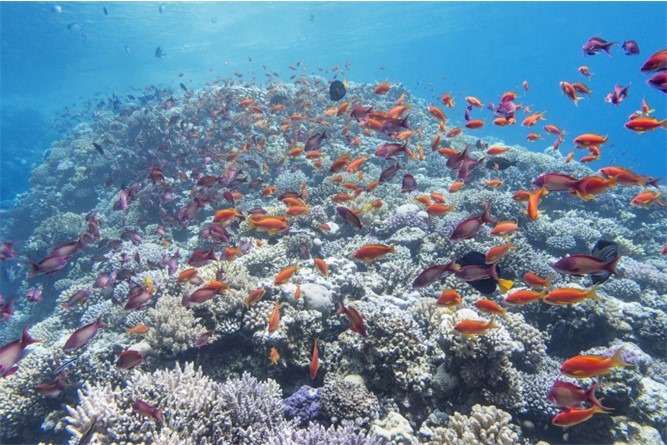
Fig 1. An extensive body of bleached but heterogeneous Red Sea zooxanthellate Scleractinia (hermatypes).
Investigations of the microscopic populations of reef-forming corals initially focussed on their “algal” partner and bacterial communities because of established experimental procedures, and it was hoped that elucidation of the mechanisms responsible for terminating symbiosis may help slow the decline in wild reef ecology (Fig 1.; Clerissi et al. 2018; Santoro et al. 2021). Much progress has been made yet the “trigger” for symbiont expulsion remains unclear (Boilard et al. 2020; Nielsen et al. 2018).
Putative mutualist prokaryotes of the genera Endozoicomonas and otherwise rare Ralstonia and Propionibacterium were found to be widespread members of coral holobionts, which may impart beneficial secondary metabolites, cycle nitrogen, or liberate protective antimicrobials (Clerissi et al. 2018). Nominal hydrogen sulphide-synthesising anaerobic green sulphur bacteria known as endoliths inhabit the deep skeletal matrices of corals (Takashima et al. 2000; Bryantseva et al. 2019; Yang et al. 2019).
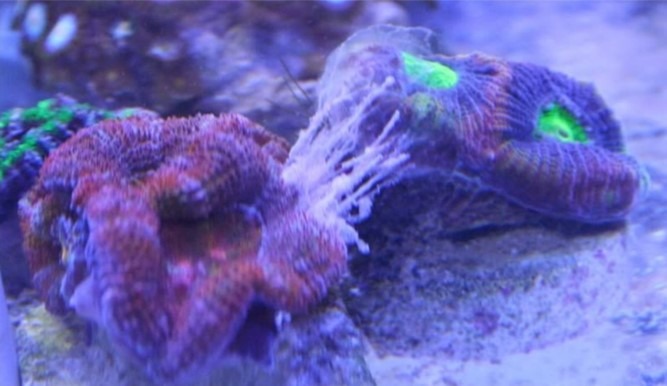
Fig 2. An iconic and compelling snapshot from the thread “When acans attack!” on the Aquarium Advice forum. The Acanthastrea species (Faviina: Lobophylliidae) fragment on the left has released mesenterial filaments to digest the Favia species (Faviina: Faviidae) “frag” on the right. Image courtesy of Jimbo7© 2010.
A loss of key microbial taxa in the mucosal microbiome exemplifies dysbiotic purging, which is frequently the harbinger of disease. Equally, probiotic manipulation restores multiplicity and transforms gene expression to reinstate eubiosis and defence, which supports the paradigm of hologenomics. Moreover microbial communities appear to support homeostasis in the face of anomalous temperatures (Clerissi et al. 2018; Santoro et al. 2021). The microeukaryotes of corals were considered less significant until these groundbreaking studies and the paradigm of the holobiont which acknowledges the vitality of each organism is contingent on its resident biota. Such is the philosophy behind holosystemics where contextualising the role of each member is unobtainable without the findings of leading-edge scientific investigations, while the preceding editorials convey indispensable background.
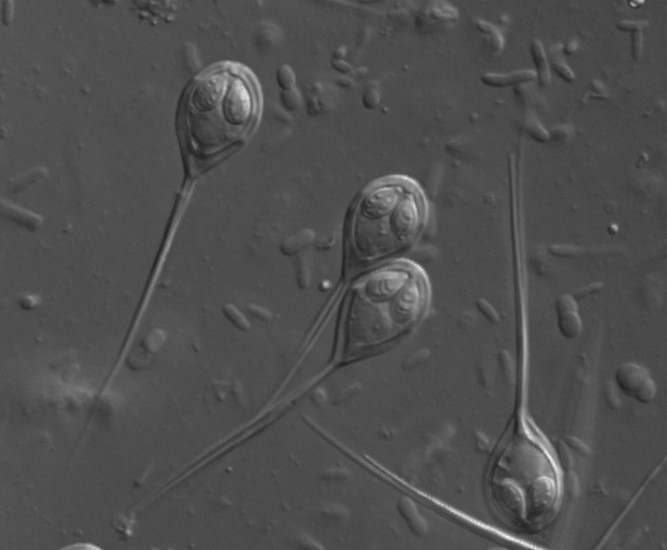
Fig 3. A micrograph of the chitinous myxospores of Henneguya species of myxozoan parasite. Image courtesy of https://www.FishPathogens.net/

Fig 4. A representation of an algal chromist of the phylum Chlorophyta.
The mucosal surfaces of corals extend several millimetres above their epidermis which ensnare some prey that may be digested by mesenterial filaments of the gastrodermis (Fig 2.; Kramarsky-Winter et al. 2006).
Dinoflagellates are members of the kingdom Chromista, the subkingdom Harosa, the infrakingdom Alveolata, the phylum Myzozoa, the subphylum Dinozoa, the infraphylum Dinoflagellata, and the class Dinophyceae (WoRMS 2021).
