figure 4. Blue-shifted cyan fluorescence of Montipora danae (the ‘Superman’ Montipora). This pigment probably belongs to Clade C3. The orange-red polyp fluorescence is peaking at 611 nm, and its classification is currently unknown. Photo by the author.
Cyan Fluorescent Pigment 483
This pigment from Acropora pulchra was found by D’Angelo et al., (2008) to be most notable at their ‘moderate’ light intensity category of 400 µmol·m²·sec. Increasing light intensity did not increase cyan fluorescence. CFP483 was also noted at lesser but significant concentrations at lower light intensities of 80 µmol·m²·sec and 100 µmol·m²·sec (see Figure 5). In their spectral quality experiments, these researchers found red light alone could promote the expression of this particular cyan pigment, although ‘green’ and blue light were more efficient in making the coral animal produce this coloration (see Figure 6).

Figure 5. Effects of light intensity (generated by a metal halide lamp) on the generation of fluorescent Pigment 483.
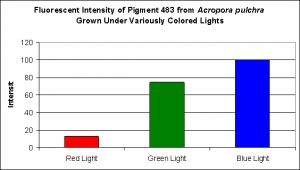
Figure 6. Effects of different colored lighting on expression of fluorescence of Pigment 483. Light intensity was 200 µmol·m²·sec for each treatment.
Cyan Fluorescent Pigment 484

Figure 7. The cyan fluorescence (along with red fluorescence) of Acropora millepora. Photo by the author.
This pigment, also found in an Acropora species (specifically A. millepora – see Figure 7) responds in a different fashion to light intensity and spectrum than CFP-483 (described above).
D’Angelo and her research partners found this particular pigment was found in increasing concentrations as light intensity rose from 80 µmol·m²·sec to 100 µmol·m²·sec and finally 400 µmol·m²·sec. Fluorescent intensity decreased when light intensity was increased to 700 µmol·m²·sec (see Figure 8). Red light was least efficient in promoting the production of Pigment 484 (see Figure 9).
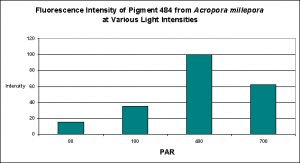
Figure 8. Effects of light intensity (generated by a metal halide lamp) on the generation of fluorescent Pigment 484.

Figure 9. Effects of different colored lighting on expression of fluorescence of Pigment 484. Light intensity was 200 µmol·m²·sec for each treatment.
Cyan Fluorescent Pigment 486

Figure 10. Effects of light intensity (generated by a metal halide lamp) on the generation of fluorescent Pigment 486.
As most hobbyists know, Montipora digitata specimens are available in quite a number of colors, ranging the common orange morph to the exotic multi-colored specimens. Figures 10 and 11 demonstrate this pigments production in relation to light intensity and light quality, respectively.
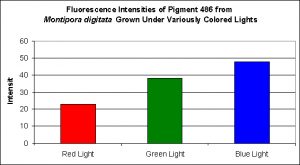
Figure 11. Effects of different colored lighting on expression of fluorescence of Pigment 486. Light intensity was 200 µmol·m²·sec for each treatment.
Cyan Fluorescent Pigment 492
The stony coral Hydnophora grandis contains this pigment (see Figure 12). Expression of this fluorescent pigment is seen a low light intensities of 80 and 100 µmol·m²·sec, with fluorescent pigmentation increasing to a maximum at 400 µmol·m²·sec, and then slightly falling when the coral is exposed to 700 µmol·m²·sec (see Figure 13). When examined for response to blue, green and red light, the least expression was noted under red light (although there is a considerable amount of fluorescence), while green and blue light were better at promoting fluorescent pigment production (see Figure 14).
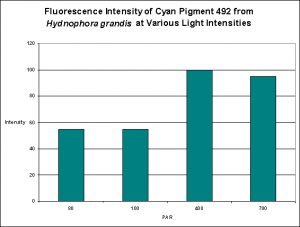
Figure 13. Effects of light intensity (generated by a metal halide lamp) on the generation of fluorescent Pigment 492.

