Circadian rhythm is defined by one source as “any recurrent sequence of physiological or behavioral activities repeated on a daily basis” (Wallace et al. 1996) which become merely quasi-equivalent to 24 hours in fixed conditions, circa day (dian; Dominoni 2015). It is customary to italicize genes while herein we present foundational studies that paved the way for our current understanding to assist aquarists’ comprehension of this fascinating discipline. Consult: Appendix I for unfamiliar terms.
Complex intra- and extra-cellular biochemical, metabolic, and endocrinal processes have evolved to ensure the majority of living organisms have a daily 24-hour “clock” which demands hour-by-hour differential expression of a repertoire of genes, where seasonal and 28-day cycles drive an organism’s procreation competency (fecundity; Fig 3.). Circadian rhythms appear to be intrinsic and indispensable components of homeostasis which have evolved autonomously numerous times in most forms of life (Rosbash 2009). Periodic entrainment relies upon environmental cues (zeitgebers) like light and nutrition, where hermatypic corals appear stimulated nocturnally by moonlight and a profusion of zooplankton (Abe 1939; Jokiel et al. 1985; Hoadley et al. 2011; Brady et al. 2016). Light impacts amino acid uptake like glycine, alanine, and phenylalanine that elicit scleractinian feeding responses without which the tentacles of most daytime large polyps stony (LPS) corals do not extend (Houlbrèque & Ferrier‐Pagès 2009; Hoadley et al. 2011; Ying et al. 2018). Polyp expansion and contraction appears direct and light-responsive because tentacle activity exhibited a robust rhythmicity when colonies of LPS Favia fragum were exposed to diurnal light:dark cycles, insofar as polyps were routinely fully open at night, albeit precise periodicity was lost in continuous darkness (Fig 2.; Hoadley et al. 2011).
The basic-helix-loop-helix (bHLH)-Per-Arnt-Sim (PAS) transcription factors encoded on clock and cycle (mammalian: bmal1/mop3) are integral to a positive feedback loop where the CLOCK:CYCLE heterodimer regulates E-box motif (CACGTG) downstream transcription, while a negative feedback loop exploits PERIOD:CRYPTOCHROME dimers such as PERIOD:CRY2 in mammals or PERIOD:TIMELESS in fruit flies which repress CLOCK:CYCLE (Dunlap 1999). TIMELESS:CRY-modulated negative feedback loops in insects are slightly diverse and disparities occur in mammals, hence numerous “clock” intracellular mechanisms and cascades use an assortment of circadian proteins (Harmer et al. 2001; Yuan et al. 2007).
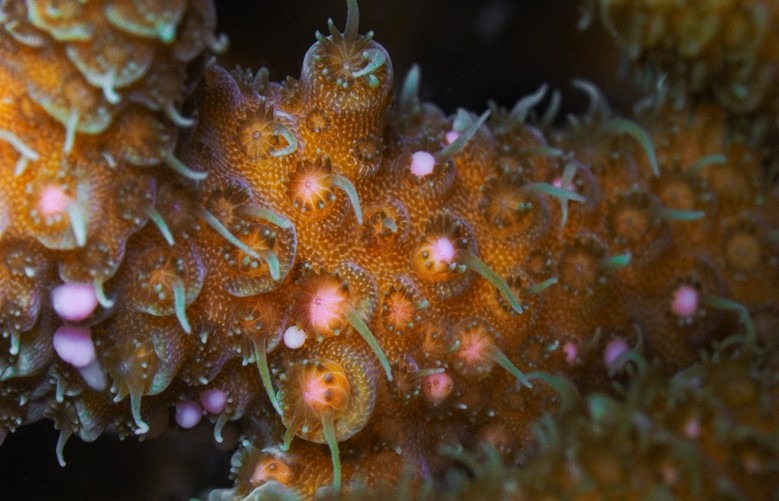
Fig 1. Imminent egg and sperm bundle broadcasting in Acropora millepora.
Entrained endogenous timekeeping retains an inertia that operates irrespective of cues for finite periods which is conspicuous in humans with jetlag (Konopka & Benzer 1971; Brady et al. 2016). Likewise phenotypic, metabolic, and transcriptomic coral rhythms continue when irradiance-deprived, which are distinct from photon-induced direct diurnal responses
(Dibner et al. 2010; Brady et al. 2011; Hoadley et al. 2011). The molecular means by which coral impulses occurred remained enigmatic despite their synchronized spawns (Brady et al. 2011; Sorek et al. 2014) where punctuality expedites the fusion of intraspecific gametes while it hinders interspecific hybridization (Levitan et al. 2004; Baird et al. 2009). These remarkable events are preceded by months of physiological change (Harrison et al. 1984) where dinoflagellate chronobiology is better understood which may orchestrate their host’s (Sorek et al. 2013).
Corals have neurone webs but lack central nervous systems (CNSs) and eyes hence the timing of their annual spawns is extraordinary (Brady et al. 2011) albeit plants exhibit analogous behaviors, however, conspecific wild coral cohorts broadcast their gametes reliably each year within the same 15-minute window (Vize et al. 2005; Levitan et al. 2011). Seasonal variances in temperature and weather, moonlight, and solar irradiance set the month, date, and hour respectively (Babcock et al. 1986; Wallace et al. 1986; Penland et al. 2004; van Woesik et al. 2006; Brady et al. 2009) where spawning typically occurs on still nights in tranquil seas (Oldach et al. 2017). Nevertheless, the molecular intricacies that underpin this timekeeping remain largely unexplained (Levitan et al. 2011) where spawns appear to be stimulated by daily solar irradiance making them non-circadian (Brady et al. 2009). Such activities have acquired asynchrony in the Red Sea which poses a further threat to wild reef ecology (Shlesinger & Loya 2019).
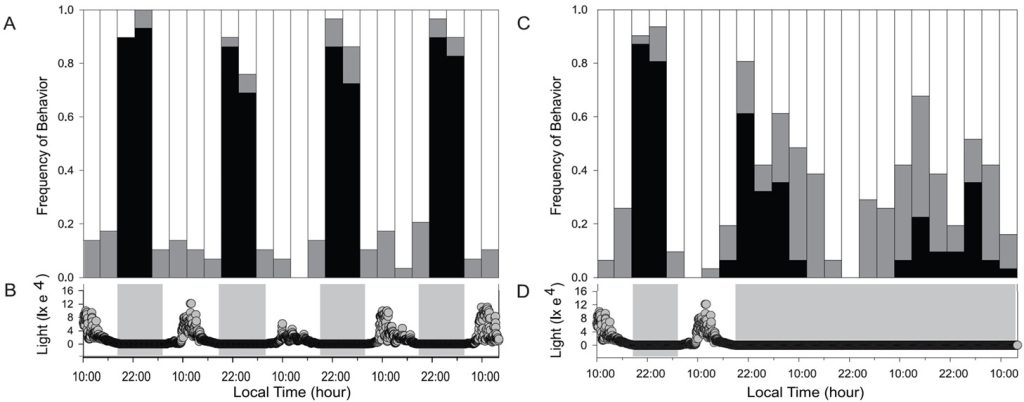
Fig 2. Polyp extension assays of large polyp stony (LPS) Favia fragum where black indicates fully open, partial retraction (grey), and fully closed (white). [A] throughout a tropical cycle of day and night where shaded regions indicate night [B]. [C] during continuous darkness when extension becomes arhythmic which suggests that polyps respond directly to light and are not under the control of an endogenous “clock” (circadian rhythm). Graphs and analyses courtesy of Hoadley et al. 2011 and the Creative Commons Attribution License.
cry2 has been associated with short-term circadian and long-term reproductive rhythms in insects and mammals despite awareness that coral gametogenesis and release are not ostensibly modulated by an endogenous pacemaker (Boden & Kennaway 2006; Dolatshad et al. 2006; Sandrelli et al. 2008; Dolatshad et al. 2009, cited in Hoadley et al. 2011; Hardin 2009; Brady et al. 2011). cryptochrome orthologues are likely key players in diel and monthly chronobiology (Hoadley et al. 2011).