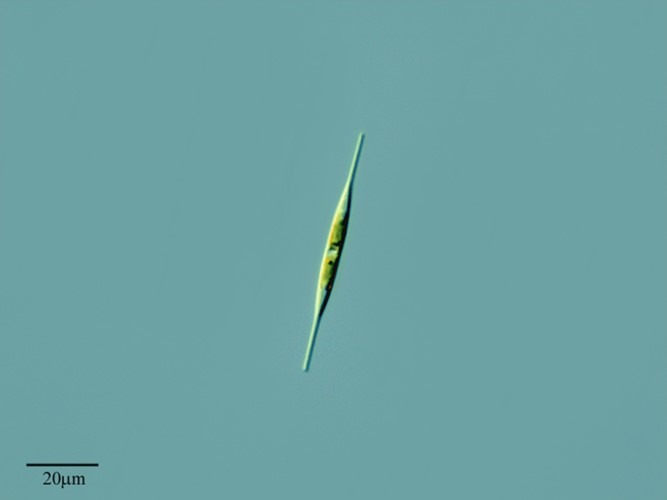
Fig 5. A micrograph of the motile neurotoxigenic heterokont chromist and diatom Nitzschia acicularis which may cause blooms in reef aquaria throughout “new tank syndrome” (Aslett 2024). Image courtesy of Antonio Guillén©, Proyecto Agua, https://www.Flikr.com/

Fig 6. A micrograph of a ciliophoran chromist featuring its prominent infraciliature used for locomotion that is synchronised and controlled by a microtubule network. A kinetosome is a cilium’s associated tubule which can be “bald” whereas a pair of kinetosomes is referred to as a kinetid, which can be a monokinetid, a dikinetid, or a compound polykinetid, whereas each row of kinetids is called a kinety. Recognise the pronounced oral groove and lengthy polykinetids that sweep particles into the phagocytic cytostome that engulfs nutrition in phagosomes via phagocytosis. Alternatively ciliates ingest particulate organic debris or entire host cells via their cytopharyngeal tube lined with rods called nematodesmata, which are often capped with beak-like armature (Fig 7.).
Zooxanthellae of the family Symbiodiniaceae utilise chlorophylls a and c2 (Riddle 2014) whereas Chlorophyta use chlorophylls a and b and therefore exploit unobstructed wavelengths. They are ubiquitous aquatic phototrophs of the family Ulvophyceae with polymorphisms that include free-living unicellular and multicellular macroalgae. Facultative anaerobic endolithic Ostreobium species have been isolated from Octocorallia and antipatharian black corals (McCauley et al. 2022) that interact with other holobiont affiliates and likely gain vitamin B12 from nearby bacteria, and trade C and N with the coral which includes biomineralisation of their skeleton. They protect the coral by lessening skeletal reflectance throughout detrimental irradiance and proliferate to assist postbleaching recuperation and survivorship (Bonacolta et al. 2023).
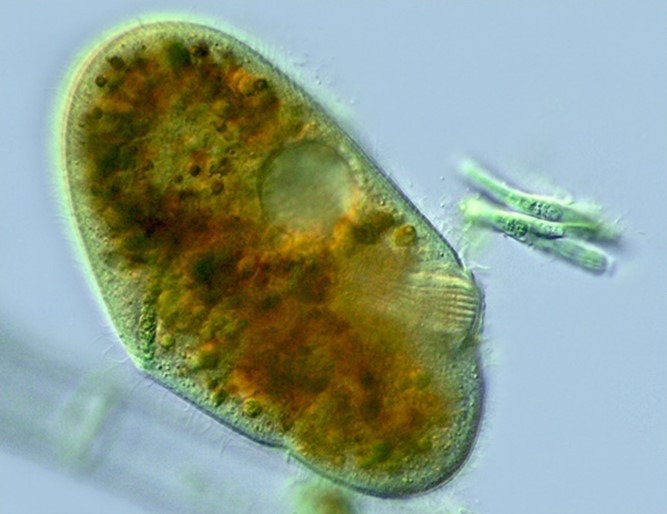
Fig 7. A micrograph of Nassula species of ciliate chromist of the family Nassophorea featuring its cytopharyngeal tube lined with nematodesmata. Image courtesy of Antonio Guillén©, Proyecto Agua, https://www.Flikr.com/
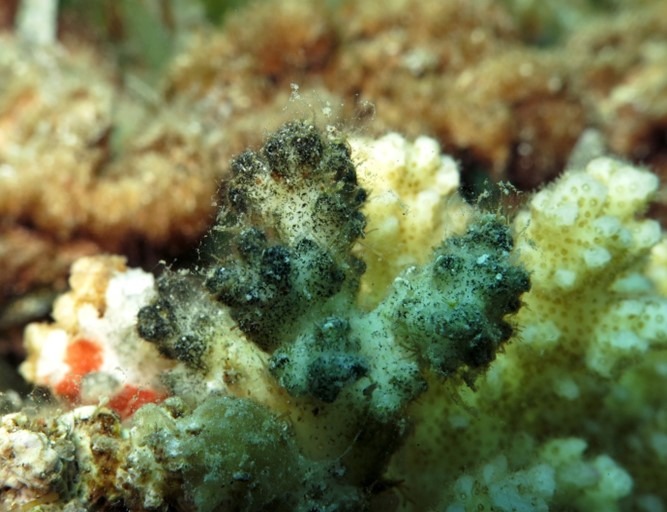
Fig 8. Skeletal eroding band (SEB) of Pocillopora species caused by the ciliophoran chromist Halofolliculina corallasia (Heterotrichea: Folliculinidae).
The phycobilins of aflagellate unicellular Rhodophyta confer their red colouration which is augmented by the fluorescence of chlorophyll a. Heterokonts are a diverse supergroup of mixotrophic photosynthetic eukaryotes representing a major branch of the kingdom Chromista hitherto considered Protozoa of the kingdom Protista (Fig 5.; Cavalier-Smith et al. 2006; Cavalier-Smith 2010; Cavalier-Smith 2018) whereas the plastids (chloroplasts) of dinoflagellates, rhodophytes, and heterokonts evolved from a red algal intracellular symbiont (Janouškovec et al. 2010; Bonacolta et al. 2023).