Figure 14. Effects of different colored lighting on expression of fluorescence of Pigment 492. Light intensity was 200 µmol·m²·sec for each treatment.
Cyan Fluorescent Pigment 497
Pigment 497 is a bit different than other cyan pigments in its responses to both light intensity and spectral qualities. Production of this particular pigment in Acropora millepora was found to be most efficient at 100 µmol·m²·sec and only slightly less so at 400 µmol·m²·sec. Interestingly expression of this pigment was found to be practically non-existent at 80 µmol·m²·sec, and even less so at 700 µmol·m²·sec (see Figure 15).
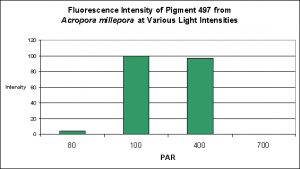
Figure 15. Effects of light intensity (generated by a metal halide lamp) on the generation of fluorescent Pigment 497.
Although red light promoted pigment production to a slight degree, green light and blue light were much better in making this coral colorful (see Figure 16).
Discussion
Before beginning our discussion of various cyan pigments and their reactions to light intensity and spectral quality, we should put the light intensities used by D’Angelo et al. in the perspective of reef aquarium hobbyists.
Recall that these researchers used these categories of light: ‘Very low’ (80 µmol·m²·sec), ‘low’ (100 µmol·m²·sec) ‘moderate’ (400 µmol·m²·sec), and ‘high’ (700 µmol·m²·sec) and used a photoperiod of 12 hours. Since a 12-hour photoperiod (or close to it) is fairly common among the hundreds of reef tanks I’ve had the pleasure of viewing, we will not concern ourselves with this any further. It is the light intensities that should be scrutinized. If light intensity plays an important part, this question begs to be asked:
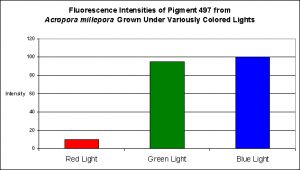
Figure 16. Effects of different colored lighting on expression of fluorescence of Pigment 497. Light intensity was 200 µmol·m²·sec for each treatment.
How common is a light intensity of, say, 400 µmol·m²·sec in reef tanks?
To try to answer this question, I present PAR measurements made in aquaria across the country. Various lighting systems were used (i.e., metal halides, fluorescents, PCs, etc. or combinations of any of these). Measurements were taken at or about the vicinity of corals that were thriving in captivity, and it give us a good idea of general light intensities found in home aquaria. See Figure 17.
As we can see from Figure 17, light intensities of 80 µmol·m²·sec and 100 µmol·m²·sec are easily achievable in home aquaria. However, the same cannot be said for the category of 400 µmol·m²·sec since only 13% (approximately) of the light measurements matched or exceeded that category. Very few aquariums (~1%) with thriving corals reached D’Angelo’s highest category (700 µmol·m²·sec). We should bear this in mind while evaluating following information.
In the same vein, we should review the spectral transmission qualities of the filters used in these experiments. Recall that we do not know the exact lamps used (although we know they were ‘blue’ metal halides). It would be much better to have the spectral signature of the light transmitted through the blue, green and red filters, but we do not.
Most of the cyan fluorescent pigments were expressed most strongly by the coral animals at 400 µmol·m²·sec (remember, the lamps used in these experiments produced ~50% of their output in the ‘blue’ region of the spectrum, suggesting they were high kelvin lamps. Unfortunately, email to the primary investigator requesting clarification has gone unanswered). These pigments include CFP483 (from Acropora pulchra), CFP484 (from Acropora millepora), CFP486 (from Montipora digitata), and CFP492 (from Hydnophora grandis).
The cyan fluorescent pigment 497 from Acropora millepora behaved differently – it was expressed most strongly at a light intensity of only 100 µmol·m²·sec, and was not expressed at all at 700 µmol·m²·sec.
In every case, red light promoted the least amount of colorful pigmentation although there were considerable differences in the level of expressions at the given light intensity of 200 µmol·m²·sec used in these experiments. For example, the levels of cyan pigment 486 found in the ‘red light’ Montipora digitata specimens were found to be at a level of 50% of that found in the specimens exposed to blue light. Similarly, the cyan pigment 492 levels in the Hydnophora grandis specimens were ~40% of those ‘blue light’ specimens. In all other cases, pigment expression in the animals exposed to red light was only 10-15% of that seen in the corals subjected to blue light.
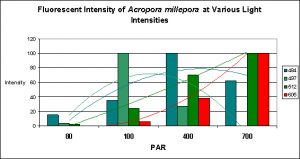
Figure 18. Trend lines show that Pigments 484 and 497 decrease in fluorescent intensity as PAR increases, while Pigments 512 and 605 increase in intensity. At 80 µmol·m²·sec, this coral would appear blue-green with apparent color becoming progressively greener until red and green pigments are dominant at 700 µmol·m²·sec. Is this a case of photoconversion, where light intensity alters the chemical makeup of pigments and causes them to shift from one fluorescent color to another? Or is this a case of pigment bleaching (P-484 & P-497) and light intensity-induced fluorescence (Pigments 512 and 605), or both?
Photoconversion, Pigment Bleaching and Color Mixing
It is common knowledge that corals often contain at more than one fluorescent pigment (a Favia specimen was found to have at least one dozen). The combined fluorescence of these pigments can and likely will influence our visual perception of the animal’s color in a phenomenon called ‘color mixing’. The addition (increase in expression or photoconversion), alternation (photoconversion) or subtraction (photobleaching or decrease in expression) of a pigment can have a profound affect on the animal’s appearance. Figure 18 demonstrates the possible but hypothetical increase or decrease in pigments according to light intensity alone. Imagine the possibilities we might observe when we add the effects of spectral quality on pigment expression. The exercise becomes mind boggling, and this is a relatively simple example!