10 adult colonies of Acropora millepora were collected from Orpheus and Pelorus Islands, The Great Barrier Reef (GBR) in November 2008 and were cultured ex situ under wildtype illumination at the Orpheus Island Research Station where they spawned in harmony with those in the wild. Gamete bundles were mixed and left for up to two hours to facilitate zygotic fusion. Planktonic planulae were washed and reared in vessels supplied with 1.0 µm filtered seawater at 28oC and 12 hours of 150 lux (~4 μmol m-2 s-1 PAR) from a Hagen Sun-Glo 40W fluorescent tube per day (Brady et al. 2011).
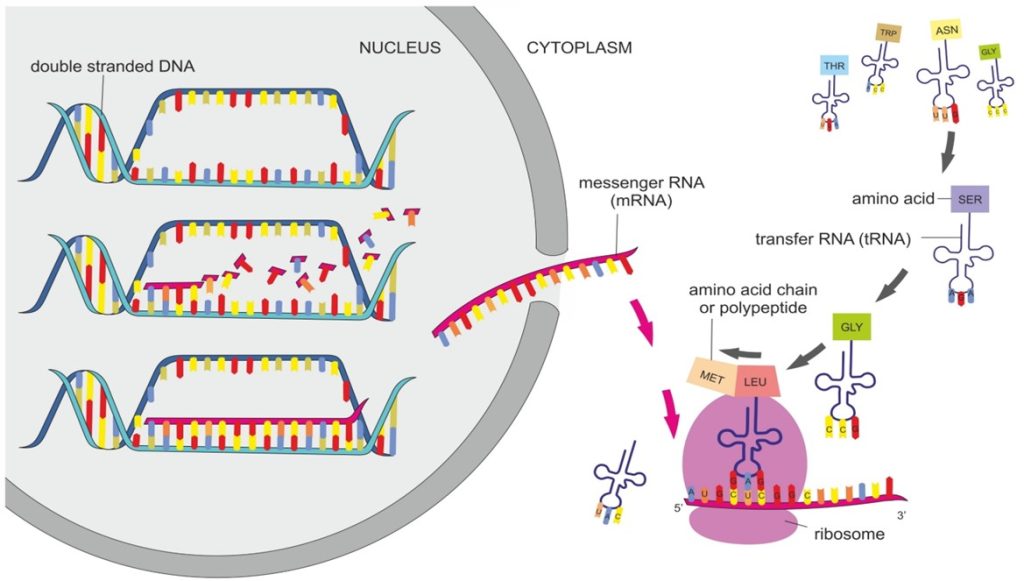
Fig 3. Polypeptide chain manufacture: transcription (expression) of nucleus-bound deoxyribonucleic acid (DNA) creates messenger ribonucleic acid (mRNA) which is destined to be translated by a ribosome (pink) after translocation to the cytoplasm. Transfer ribonucleic acid (tRNA)-bound amino acids are concatenated according to the mRNA sequence, while their R-group (sidechain) charges and shapes determine protein folds.
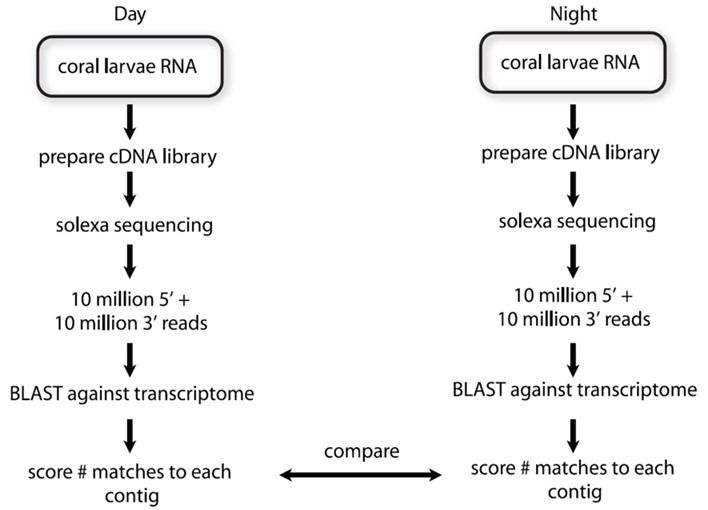
Fig 4. A comparative experiment devised by Brady and colleagues to ascertain the differential diurnal expression of candidate circadian and light-responsive genes over 24 hours in azooxanthellate larvae of A. milliepora (Brady et al. 2011). Flowchart courtesy of Brady et al. 2011 and the Creative Commons Attribution License.
A. millepora planulae transmute after six days from elongate plankton to a pre-settling morphotype with nascent tentacle buds, when one group was subjected to 24 hours of darkness (DD) while the other continued receiving 12 hours of each (LD). Larvae were sampled from both groups at four-hour intervals commencing two hours after illumination in LD, and each batch of 200 to 500 planulae were frozen in a proprietary RNA stabilizing liquid, and the same procedures were carried out in November 2009 (Brady et al. 2011).
Mature colonies collected from Orpheus and Pelorus Islands in November 2009 were exposed to 13 hours of 21,000 lux (~567 μmol m-2 s-1) per day from a Sylvania Coral-Arc 20,000K metal halide (MH) lamp for 35 days (13:11 LD). A two-to-three-centimeter branch was removed from a colony every four hours throughout a 24-hour period, immediately immersed in 1 ml of trizol reagent, ground into a paste with a pestle and mortar, and cryogenically frozen at -80oC, where another round of 24-hour sampling was carried out 20 days later. mRNA was later extracted, sequenced, and analyzed (Brady et al. 2011).