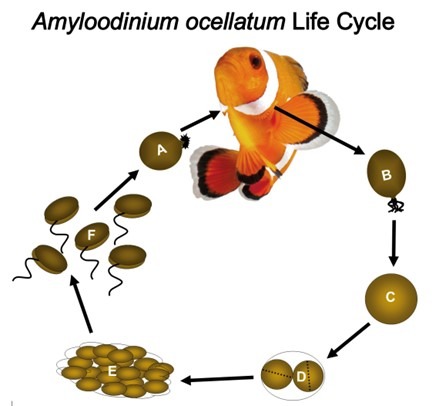
Fig 9. A representation of the life cycle of the parasitic alveolate dinoflagellate Amyloodinium ocellatum that causes “marine velvet”. [A] trophonts feed whilst attached to gills and skin. [B] mature trophonts detach and become encysted tomonts [C/D] and vegetatively reproduce [E]. Lysis releases swarms of encapsulated daughter dinospores [F] that locate and infect a host (Fig 11.; Fig 12.). Adapted from Reed & Francis-Floyd 1994, Schwarz & Smith 1998, and Smith 2019.
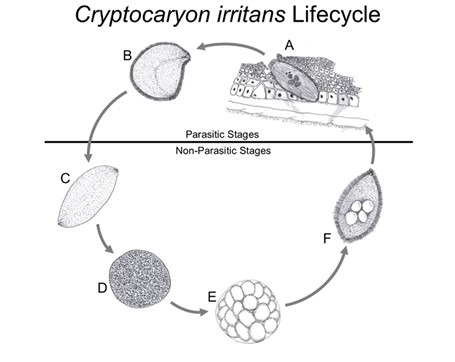
Fig 10. The life cycle of Cryptocaryon irritans: [A] phoront; [B] trophont; [C] protomont; [D] tomont; [E] tomites; [F] theront (Fig 13.). Drawings of the accurate representations of biological structures within Colorni and Diamant 1993, Colorni & Burgess 1997, and Chi et al. 2020.
Various kinds of rhodophyte prevail in deep and shallow saltwater ecosystems including macroalgae and crustose coralline algae, while the bacteria of their holobiont liberate cues that induce the settling metamorphosis of several species of coral planulae (Morse et al. 1988; Bonacolta et al. 2023) yet “algae” and algal turfs can perturb the recruitment of first polyps (Birrell et al. 2008; Bonacolta et al. 2023). However routine manual removal (“sea weeding”) increases wild coral cover by as much as 47 percent over three years (Smith et al. 2023). The filamentous conchocelis life stages of the rhodophytes Porphyra marcosii and Pyropia vietnamensis exclude other endoliths in zooxanthellate Scleractinia; and erode the frameworks of hydroids and may be endoparasites (Bonacolta et al. 2023).
Chromists of the infrakingdom Alveolata like apicomplexans (synonym: corallicolids) retain vestigial non-functional plastids that cause pernicious diseases in humans like malaria and toxoplasmosis (Howes et al. 2015; Kochanowsky & Koshy 2018; Vohsen et al. 2020). Their phylogeny is somewhat tenuous yet two species of photosynthetic chromerid have been isolated from reefs (Bonacolta et al. 2023) which flourish in sediments instead of forming enduring associations with corals (Vohsen et al. 2020; Bonacolta et al. 2023).

Fig 11. A scanning electron micrograph (SEM) of a dinospore of the brevetoxigenic “red tide” dinoflagellate Karenia brevis. Image courtesy of The Florida Fish and Wildlife Commission©.
The sequences of Type-N 18S ribosomal ribonucleic acid (rRNA) and 16S rRNA plastid apicomplexan-related lineages (ARLs) are common to corallicolids that dwell in symbiocytes with zooxanthellae (Putnam et al. 2017; Vohsen et al. 2020; Bonacolta et al. 2023). 23 genotypes of corallicolid plastid were found in 14 species of corals from three orders, where each type was limited to an order and depth, yet these zones and residents were sufficiently alike to suggest diversity and adaptability. However, four transcriptionally-active typically chlorophyll-associated gene homologues were isolated from the plastids of corallicolids at various depths from euphotic to aphotic implying a non-photosynthetic role (Vohsen et al. 2020). Nascent Corallicola aquarius-like species were nested in the dedicated order Corallicolida in 2021 where Gemmocystis cylindrus and Anthozoaphila gnarlus were recognised as coral symbionts (Kwong et al. 2021). This clade of corallicolids and chromerids thus represents the succession of non-photosynthetic obligate endoparasites from free-living phototrophs (Vohsen et al. 2020).
Fungi (mycoses) are recognised saprophytes of the coral holobiont that breakdown and absorb decaying particulate matter which have inspired the term “mycobiome”. They are unicellular or colonial with cell walls composed of b-glucan and chitin, which forms the exoskeletons of insects and the spores of myxozoan and microsporidian parasites (Fig 3.). b-glucan profoundly stimulates fish immunity (Bartelme 2004; Galindo-Villegas & Hosokawa 2004; Ganguly et al. 2010; Ringo et al. 2011; Alba et al. 2019) which is a major constituent of the human health supplement and useful coral feed, brewer’s yeast. The phyla Ascomycota and Chytridiomycota predominate in aquatic ecosystems where they perform a vital role in the parasitism and recycling of challenging-to-degrade (refractory) living substrates such as diatoms from which they generate particulate organic matter (POM), that is later biomineralized by microbes. Many reproduce both sexually and asexually and several have been associated with aetiologically-cryptic coral syndromes. Symbiotic mycoses may benefit the coral by converting organics to inorganics which are bioavailable to autotrophs, whereas several tunnelling skeletal fungi have been recognised. However, their incidence is likely underestimated inasmuch as their 18S rRNA sequences are challenging to extricate from their host’s (Bonacolta et al. 2023). Nevertheless a recent study used primers in the polymerase chain reaction (PCR) that annealed to scleractinian 18S rRNA and blocked its amplification (Clerissi et al. 2018). See figure 13. in part I of this series (Aslett 2023b).