There are only a few described cases of photoconversion in cyan pigments. Two are from Clade A (496 nm to an undetermined wavelength in Condylactis gigantea – Labas et al., 2002); 499 nm to 522 nm Anemonia sculata – Wiedenmann, 2002; and one case involves Acropora secale‘s conversion from cyan (484 nm) to green (515 nm) – Papina et al., 2002. See Figures 18-20 for additional information.
Conclusions
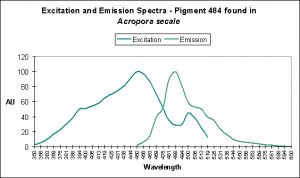
Figure 18. Light (mostly likely blue light) induces a shift in fluorescence from blue-green (484 nm) to green (with a peak emission at 515 nm). After Papina et al., 2002.
Light intensity and its spectral characteristics play important parts in promotion of coral coloration. Certainly, these are not the only requirements and become deciding factors only when other conditions such as water motion and water chemistry are correct.
The light intensity categories used by D’Angelo et al. are apparently relative to amounts of radiation received by natural reefs. Surprisingly, these categories were fairly close to those seen in aquaria when I divided the number of light measurements made in aquaria and divided the total number into 4 categories containing an equal number (see Figure 17). These were my classifications:
- Very Low: 15-100 µmol·m²·sec
- Low: 101-200 µmol·m²·sec
- Moderate: 201-400 µmol·m²·sec
- High: 401-700 µmol·m²·sec
Note that light intensities fitting into the moderate/high categories are often found directly beneath high intensity lamps and are comparatively small in area. Hence, some sort of light-measuring device, whether a lux meter or quantum meter, would be most helpful in determining proper placement of corals containing cyan fluorescent pigments. See here for comments on lux-to-PAR conversions: (www.advancedaquarist.com/2008/2/review) and a Product Review on a relatively inexpensive PAR meter: (www.advancedaquarist.com/2005/7/review).
It will take some time for me to review 11 years of lighting data I’ve collected, but I will try to refine these categories before this series is completed.
Most of the cyan pigments’ maximum fluorescent was noted in light fields of around 400 µmol·m²·sec (with the exception of Acropora millepora‘s pigment 497 that peaked at 100 µmol·m²·sec).
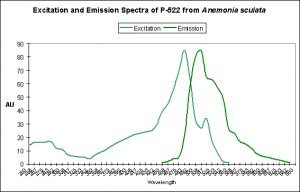
Figure 20. The double-peaked emission (and excitation, for that matter) suggests we are observing a pigment in transmission from one peak emission to another (499 nm to 522 nm, which is blue-green to green, respectively). From Wiedenmann, 2002.
When we examine the effects of spectral quality on pigmentation, we find in all cases that blue light promoted the expression of fluorescent pigmentation most effectively. Green light ran second best in promoting fluorescent pigment production, although the filter used by these researchers transmitted a fair amount of blue light. The red filter effectively, and almost exclusively, transmitted red wavelengths and was least effective in coaxing the coral animal to produce fluorescent pigments. Lamp spectral quality is another issue that should be investigated further.
In conclusion, the amount of light required to make corals ‘color up’ is not particularly difficult to achieve, although the low and high coloration thresholds can be fairly narrow (as in the case of Pigment 486 found in Montipora digitata). Perhaps more importantly, the production and/or maintenance of cyan pigments are not as sensitive to spectral quality (especially to red light) as some of the pigments we’ll examine in future articles. Simple visual observations of corals within our aquaria support the experimental evidence that blue-green pigments are not all that difficult for corals to produce with these artificial environments.
The negative effects of exposure to red light for prolonged periods (exceeding ~30 days or so) are becoming more apparent. In this article, we see that red light apparently fails to promote coral coloration as efficiently as either green or blue bandwidths. We discussed the effects of red light on coral growth in the December 2008 edition of Advanced Aquarist. See here for details: www.advancedaquarist.com/2008/12/aafeature1
The concept and practice of classifying pigments by clade is another important step. Previously, our best method of categorizing pigments was by fluorescence excitation and, more particularly, emission. While we can not say that pigments within a certain clade will react in roughly the same manner as another pigment within the same clade, we can begin to form generalizations. With time, the clade categories will probably prove to be an important tool in our understanding of fluorescent pigments and their production and maintenance.
I’m presently working on a project with the goal of finding an inexpensive method of classifying the particular fluorescent pigment(s) within corals. If successful, I’ll present this information in a future article.
With that, our examination of fluorescent pigments will end for this time. Next month, we’ll look at new information on green fluorescent pigments and how light intensity and quality promotes or discourages pigmentation. Future articles will focus on orange and red fluorescent proteins. This series will close with a look at the non-fluorescent chromoproteins found in so many corals. A complete reference list will appear in that article.
Questions? Comments? Please send them to me at [email protected].
0 Comments