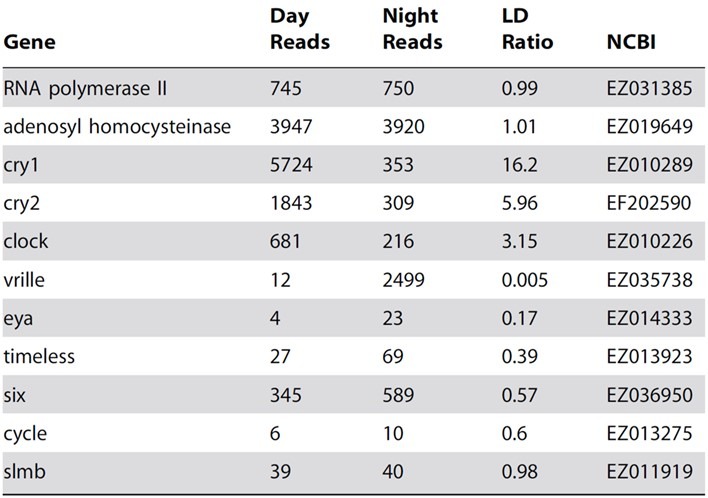
Table 1. The comparative day and night expression of candidate circadian orthologues within A. millepora larvae with elucidated roles in other organisms, with The National Centre for Biotechnology Information’s (NCBI’s) gene accession identifiers. Light impacted expression by more than 1.5-fold in all but slmb (supernumerary limbs) where cryptochrome (cry) 1 and 2 had the highest LD ratios due to their meaningful daytime transcription. The expression of phosphorylation-modulating F-box protein encoded by slmb, appears non-photosensitive. A differential threshold of 1.5-fold is adopted in numerous genomic approaches insofar as it reflects a significant transcriptional change (Urban et al. 2006, cited in Reitzel et al. 2010). However, clock and non-cnidarian per1 (period 1; Reitzel et al. 2010) and timeless and vrille were upregulated in light and dark respectively, where the latter encodes for a leucine-zipper class transcription factor carried by fruit flies of the genus Drosophila (Blau & Young 1999) which was differentially expressed 200-fold (LD ratio 0.005; Brady et al. 2011). eyes absent (eya) and the six homeobox (six) encode for seasonal daylength-modulated transcription factors in other organisms (Dardente et al. 2010; Masumoto et al. 2010) that appeared light-responsive in A. millepora. RNA polymerase II and adenosyl homocysteinase were used as primary and secondary controls due to their 24-hour elevated abundances with LD ratios approaching 1 (Brady et al. 2011). Table courtesy of Brady et al. 2011 and the Creative Commons Attribution License.
Two samples from the light:dark (LD) larval groups taken 12 hours apart at 10 hours after commencement of light and dark phases were sent for next generation Solexa whole transcriptome sequencing where ten million reads were performed from both 5- and 3-prime (3’) ends available at ftp://ftp.xenbase.org/pub/Coral/. A library of complementary strands of deoxyribonucleic acid (cDNA) was generated from messenger ribonucleic acid (mRNA) using the enzyme AMV reverse transcriptase, and quantitative polymerase chain reaction (QPCR) determined 24-hour differential expression and whether the candidate circadian genes identified by Vize in 2009 were integral to an entrained “clock” or were essentially light-responsive (Brady et al. 2011).
BLAST analysis searched for day and night transcribed orthologues within the expressed sequence tag (EST) data generated by Meyer and collaborators for A. millepora 454 in 2009, whereafter sequences comprising overlapping oligomers (contigs) were screened for similar biological functions including those recognized in the azooxanthellate anemone, Nematostella vectensis (Fig 4.; Brady et al. 2011).
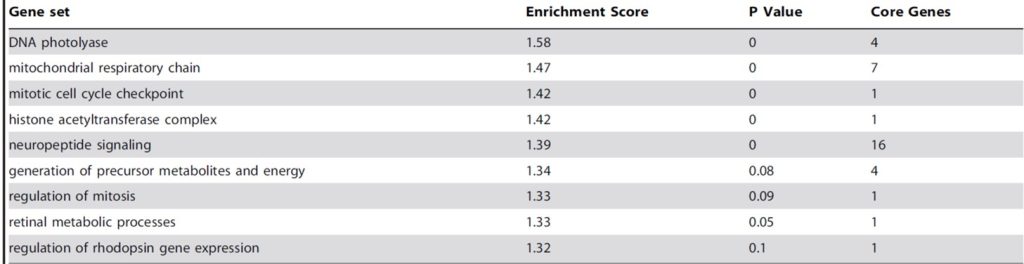
Table 2. Daytime gene ontology (GO) clustering pathways determined using gene set enrichment analysis (GSEA) of Acropora orthologues matching 26 sets with designated roles in the genome of Nematostella species. Table courtesy of Brady et al. 2011 and the Creative Commons Attribution License.
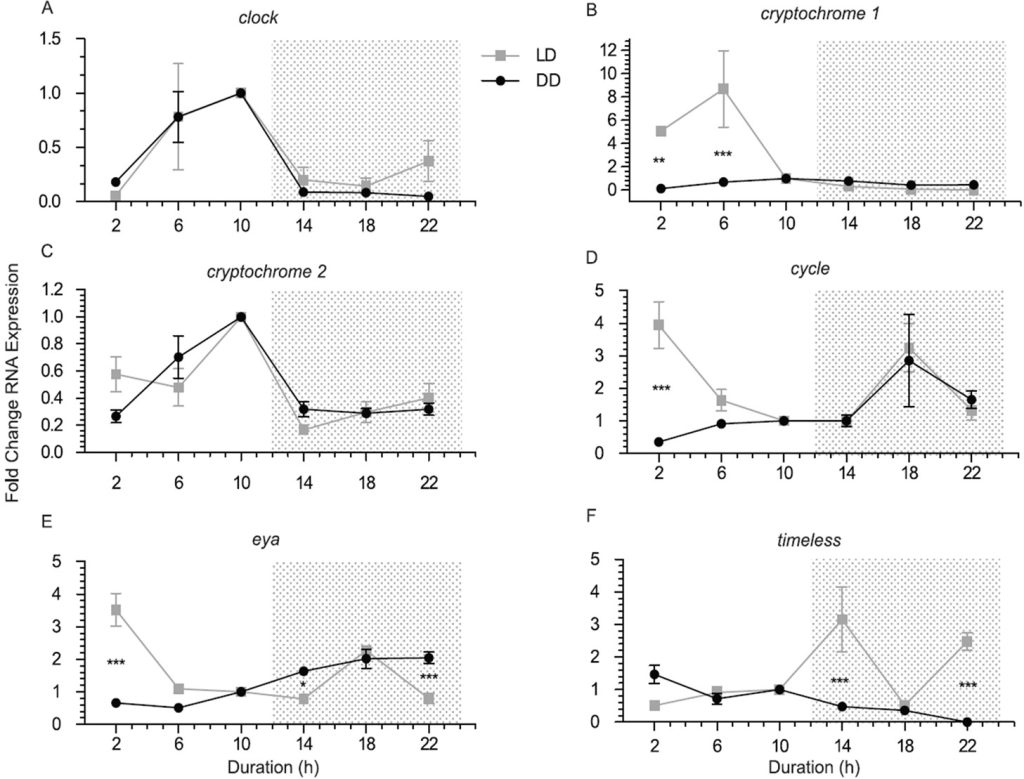
Fig 5. Duration in hours versus alteration in mean-fold abundance of mRNA evaluated by quantitative polymerase chain reaction (QPCR) from the putative circadian genes: clock [A]; cryptochrome 1 [B]; cryptochrome 2 [C]; cycle [D]; eyes absent [E], and timeless [F] in Acropora millepora seven-day-old azooxanthellate larvae from the 12:12 LD (silver) and 24 DD groups. Shaded regions represent darkness to which DD (black) was exposed for 24 hours. Asterisks represent statistically significant variances (p<0.05) while sampling commenced two hours after illumination (Brady et al. 2011). Analyses courtesy of Brady et al. 2011 and the Creative Commons Attribution License.