Fig 12. A common clown (Amphiprion ocellaris) suffering from “marine velvet” (Amyloodinium cf. ocellatum; Fig 9.). Image courtesy of PhotoByMark©, https://www.Flickr.com/
Heterotrophic eukaryotes of the kingdom Chromista include non-photosynthetic pseudo fungi (Cavalier-Smith et al. 2006; Mohammadi et al. 2021) yet several ranked in the infrakingdom Alveolata and phylum Ciliophora cause devastating diseases of corals and fish (Fig 8.; Fig 9.; Fig 10.; Fig 12.; Fig 13.; Fig 14.; Fig 15.; Andrews 1914; Cheung et al. 1980; Colorni & Diamant 1993; Colorni & Burgess 1997; Diamant 1998; Antonius 1999; Antonius & Lipscomb 2000; Antonius 2000; Bruno et al. 2006; Woo 2006; McLean et al. 2008; Harikrishnan et al. 2010; Sweet & Bythell 2012; Sweet et al. 2012; Sweet et al. 2013; Bonacolta et al. 2023). Many ciliates are commensals or specialised ecto- and endo-symbionts of animals (Bonacolta et al. 2023) and their unique physiology has spawned terminology understood by a mere handful of parasitologists (Fig 6.; Fig 7.; Lom & Nigrelli 1970; Nelsen & Frankel 1979; Lynn 1981; Lynn 1982; Chapman et al. 2000; Woo 2006; Lynn 2008; Aslett 2024). They range from a few microns (mm) to several millimetres where their predation of autotrophic primary producers provides their predators with nutrients, albeit few ciliates are coral symbionts or commensals insofar as they typically cause disease (Bonacolta et al. 2023).
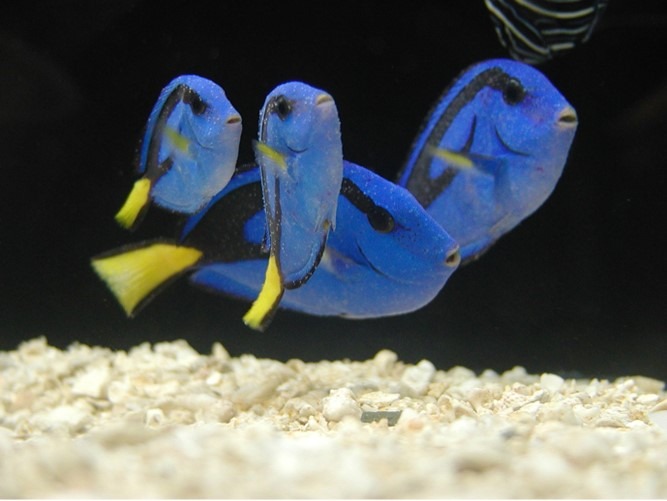
Fig 13. A quartet of regal tangs (Paracanthurus hepatus) peppered with “marine white spot” (Cryptocaryon irritans; Fig 10.). PhotoByMark©, https://www.Flickr.com/
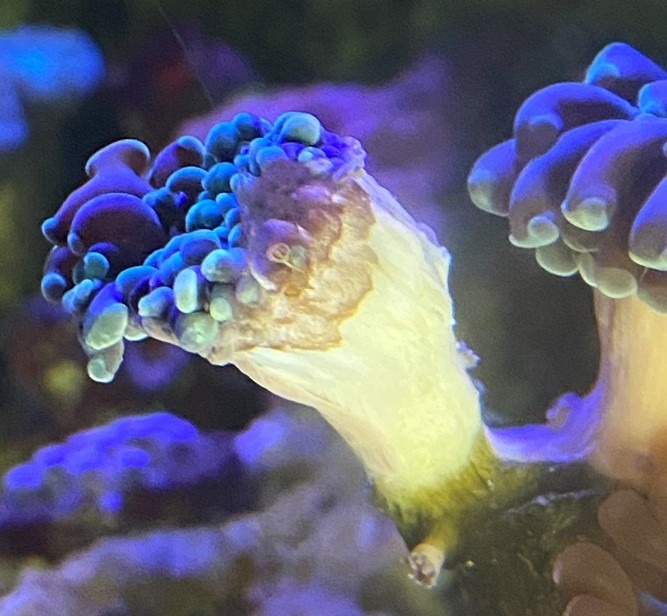
Fig 14. Brown jelly syndrome of Fimbriaphyllia ancora caused by the alveolate scuticociliate Philaster digitiformis strain FJ64835 (Sweet et al. 2013). Image courtesy of Tom Rudge©, Redditch, Worcestershire. https://instagram.com/toms_reef/
Unicellular stramenopile chromists of the genera Aplanochytrium, Thraustochytrium, and Labyrinthuloides and family Thraustochytriidae pervade the mucosal microbiome of Red Sea and Great Barrier Reef Faviidae (Siboni et al. 2010) of the remarkably aggressive suborder Faviina (Fig 2.; Aslett 2024). These geographically separated communities were remarkably alike that bloomed in Red Sea corals during autumn and winter (Siboni et al. 2010). Stramenopiles are heterotrophs that absorb nutrients saprophytically using an extracellular membranous ectoplasmic network secreted by the bothrosome (sagenogenetosome) peculiar to Thraustochytriidae (Bonacolta et al. 2023).
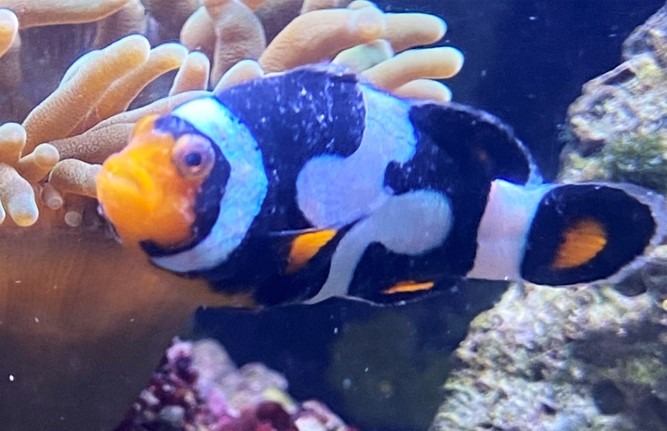
Fig 15. A moribund colour variant percula clownfish (Amphiprion percula) riddled with the alveolate ciliophoran chromist Brooklynella cf. hostilis. Image courtesy of Alex Cole©, Meridian, Idaho, USA.
High powered electron microscopy and nucleic acid sequence analyses putatively identified seven species of stramenopiles, that were embedded in the epidermis and mucosal layer of the Red Sea corals Fungia granulosa and Favia favus of the families Fungiidae and Faviidae. These organisms were 3 to 30 mm in diameter with spheroidal cytoplasmic inclusions of around 1 mm that formed white patchy aggregates concentrated around polyp mouths, that also occurred in the gastrodermis. Related pico- and nano-planktonic chromists are widespread in marine ecosystems where they assimilate environmental organic substrates which promotes these nutrients to more sizeable organisms (Kramarsky-Winter et al. 2006), whereas others are cephalopod, sponge, bivalve, and cnidarian pathogens but they appeared beneficial. Their preference for the mouths of polyps implies they may be parasites insofar as their degradative and assimilative competence for utilising organic waste, mucus, and/or prey, or they may be housekeeping commensals that degrade sloughing tissue (Kramarsky-Winter et al. 2006). Stramenopile profusion suggests they are unlikely to be sustained by nominal cellular turnover, however flat and boulder morphotypes are prone to significant sedimentation and its management would elevate these chromists to symbionts. Contrasting thraustochytrid stramenopiles manufacture polyunsaturated fatty acids including omega-3 and carotenoids which would benefit the holobiont (Siboni et al. 2010).
Stramenopiles remained in mucus and the gastrodermis when Fungia granulosa were irradiance-deprived for several months, where the corals became devoid of zooxanthellae but subsisted without zooplankton. These chromists may therefore be responsible for the remarkable postbleaching recuperation of nonbranching massive colonies and Fungiidae (Kramarsky-Winter et al. 2006). Nevertheless, they have been isolated from branching dysbiosis-susceptible Pocillopora damicornis (Clerissi et al. 2018).
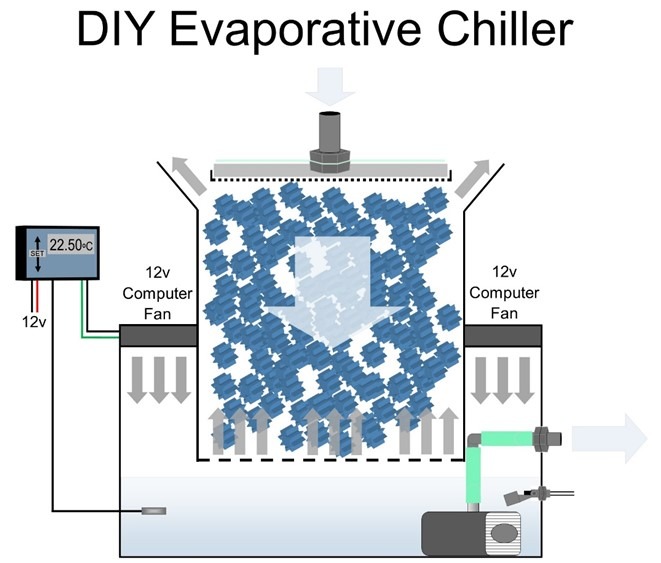
Fig 15. A blueprint of a low consumption DIY evaporative chiller that can be fabricated from Really Useful Boxes stacked on a small glass or acrylic aquarium (Aslett 2024). Deltec® manufactured evaporative chillers early in the millennium which are still available on selling platforms, but alas, trends and good practice seldom converge.
Over 30 to 50 percent of the sequences retrieved from the tissues of Djiboutian and New Caledonian P. damicornis were microeukaryotic, where over 90 percent were Symbiodiniaceae. The residuum appeared dominated by alveolate strains of non-pathogenic species of Licnophora ciliate and coccidians of the phylum Apicomplexa, while holobiont compositions were regionalised and may therefore climate-adapt (Fig 16.; Clerissi et al. 2018). Symbiodinium, Cladocopium, and Durusdinium-like species dominated the zooxanthellae, where Cladocopium clonatypes were endemic to New Caledonia, whereas Symbiodinium and Durusdinium prevailed in Djibouti. The study identified Navicula species of the phylum Bacillariophyta which are common to “soft” corals of the genus Dendronephthya, and fungi of the class Basidiomycota and family Agaricomycetes that populate Acropora hyacinthus. Coccidians and Licnophora species of ciliate have been isolated from coral disease, yet one study isolated the former in healthy Agaricia agaricita, A. tenuifolia, Favia fragum, Montastraea annularis, M. faveolata, Mycetophyllia ferox, Porites astreoides, and Siderastrea sidereal. No clinical signs were observed in P. damicornis and thus all genera were believed commensals or mutualists, however researchers could not rule out PCR-derived amplification of environmental sequences (Clerissi et al. 2018).
Dinoflagellates of the family Gymnodiniaceae and genus Gyrodinium are non-zooxanthellae members of the holobiont that manufacture UV-protective mycosporine-like amino acids (MAAs; Bonacolta et al. 2023).
Planktonic Microeukaryotes
The ecology of free-living microeukaryotes broadens our understanding of their role within nature and our systems. Parasitic Dinophyceae were first classified as alveolate groups I to V (MALV) and later group I to V Syndiniales. Group I includes the genera Ichthyodinium and Duboscquella whereas group II was designated Amoebophryidae which comprises at least 44 known clades. Syndiniales are typically non-genera-defined which exploit and cause lethality in other dinoflagellates, cercozoans, radiolarians, crustaceans, and fish where they develop their swarmer infective dinospores (Fig 9.; Fig 11.; Qui et al. 2021).

Fig 16. An illustration of a chromist of the phylum Apicomplexa.