clock is promoted/unrepressed during daylight yet its rhythm is repeated when kept in constant darkness and thus may be regulated by an endogenous rhythm. cryptochrome 1 (cry1; cry1a in Nematostella species; Brady et al. 2011) had the greatest daytime upregulation peaking at six hours mid-photoperiod yet it was transcribed nominally in continuous darkness and was therefore at this time considered non-circadian (Fig 5.; Levy et al. 2007; Reitzel et al. 2010). cryptochrome 2 (cry2; cry1b in Nematostella species; Reitzel et al. 2010) exhibited robust upregulation in light peaking late photoperiod which was repeated in continuous darkness and is thus circadian. cycle/bmal (cycle) is a 24-hour rhythmically transcribed gene that peaks nocturnally with a contrasting conspicuous spike two hours after commencement of illumination in the 2009 larval LD group. It is thus controlled by core mechanisms and possibly assumes analogous functions to those elucidated in other animals (Dibner et al. 2010). The circadian transcription factors eyes absent (eya) and six homeobox (six) are associated with core “clock” daylength responses in contrasting organisms (Dardente et al. 2010; Masumoto et al. 2010) where the nearest Acropora homologue is nominally expressed cyclically but more meaningfully at night which appears modulated by an irradiance-entrained rhythm, that peaks again immediately after lights on (Fig 5.). homeobox Prox-1 is pivotal in the stem cell-developed lymphatic system of embryonic teleosts (Hong et al. 2002; Butler et al. 2009; Isogai et al. 2009; Choi et al. 2012; Koltowska et al. 2015; Nicenboim et al. 2015) whereas the homeobox motif is conspicuous in genes that modulate the development of insects and vertebrates (Stryer 1995a). timeless is merely light-responsive inasmuch as it is differentially transcribed after illumination but will not cycle during 24 hours of darkness, although its mRNA is more abundant at night with peaks at 14 and 22 hours post-dawn (Fig 5.; Brady et al. 2011).
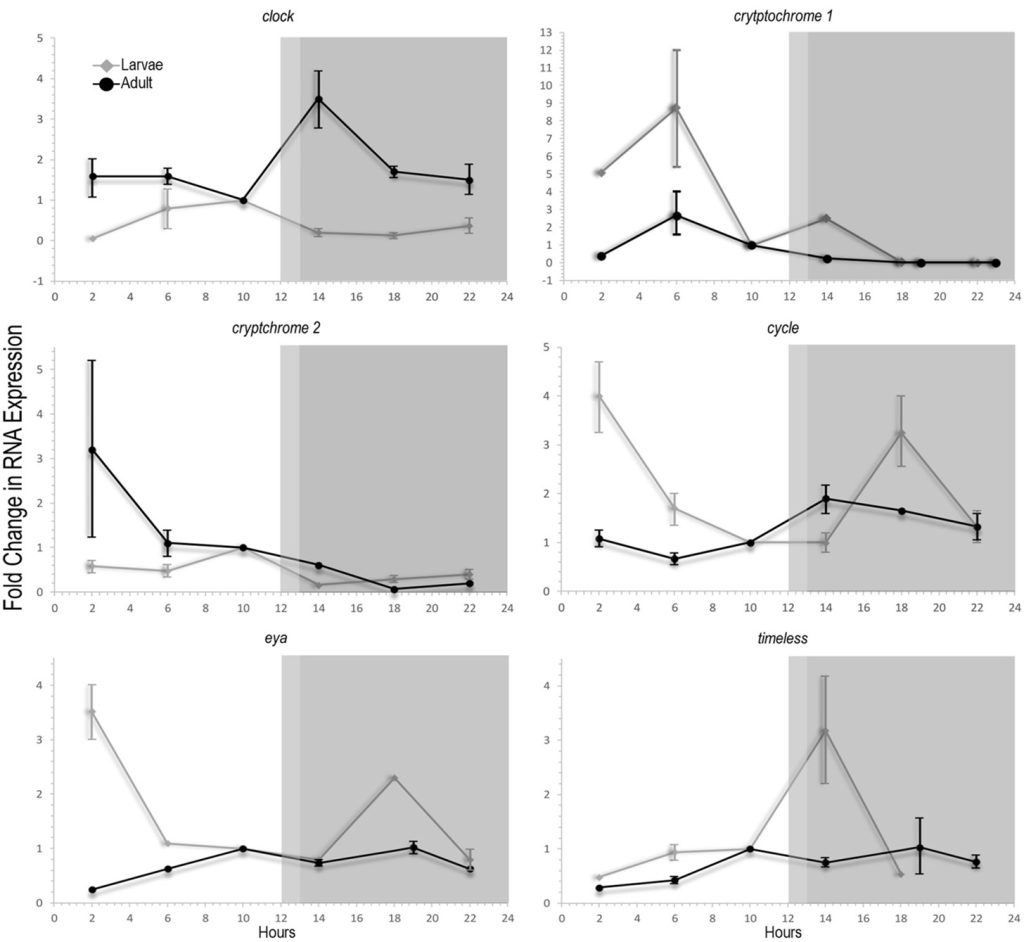
Fig 6. Time in hours versus differential mean-fold abundance of mRNA evaluated by quantitative polymerase chain reaction (QPCR) from the putative circadian genes: clock; cryptochrome 1; cryptochrome 2; cycle; eyes absent (eya), and timeless in Acropora millepora seven-day-old azooxanthellate larvae exposed to 12 hours of light and darkness (12:12 LD; silver) and adult colonies under 13:11 LD (black). Shaded regions represent 12 and 11 hours of darkness. Adapted from the data presented in Brady et al. 2011.
The experimenters were yet to assay mRNA with deep sequencing and QPCR in the tissues of wild adult zooxanthellate colonies of A. millepora maintained ex situ in a native cycle of 13 and 11 hours of light and darkness (LD), since photosynthesis, zooxanthellae, and other microbes may impact gene expression and the endogenous rhythm of their host. These colonies spawned in synchrony with their wild counterparts which is stimulated by variations in daily irradiance, whereas assays were validated by the expression of the primary and secondary control genes: RNA polymerase II and adenosyl homocysteinase. Transcription of clock, cry1, cry2, cycle, eya, and timeless was somewhat commensurate with that of azooxanthellate 12:12 LD larvae, albeit the colonies adopted divergent patterns throughout their 13:11 LD phases (Brady et al. 2011).
- Fig 7. F. fragum‘s “clock” genes throughout diel light cycles. Samples collected between 5 to 6 August 2009 (grey circles) and 31 May to 2 June 2010 (black circles). Featuring relative expression of cry1 [A]; cry2 [B]; clock [C], and cycle [D] where shaded bars indicate nighttime. [E] natural irradiance in lux measured at 5-minute intervals at half a metre’s depth. Graphs and analyses courtesy of Hoadley et al. 2011 and the Creative Commons Attribution Licence.
Figure 6. features a direct comparison between the transcription of clock, cry1, cry2, cycle, eya, and timeless mRNA in the 12:12 LD larval and 13:11 LD adult groups. cry1’s “non-circadian” upregulation was highest peaking at six hours after illumination and reflected the pattern of azooxanthellate larvae, albeit less pronounced. The transcription of the remaining genes was analogous in both larvae and adults albeit a shift of up to five hours was evident when comparing the peaks and troughs. clock reached maximum expression two hours before lights out in larvae, while it was most pronounced in adults an hour after. Daytime peaks in cry2’s expression occurred at two and 10 hours after illumination in adult and larvae respectively yet the upturns originated from six and two hours after lights out. cycle was up- and down-regulated most strongly in larvae where it peaked two hours after illumination and again after six hours of darkness. A similar yet reduced response was observed in adults where mRNA was most plentiful an hour after lights out and thus five-hour nocturnal shifts occurred in larvae. The most profound upregulation patterns generally arose in larvae before adults yet this trend was reversed with cycle. Daytime peaks of eya occurred at two and 10 hours after illumination in larvae and adults respectively, albeit both spiked again six hours after dark. The abundance of timeless mRNA was greatest two or six hours after lights off in larvae or adults which is intriguing because this direct gene is up- and down-regulated in response to daily solar irradiance which was only delayed by an hour but shifted four (Brady et al. 2011).
The experimenters understood the importance of side- and up-welling and thus opted for fluorescent tubes and MH high intensity discharge (HID) lamps rather than LED fixtures. The author aspires to a renaissance in HID technology because LEDs emitting the spectral gamut and an equivalent wattage would be necessary to replicate the irradiance from a 400W MH lamp (Joshi, personal communication) which conserve the most light-demanding of reef-forming zooxanthellate small polyp stony (SPS) hermatypes. LEDs remain experimental while technology has yet to devise a real and economical replacement for traditional reef lighting.
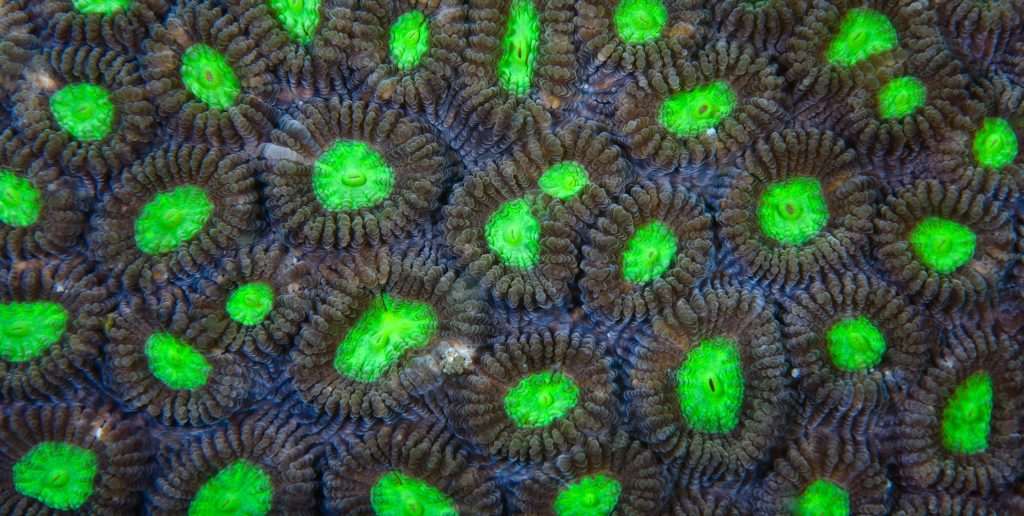
Fig 9. Polyps of remarkably aggressive cf. Favia species (Faviina: Faviidae). See: figure 17.
The genera Acropora and Nematostella diverged five hundred million years ago and thus survived the Permian/Triassic mass extinction when temperatures and atmospheric CO2 were much higher. Homocysteine and serine are catalyzed by cystathionine β-synthase (Cbs) or cystathionase (cystathionine γ-lyase; Cth) to cysteine which is a vital amino acid for protein-stabilizing intramolecular disulphide bridges. However, genes encoding these enzymes are lacking in the genome of Acropora despite homologues in Nematostella anemones, Ctenactis echinata, Favia lizardensis, Favites chinenis, and Galaxea fascicularis (Shinzato et al. 2011). Acropora genomes encode for adenosyl homocysteinase that catalyzes the potent methyl group donator adenosyl methionine to the cysteine precursor homocysteine, yet they appear to lack the concluding enzyme (Stryer 1995). Acropora species must therefore obtain this indispensable and irreplaceable amino acid from holobiont affiliates or zooplanktonic ingestion.
All plots for all genes featured in figure 6. converged at 1-fold differential expression 10 hours into each experiment that is likely an artefact of comparative data set normalization, which is borne out by this point’s lack of mean variance. Larval genes appear more light-responsive despite being exposed to 140 times less irradiance. This trend may reflect “algal”-partner photonic attenuation/sequestration or dissipation of surplus excitation through photoreactive pigment cycling or the other molecular and irradiance-protective mechanisms of mature colonies. Furthermore, zooxanthellae may orchestrate the expression of host circadian genes in concordance with their own.
clock, cryptochrome 2, cycle, and eyes absent appear under the control of an entrained endogenous pacemaker because their expression continued in a rhythmic manner throughout 12:12 LD and 24 DD sampling, whereas cryptochrome 1 and timeless appear ostensibly light-responsive because their transcription lacked iteration in darkness. Peaks of clock and cryptochrome 1 and 2 occur during daylight, whereas spikes of eya, cycle, and timeless occur nocturnally (Brady et al. 2011).
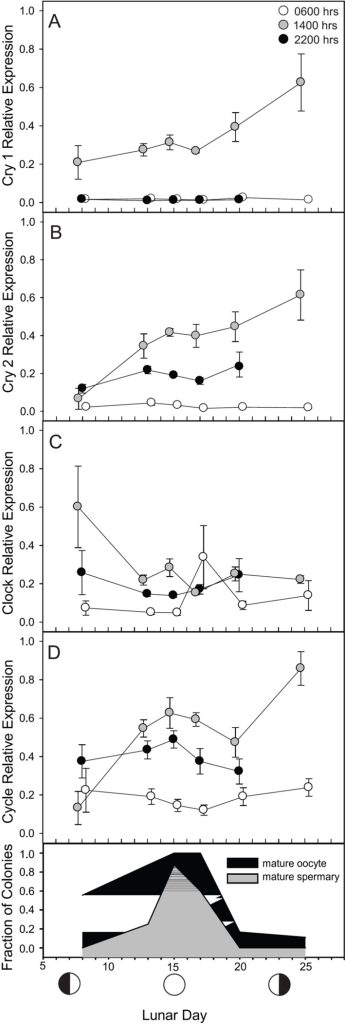
Fig 10. The relative expression of cry1, cry2, clock, and cycle in F. fragum during a lunar cycle where samples were taken at 06:00 (white), 14:00 (grey), and 22:00 (black) from 7 July to 25 August 2009. The fraction of colonies that contained mature oocytes (eggs; black) and sperm (grey) are illustrated on the bottom panel. Graphs and analyses courtesy of Hoadley et al. 2011 and the Creative Commons Attribution License.
Expression and transcription are inequivalent which may or may not result in functional proteins. Messenger RNA (mRNA) lacks stability and is thus ephemeral and polypeptides are manufactured during translation whereafter efficacy relies upon precise folds and post-translational modification. These energy-dependent multifaceted processes take time; hence outcomes are impacted by resource allocation, other vital intracellular cascades, and the bioavailability of molecular signals, co-factors, and ligands (Fig 3.).