Merely 1 to 4 percent of plankton belong to the phyla Acanthoecida, Bacillariophyta, Chrysophyceae, Ciliophora, and Porifera from the Zhubi Reef ecosystem of the South China Sea whereas the wealth of Dinophyceae ranged from 95 to near-100 percent. Gyrodinium rubrum, G. cf. gutrula, G. jinhaense, and Lepidodinium chlorophorum were the most abundant dinoflagellates. Waterborne eukaryotic diversity was highest in the comparatively host-abundant, enclosed, sheltered, and nutrient-rich lagoons, which included parasitic Amoebophrya species of heterotrophic dinoflagellate. Phytoplanktonic communities were dominated by Dinophyceae of the orders Gymnodiniales, Gonyaulacales, and Peridiniales in every environment whereas Syndiniales were common to lagoons and the pelagic zone. The study identified 78 species originating from 21 clades of Syndiniales and 23 families and 37 genera of Gonyaulacales, Gymnodiniales, Peridiniales, Prorocentrales, and Suessiales. Nevertheless the cloning and sequencing was somewhat insensitive and could not discern rarer taxa. 30 new species and eight new sequences of parasitic Syndiniales were discovered whose hosts include related Alexandrium minutum, Gyrodinium spirale, G. aureolum, G. uncatenum, G. lohmanni, G. instriatum, Karenia mikimotoi, Karlodinium micrum, K. veneficum, Lepidodinium viride, Margalefidinium polykrikoides, Prorocentrum triestinum, and Scrippsiella trochoidea. Amoebophrya species may parasitise distantly or unrelated chromists yet they may be opportunistic or host-specific that account for up to 24 percent of lagoonal plankton (Qui et al. 2021).
It is clear from these investigations that dinoflagellates dominate benthic, planktonic, and the microeukaryotic communities of corals in all environments from mesotrophic and eutrophic lagoons to the oligotrophic and frequently nutrient-limited pelagic zone. Free-living Dinophyceae favour warmer temperatures and enriched phosphate, and manufacture biochemicals that evolved to lower diversity and eliminate broad cross-sections of ecosystems.
Parasites drive evolution, bolster immunity, stabilise interactions with their hosts, and impact food webs through modification of host and dietary dynamics. Their life cycle causes nominal discomfort and malaise in the wild but it exerts acute pathology in recirculating systems. We must therefore prophylactically limit pathogens, parasites, and pests and obtain a livestock supplier that discusses the control of communicable diseases in their reef and fish-only systems. Especially the alveolate ciliophoran chromist Cryptocaryon irritans and the “eye parasite” Neobenedenia melleni of the phylum Platyhelminthes, the class Monogenea, and the order and family Capsalidae (Fig 13.).
Maintain 23oC (73.4oF), <1 and 0.065 mg l-1 NO3– and PO43- and leave surface waters uncovered. Cooler water carries more dissolved oxygen (DO) and exceedingly economical evaporative chillers optimise degas and regas, which may be DIY fabricated with nominal skills and “killed” weekly with fresh reverse osmosis (R/O) water to preclude their maturation (Fig 15.; Aslett 2023c; Aslett 2024). Bacterial virulence is amplified and corals are weakened above 27oC (80.6oF) whereas more DO is required during darkness which is directly proportional to flow (Aslett 2023c) so exploit the nocturnal cycles of flow devices prudently.
Next time we commence to explore the microbiota of the fish holobiont.
References
Alba, K., Kontogiorgos, V., Melton, L., Shahidi, F. & Varelis, P. (2019) Seaweed Polysaccharides (Agar, Alginate Carrageenan). Encyclopedia of Food Chemistry. Varelis, P., Melton, L. & Shahidi, F. (eds.). Academic Press, Oxford. pp 240-250.
Andrews, E., A. (1914) The bottle-animalcule, folliculina; cecological notes. The Biological Bulletin. 26(5), 262-285.
Antonius, A. (1999) Halofolliculina corallasia, a new coral-killing ciliate on Indo-Pacific reefs. Coral Reefs. 18(3), 300.
Antonius, A. (2000) Bioerrosion on Indo-Pacific reef corals: the skeleton eroding band (SEB) disease. Abstract, 3rd Int. Bioerosion Workshop, Barcelona. 13,.
Antonius, A., A. & Lipscomb, D. (2000) First protozoan coral-killer identified in the Indo-Pacific. Atoll Research Bulletin. 481,.
Aslett, C., G. (2024) The Complete Reef Aquarist, for hobbyists, the trade and academics – A Conservation Manual. Aslett, C., G. (ed.). Reef Ranch Publishing Ltd, Leeds, West Yorkshire, UK. In Press. https://www.reefranch.co.uk/
Aslett, C., G. (2023b) Holosystemics Part I: Captive Reef Function versus Malfunction. https://www.reefranch.co.uk/ .
Aslett, C., G. (2023c) Reef Digress: Saltwater REDOX. https://www.reefranch.co.uk/
Bartelme, T., D. (2004) Aquarium Fish: News from the Warfront with Cryptocaryon irritans: Part Four of Five. Advanced Aquarist.com. https://www.advancedaquarist.com/2004/3/mini
Birrell, C., Mccook, L., Willis, B. & Diaz-Pulido, G. (2008) Effects of benthic algae on the replenishment of corals and the implications for the resilience of coral reefs. Oceanography and marine biology. 46,.
Boilard, A., Dubé, C., E., Gruet, C., Mercière, A., Hernandez-Agreda, A. & Derome, N. (2020) Defining Coral Bleaching as a Microbial Dysbiosis within the Coral Holobiont. Microorganisms. 8, 1682. doi:10.3390/microorganisms8111682
Bonacolta, A., M., Weiler, B., A., Porta‐Fitó, T., Sweet, M., Keeling, P. & del Campo, J. (2023) Beyond the Symbiodiniaceae: diversity and role of microeukaryotic coral symbionts. Coral Reefs. 42, 567–577. https://doi.org/10.1007/s00338-023-02352-0
Bruno, D., Nowak, B., F. & Elliott, D. (2006) Guide to the identification of fish protozoan and metazoan parasites in stained tissue sections. Diseases of aquatic organisms. 70, 1-36.
Bryantseva, I., Tarasov, A., Kostrikina, N., Gaisin, V., Grouzdev, D. & Gorlenko, V. (2019) Prosthecochloris marina sp. nov., a new green sulfur bacterium from the coastal zone of the South China Sea. Archives of Microbiology. 201(10), 1399-1404.