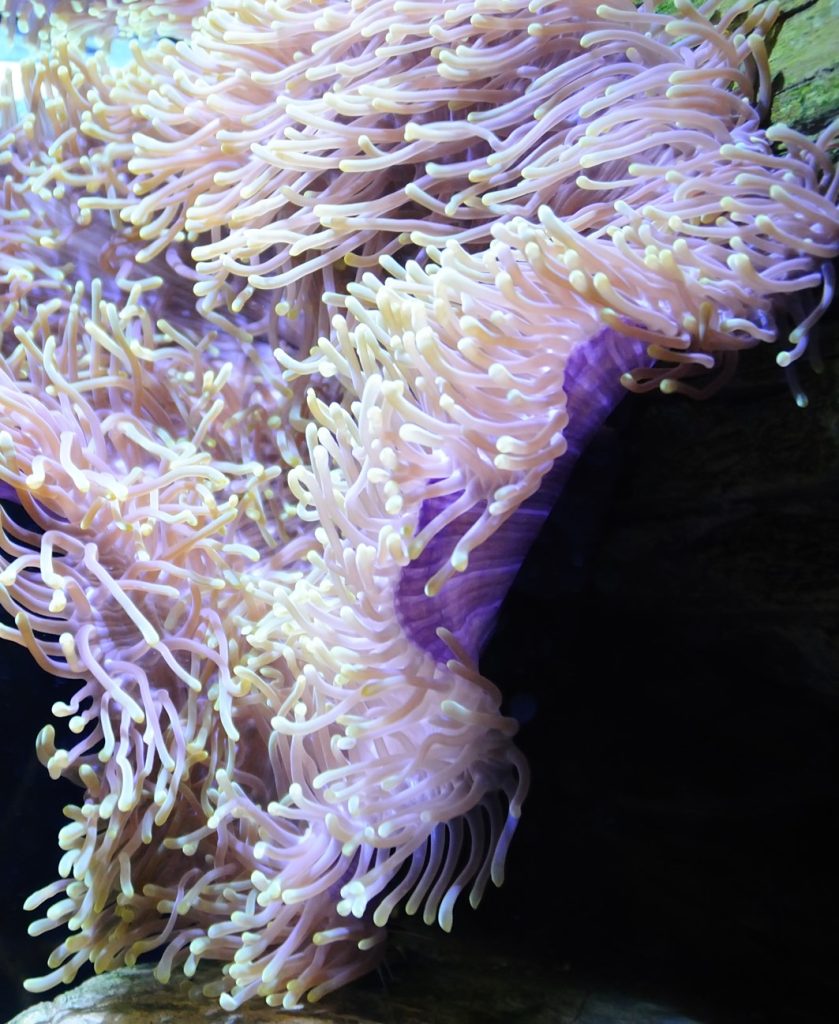
Fig 11. The azooxanthellate starlet anemone, Nematostella vectensis.
Irradiance-responsive direct and light-entrained endogenous circadian rhythms appear to mediate gene transcription in A. millepora’s azooxanthellate seven-day-old larvae and adult colonies with extensive microbial symbionts. Nevertheless, back and forth shifts of several hours in peak expression occur in 13:11 LD adults verses 12:12 LD larvae which may be driven by their divergent daylight cues and cycles. 12 and 13 hours of daylight may thus stagger transcription by as much as five hours. Circadian rhythms are entrained by cues including daylength which are tempered by dynamic transcription-translation feedback loops, where longer photoperiods typically defer expression. Larval and adult groups were also exposed to intensity and spectral disparities such as 150 lux of solar-like irradiance versus 21,000 lux of a correlated color temperature (CCT) of 20,000K (Brady et al. 2011). Diminished irradiance-entrainment causes mRNA profusion-attenuation in other organisms, but peak and trough chronologies remain intact (Hastings & Sweeney 1958; Taylor 1989).
The ectoderm-constrained products of cry1 and cry2 carry an N-terminal photolyase-related region (PHR) with two chromophore binding domains and a C-terminal oligopeptide comprising 54 or 27 amino acids respectively. cry2’s expression is significantly upregulated at midnight on a full moon (LL) but not on a new moon (D) compared with its mRNA abundance at 18:00. This gene’s product may thus be a key player in gametogenesis and spawning. cry3 and cry–dash (Acropora orthologue: dash) were also discovered in A. millepora (Levy et al. 2007).
An earlier study had highlighted the non-circadian (direct) nature of cry1 and cry2 in A. millepora (Levy et al. 2007) yet only cry1 transcription became arhythmic when subjected to 24 hours of darkness, when akin to higher eukaryotes, cry2 exhibited conspicuous periodicity (Fig 5.; Brady et al. 2011). cry2’s expression was rhythmic in the brooding coral Favia fragum over typical days and nights which was lost in continuous darkness and was thus a response to daily photons, yet these patterns were statistically insignificant (Figs 7. & 8.; Levy et al. 2007; Hoadley et al. 2011). Reitzel and associates found a similar direct transcription pattern of cry2 in the azooxanthellate anemone Nematostella vectensis (Fig 16.; Reitzel et al. 2010) where daytime expression was much higher like it was in all studies, whereas the 24 DD plots closely mirror those of the 12:12 LD group in figure 5. which strongly suggests that cry2 in A. millepora is under the control of an endogenous oscillator (Brady et al. 2011).
The cry2 plots of Reitzel and collaborators were not the only to contrast with those of Brady and colleagues. timeless transcription (timeout in Nematostella; Reitzel et al. 2010) exhibited a pronounced peak in the larval 12:12 LD groups shortly after lights out which was not evident in 24 DD or adults (Figs 5. & 6.). This clearly indicates A. millepora’s timeless is non-circadian and light-responsive like it is in Drosophila species (Sehgal et al. 1995) whereas the timeout plots of Reitzel and associates were unremarkable which may reflect Acropora’s and Nematostella’s interspecific divergence (Reitzel et al. 2010; Brady et al. 2011).
Notwithstanding LD and DD curves conflicting during daylight, a pronounced peak of cycle mRNA occurred in both groups six hours after lights out, which verifies a robust entrained rhythmicity (Brady et al. 2011). Daytime synchronized non-circadian peaks of cycle transcripts were observed in the LD groups of F. fragum (Fig 8.) albeit diurnal expression was arhythmic (Fig 7.; Hoadley et al. 2011) like it was in N. vectensis (Reitzel et al. 2010). These findings are exceptional insofar as the roles of near-match homologues are typically conserved amongst related organisms (Brady et al. 2011) yet this fidelity may be weakened in genes that confer immunity (Aslett 2024).
Peak clock expression occurs in late afternoon in the genera Acropora (Brady et al. 2011) Nematostella (Reitzel et al. 2010) and Favia (Hoadley et al. 2011) while clock’s circadian rhythmicity appears lacking in the latter two (Reitzel et al. 2010; Hoadley et al. 2011). Nevertheless, this gene is circadian in Acropora like it is in fruit flies where its mRNA profusion is greatest after dawn (Fig 5.; Brady et al. 2011).
The experimenters remarked that QPCR and deep sequencing are not affected by the inconsistencies and external factors that impact conventional PCR, albeit their cost is prohibitive inasmuch as it constrains the number of replicates and sampling points. Some mRNA may have peaked and degraded within three and a half hours which will be missed using the current protocol (Brady et al. 2011).
Table 2. features daytime gene ontology (GO) clustering pathways ascertained using gene set enrichment analysis (GSEA) software for Acropora orthologues matching 26 sets with implicated roles in the genome of Nematostella species. The data is merely provisional where comprehensive transcriptome annotation and extensive assays under various illumination strategies are warranted. GO clustering was determined by blasting A. millepora’s contig cDNA library against a machine annotated Nematostella genome where such data comprises errors many of which are worsened during BLAST (Brady et al. 2011). Notwithstanding, the study unearthed some conspicuous pathways in other animals such as murine mitosis regulation (Tamai et al. 2008) whereas light-phase retinal metabolic processes and rhodopsin gene expression occur in the pineal glands of zebrafish (Toyama et al. 2009). The DNA photolyase gene set exhibited the most significant differential expression from night to daylight (Levy et al. 2011; Brady et al. 2011) which may be due to its derivative cryptochromes (Brady et al. 2011) while both studies highlighted the daytime upregulation of mitochondrial respiration as well as the diel rhythm of mitotic cell cycle (Brady et al. 2011; Levy et al. 2011). Coral bioinformatic and genomic data bases were barely established at this time.
Sizeable changes in expression occur over 24 hours in adult A. millepora and their larvae where 13- versus 12-hour photoperiods appear to exert meaningful impacts. Some genes exhibiting patterns of diurnal expression encode for acknowledged circadian components which are under the control of an entrained endogenous “clock”, whereas others respond directly to light which lose their rhythmicity when kept in darkness. These findings contribute significantly to this complex and often confounding discipline that assist the elucidation of the chronobiological mechanisms that underpin the wellbeing and fecundity of reef-forming corals (Brady et al. 2011).
Viviparous Caribbean Favia fragum are hermaphrodite members of the extraordinarily aggressive suborder Faviina (Fig 17.; Lang 1973; NCBI 2019) that sexually reproduce monthly. Sperm are broadcast environmentally and are later internalized where they fertilize oocytes, while zygotes are brooded by colonies until free-swimming zooplanktonic planulae are released (Shlesinger et al. 1998; Thompson et al. 2015). Nurture of this kind exerts a meaningful impact on survivorship.
Healthy Favia fragum colonies with at least 20 polyps were collected off western Isla Magueyes in Puerto Rico at 06:00, 14:00, and 22:00 on day 7 to 15 of a lunar cycle starting on 29 July and continuing 3, 5, 7, 10, and 15 August 2009. Further colonies were collected every four hours for a total of 24 on the full moon of 5 August 2009 and on 31 May 2010 every four hours for a total of 60. RNA was extracted and preserved from half colonies while the remaining halves were histologically prepared for microscopic evaluation. Sampling days were chosen to capture transcription during key events like embryogenesis from day 15 to 20 and the full moon, where previous spawning windows occurred at dusk in F. fragum (Szmant-Froelich et al. 1985) and at midnight in A. millepora (Levy et al. 2007; Hoadley et al. 2011).
60 colonies from the 31 May collections were housed in temperature- and illumination-monitored outdoor ponds exposed to natural light and a flow through supply of Puerto Rican reef water. 30 corals were moved to a continuously dark pond after 24 hours. RNA was extracted from five colonies after 72 hours and further samples were taken at 10:00, 14:00, 18:00, 02:00, 10:00, 14:00 and 18:00 over two days (Hoadley et al. 2011).
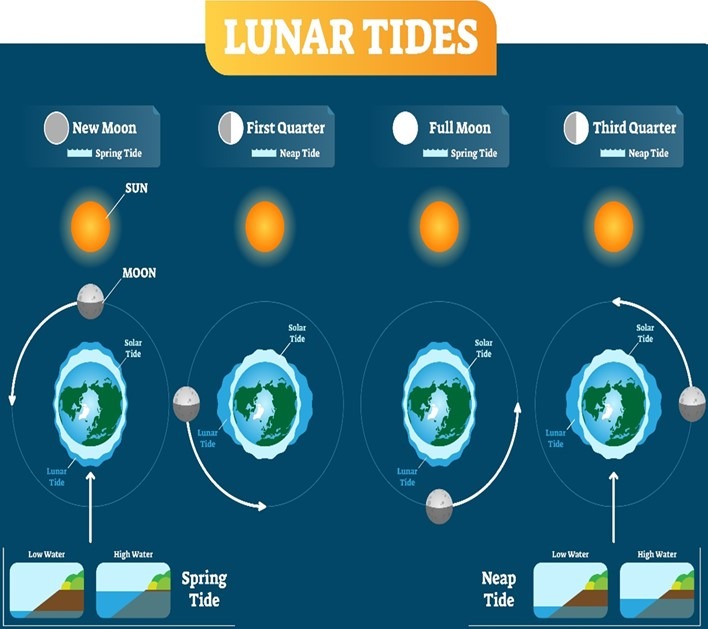
Fig 12. The spring and neap tides occurring on lunar quarters.
The analytical techniques used to extract RNA, prepare a cDNA library, BLAST orthologues, and evaluate transcription were not dissimilar to those exploited by Brady and colleagues of the same year.
Figures 7. and 8. illuminate Hoadley and collaborators’ relative expression of cry1, cry2, clock, and cycle within the tissues of F. fragum, whereas figure 10. features gene transcriptional analyses over a lunar cycle.
Hoadley and colleagues discovered inconsistencies in the cryptochrome descriptors of A. millepora and N. vectensis and thus adopted the nomenclature of Levy and allies from 2007. F. fragum’s cry1 corresponds to the cry1 of A. millepora but is a near match for the cry2 of N. vectensis, while the cry2 of F. fragum is a primary homologue of A. millepora’s cry2 and the cry1a and cry1b of N. vectensis. Nevertheless, these anthozoan genomes encode for clock and cycle orthologues (Hoadley et al. 2011).
Figure 7.: cry1 peaked in the morning compared with its nocturnal and sunup and sundown transcription, whereas cry2’s expression was highest at dusk as opposed to dawn and night. clock mRNA was also more abundant at dusk compared with morning and dawn. cycle was upregulated at night in contrast to dawn and midday in the flesh of colonies from 5 August 2009, yet significant diurnal expression was lacking in the plots for the generous samples from May 31 to 2 June. All differential expression was statistically significant (p<0.05) apart from that of May’s cycle (Hoadley et al. 2011).
Figure 8.: light and dark comparative expression data for cry1, cry2, clock, and cycle were generated for colonies exposed to tropical days and nights (LD) and those kept in utter darkness (DD). cry1 was upregulated in the morning whereas cry2 and clock were elevated at dusk in comparison to night and dawn where these differences were statistically significant (p<0.001). Yet transcription of these three genes became arhythmic during continuous darkness, albeit cry1’s mRNA was enriched compared to that of a typical nighttime (p<0.001). Merely the differential expression of cycle in LD and DD groups proved insignificant (p=0.52) but p was <0.05 for both groups’ when considering the nominal change in transcription observed between midday and nighttime. That said, such midday upregulation was not evident the next day which is likely due to the stochastic transcription of cycle in this study (Hoadley et al. 2011) which contrasts with Brady and colleagues’ plots of this gene in A. millepora (Fig 5.; Brady et al. 2011).
cry1 mRNA was enriched at 10:00 while cry2 and clock transcripts peaked at 18:00, but dwindled to nominal at night, where cycle’s expression was arhythmic throughout. These findings somewhat concur with those of Levy and colleagues for cryptochrome in A. millepora and Reitzel and allies for cryptochrome, clock, and cycle in N. vectensis (Hoadley et al. 2011). cry1 and cry2 orthologues in A. millepora were upregulated in response to light (Levy et al. 2007), whereas cry1a and cry1b (F. fragum ortholog: cry2) and clock peaked mid-photoperiod and just before sunset (18:00) respectively (Reitzel et al. 2010). cycle expression remained light insensitive (Hoadley et al. 2011).