Cavalier-Smith, T. (2010) Kingdoms Protozoa and Chromista and the eozoan root of the eukaryotic tree. Biol. Lett. 6, 342-345.
Cavalier-Smith, T. (2018) Kingdom Chromista and its eight phyla: a new synthesis emphasising periplastid protein targeting, cytoskeletal and periplastid evolution, and ancient divergences. Protoplasma. 255, 297-357.
Cavalier-Smith, T. & Chao, E., E., Y. (2006) Phylogeny and Megasystematics of Phagotrophic Heterokonts (Kingdom Chromista). J Mol Evol. 62, 388-420.
Chapman, M., Dolan, M. & Margulis, L. (2000) Centrioles and kinetosomes: form, function, and evolution. The Quarterly review of biology. 75(4), 409-429.
Cheung, P., Nigrelli, R. & Ruggieri, G. (1980) studies on the morphology of uronema marinum Dujardin (Ciliatea: Uronematidae) with a description of the histopathology of the infection in marine fishes. Journal of Fish Diseases. 3(4),.
Chi, H., Goldstein, M., Pichardo, A., Wei, Z., Chang, W. & Gong, H. (2020) Infectivity and genes differentially expressed between young and aging theront cells of the marine fish parasite Cryptocaryon irritans. PLoS ONE. 15(8),.
Clerissi, C., Brunet, S., Vidal-Dupiol, J., Adjeroud, M., Lepage, P., Guillou, L., Escoubas, J-M. & Toulza, E. (2018) Chromists within corals: the hidden diversity. Front Microbiol. 9, 2043.
Colorni, A. & Burgess, P. (1997) Cryptocaryon irritans Brown 1951, the cause of “white spot disease” in marine fish: an update. Aquarium Sciences and Conservation. 1, 217-238.
Colorni, A. & Diamant, A. (1993) Ultrastructural features of Cryptocaryon irritans, a ciliate parasite of marine fish. European Journal of Chromistology. 29(12), 425-434.
Diamant, A. (1998) Brooklynella hostilis (Hartmannulidae), a pathogenic ciliate from the gills of maricultured sea bream. Bulletin of the European Association of Fish Pathologists (United Kingdom). 18(1), 33-36.
Galindo-Villegas, J. & Hosokawa, H. (2004) Immunostimulants: Towards Temporary Prevention of Diseases in Marine Fish. https://www.researchgate.net/profile/Jorge_Galindo-Villegas/publication/260087945_Immunostimulants_Towards_Temporary_Prevention_of_Diseases_in_Marine_Fish/links/5627702e08ae2b313c54d801/Immunostimulants-Towards-Temporary-Prevention-of-Diseases-in-Marine-Fish.pdf
Ganguly, S., Paul, I. & Mukhopadhayay, S. (2010) Application and Effectiveness of Immunostimulants, Probiotics, and Prebiotics in Aquaculture: A Review. Israeli Journal of Aquaculture – Bamidgeh. 62, 130-138.
Harikrishnan, R., Balasundaram, C. & Heo, M. (2010) Scuticociliatosis and its recent prophylactic measures in aquaculture with special reference to South Korea Taxonomy, diversity and diagnosis of scuticociliatosis: Part I Control strategies of scuticociliatosis: Part II. Fish & shellfish immunology. 29(1), 15-31.
Howes, R., Reiner, R., Jr., Battle, K., Longbottom, J., Mappin, B., Ordanovich, D., Tatem, A., Drakeley, C., Gething, P., Zimmerman, P., Smith, D. & Hay, S. (2015) Plasmodium vivax Transmission in Africa. PLoS Neglected Tropical Diseases. 9(11),.
Janouškovec, J., Horak, A., Obornik, M., Lukes, J. & Keeling, P., J. (2010) A common red algal origin of the apicomplexan, dinoflagellate, and heterokont plastids. Proc Natl Acad Sci. 107, 10949–10954.
Kochanowsky, J. & Koshy, A. (2018) Toxoplasma gondii. Current Biology. 28(14), 761-802.
Kramarsky-Winter, E., Harel, M., Siboni, N., Ben Dov, E., Brickner, I., Loya, Y. & Kushmaro, A. (2006) Identification of a chromist-coral association and its possible ecological role. Mar Ecol Prog Ser. 317, 67–73.
Kwong, W., K., Irwin, N., A., T., Mathur, V., Na, I., Okamoto, N., Vermeij, M., J., A. & Keeling, P., J. (2021) Taxonomy of the Apicomplexan Symbionts of Coral, including Corallicolida ord. nov., Reassignment of the Genus Gemmocystis, and Description of New Species Corallicola aquarius gen. nov. sp. nov. and Anthozoaphila gnarlus gen. nov. sp. nov. J Eukaryot Microbiol. 68, e12852.
Lom, J. & Nigrelli, R. (1970) Brooklynella hostilis n. g., n. sp., a Pathogenic Cyrtophorine Ciliate in Marine Fishes. The Journal of Eukaryotic Microbiology. 17(2), 224-232.
Lynn, D. & Small, E. (1982) Chromist kinetids: structural conservatism, kinetid structure, and ancestral states. Bio Systems. 14(3-4), 377-385.
Lynn, D., H. (1981) The organization and evolution of microtubular organelles in ciliated protozoa. Biological Reviews. 56, 243-292.
Lynn, D., H. (2008) Ciliated Protozoa, Characterisation, Classification and Guide to the Literature. Third Edition. Springer Science and Business Media B. V., New York, USA. p 204.
McLean, E., Salze, G. & Craig, S., R. (2008) Parasites, diseases and deformities of cobia. Ribarstvo. 66(1), 1-16.
Mohammadi, M., A., Cheng, Y., Aslam, M., Jakada, B., H., Wai, M., H., Ye, K., He, X., Luo, T., Ye, L., Dong, C., Hu, B., Priyadarshani, S., Wang-Pruski, G. & Qin, Y. (2021) ROS and Oxidative Response Systems in Plants Under Biotic and Abiotic Stresses: Revisiting the Crucial Role of Phosphite Triggered Plants Defense Response. Frontiers in microbiology. 12, 631318.
Morse, D., E., Hooker, N., Morse, A., N., C. & Jensen, R., A. (1988) Control of larval metamorphosis and recruitment in sympatric agariciid corals. J. Exp. Mar. Biol. Ecol. 116,193-217.
Nelsen, E., M. & Frankel, J. (1979) Regulation of corticotype through kinety insertion in Tetrahymena. J. Exp. Zool. 210, 277-287.
Nielsen, D., A., Petrou, K. & Gates, R., D. (2018) Coral bleaching from a single cell perspective The ISME Journal. 12, 1558–1567. https://doi.org/10.1038/s41396-018-0080-6
Putnam, H., Barott, K., Ainsworth, T. & Gates, R. (2017) The Vulnerability and Resilience of Reef-Building Corals. Current Biology. 27(11), 1551-1706.
Qiu, D., Huang, L., Zhuang, Y., Zhong, Y., Tan, Y., Li, X., Liu, S., Huang, H. & Lin, S. (2021) Dinoflagellate-targeted PCR reveals highly abundant and diverse communities of parasitic dinoflagellates in and near Zhubi Reef, South China Sea. Coral Reefs. 40, 1931–1939.
Reed, P. & Francis-Floyd, R. (1994) Amyloodinium infections of marine fish. College of Veterinary Medicine, Florida Cooperative Extension Service. Institute of Food and Agricultural Sciences, University of Florida. Fact Sheet VM-90. pp 1-3.
Riddle, D. (2014) Effects of Yellow Water on Light Transmission in an Aquarium – Why Should You Care. AdvancedAquarist.com. https://www.advancedaquarist.com/2014/1/aafeature
Ringo, E., Olsen, R., Gonzalez V., J. & Wadsworth, S. (2011) Use of Immunostimulants and Nucleotides in Aquaculture: A Review. Journal of Marine Science: Research & Development. 2,.
Santoro, E., P., Borges, R., M., Espinoza, J., L., Freire, M., Messias, C., S., M., A., Villela, H., D., M., Pereira, L., M., Vilela, C., L., S., Rosado, J., G., Cardoso, P., M., Rosado, P., M., Assis, J., M., Duarte, G., A., S., Perna, G., Rosado, A., S., Macrae, A., Dupont, C., L., Nelson, K., E., Sweet, M., J., Voolstra, C., R. & Peixoto, R., S. (2021) Coral microbiome manipulation elicits metabolic and genetic restructuring to mitigate heat stress and evade mortality. Science advances. 7(33), 3088.
Schwarz, M., H. & Smith, S., A. (1998) Getting Acquainted with Amyloodinium ocellatum. Virginia Cooperative Extension. Sea Grant Virginia. https://pdfs.semanticscholar.org/e5c8/5c992feee6855b31b20707f947a0d9b895bf.pdf
Siboni, N., Rasoulouniriana, D., Ben-Dov, E., Kramarsky-Winter, E., Sivan, A., Loya, Y., Hoegh-Guldberg, O. & Kushmaro, A. (2010) Stramenopile microorganisms associated with the massive coral Favia sp. J Eukaryot Microbio.l 57, 236–244.
Smith, S., A. (2019) Getting Acquainted with Amyloodinium ocellatum. FACT SHEET Publication 600-200 Virginia Tech Virginia Sea Grant College Program, Virginia Cooperative Extension. https://vtechworks.lib.vt.edu/bitstream/handle/10919/88929/600-200(CNRE-39P).pdf
Smith, H., A., Fulton, S., E., McLeod. I., M. & Bourne, D., G. (2023) Sea-weeding: Manual removal of macroalgae facilitates rapid coral recovery. Journal of Applied Ecology. 00, 1-13. DOI: 10.1111/1365-2664.14502
Sweet, M. & Bythell, J. (2012) Ciliate and bacterial communities associated with White Syndrome and Brown Band Disease in reef-building corals. Environmental microbiology. 14(8), 2184-2199.
Sweet, M., Craggs, J., Robson, J. & Bythell, J. (2013) Assessment of the microbial communities associated with white syndrome and brown jelly syndrome in aquarium corals. Journal of Zoo and Aquarium Research. 1(1), 20-27.
Sweet, M., Jones, R. & Bythell, J. (2012) Coral diseases in aquaria and in nature. Journal of the Marine Biological Association of the United Kingdom. 92(4), 791-801.
Takashima, T., Nishiki, T. & Konishi, Y. (2000) Anaerobic oxidation of dissolved hydrogen sulfide in continuous culture of the phototrophic bacterium Prosthecochloris aestuarii. Journal of bioscience and bioengineering. 89(3), 247-251.
Vohsen, S., A., Anderson, K., E., Gade, A., M., Gruber-Vodicka, H., R., Dannen- berg, R., P., Osman, E., O., Dubilier, N., Fisher, C., R. & Baums, I., B. (2020) Deep-sea corals provide new insight into the ecology, evolution, and the role of plastids in widespread apicomplexan symbionts of anthozoans. Microbiome. 8, 1–15.
Woo, P., T., K. (2006) Fish Diseases and Disorders. Volume 1. Protozoan and Metazoan infections. Seconds Edition. Woo, P., T., K. (ed.). CABI, CAB International, Cambridge, Massachusetts, USA. pp 168-169.
WoRMS (2021) World Register of Marine Species. Dinophyceae. https://www.marinespecies.org/aphia.php?p=taxdetails&id=19542
Yang, S., Tandon, K., Lu, C., Wada, N., Shih, C., Hsiao, S., Jane, W., Lee, T., Yang, C., Liu, C., Denis, V., Wu, Y., Wang, L., Huang, L., Lee, D., Wu, Y., Yamashiro, H. & Tang, S. (2019) Metagenomic, phylogenetic, and functional characterization of predominant endolithic green sulfur bacteria in the coral Isopora palifera. Microbiome, 7(3),.
Learn more about the author and his amazing work HERE!
0 Comments