Disordered tentacle extension and retraction in perpetual darkness coincided with a lack of peak transcription of cry1, cry2, and clock in the DD groups (Hoadley et al. 2011).
F. fragum’s cry1 and cry2 mRNAs were significantly enriched in daylight (Figs 7. & 8.) while the 24-hour plots of cry2 in N. vectensis (F. fragum orthologue: cry1) remained entropic (Hoadley et al. 2011).
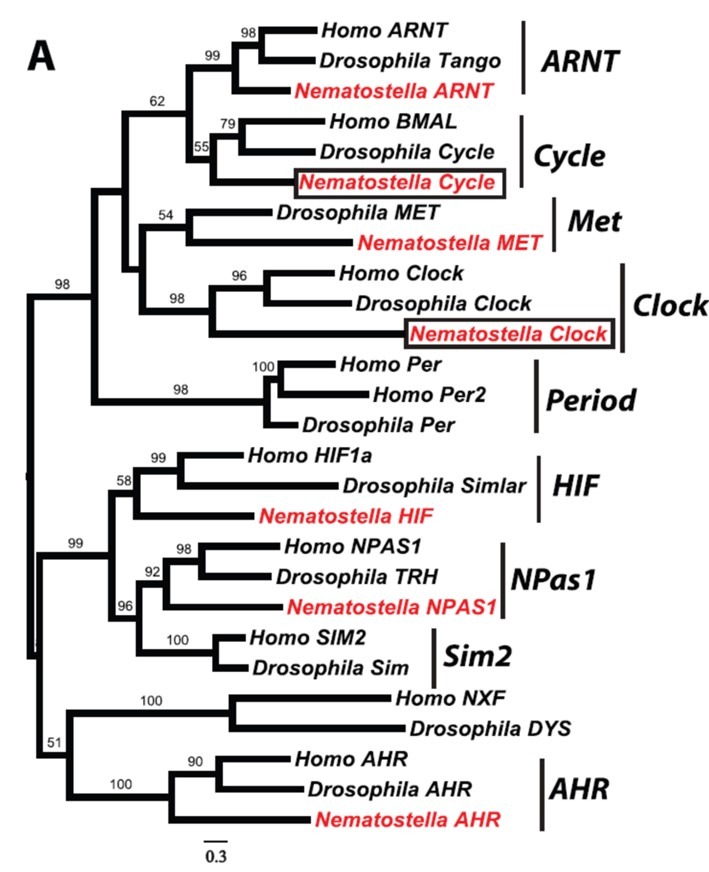
Fig 13. A phylogenic tree of some conserved circadian genes and bHLH-PAS orthologues carried by Nematostella vectensis, Homo sapiens, and Drosophila melanogaster. The bHLH-PAS transcription factors clock and cycle are boxed along with other interspecific orthologues highlighted in red (Lin & Todo 2006; Reitzel et al. 2010). Analyses painstakingly verified by the percentage of 1,000 bootstraps above each node. Findings and tree courtesy of Reitzel et al. 2010 and the Creative Commons Attribution License.
Figure 10.: the transcription of these “clock” genes appeared unrelated to the timekeeping of gametogenesis or spawning. cry1 and cry2 mRNAs were enriched at 14:00 compared to their diminishment at 06:00 and 22:00, whereas clock peaked at 14:00 and 22:00 in contrast to its expression at dawn. There was an insignificant accumulation of cry1 transcripts over the lunar sampling period, while cry2 mRNA was more abundant at 14:00 on day 20 and 25 compared to day 8 (p<0.01). clock expression did not change significantly from lunar day-to-lunar day (p=0.369) whereas cycle transcription varied throughout the day over 25 days and was upregulated on days 15, 20, and 25 (p<0.05; Hoadley et al. 2011). Transcripts of A. milllepora’s cry2 were enriched at midnight on the day of a spawning full moon but this pattern was not repeated on non-spawning full or new moons (Levy et al. 2007). However, it is possible that cryptochrome mRNA peaked and degraded during the eight-hour sampling interim (Hoadley et al. 2011).
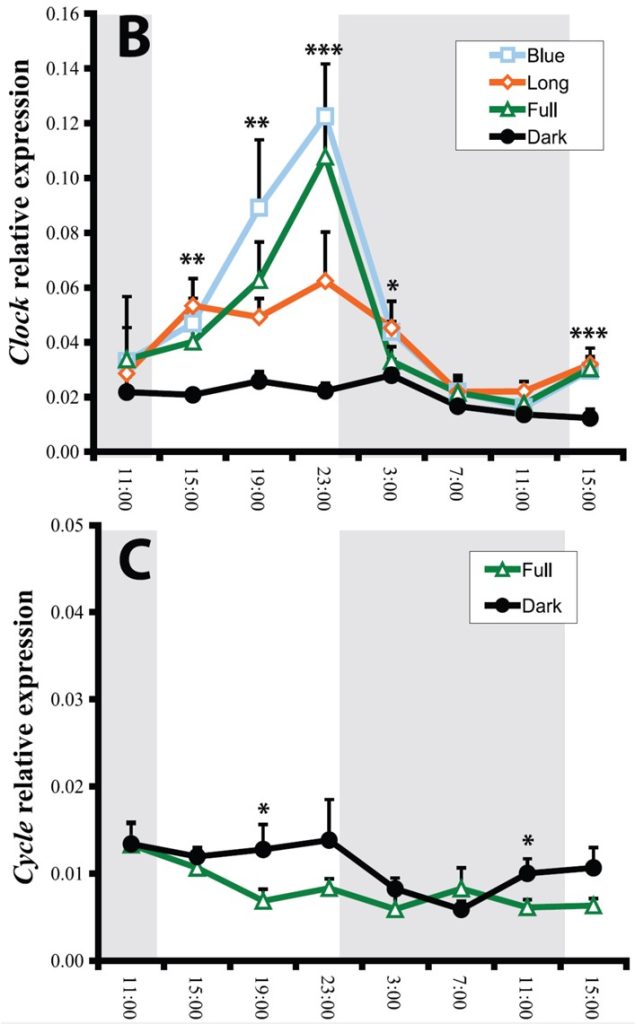
Fig 14. [B] the relative diel expression of the clock orthologue of N. vectensis exposed to short and long wavelength, full spectral irradiance, and continuous darkness. [C] the expression of anemone cycle kept in utter darkness or irradiated with diurnal full spectrum light. Shaded regions represent nighttime in light:dark (LD) groups. Analyses and graphs courtesy of Reitzel et al. 2010 and the Creative Commons Attribution License.
Oocytes and spermaries (@testes) peaked at day 15 which were absent by day 20 and thus corals had spawned (Hoadley et al. 2011). F. fragum reproduced immediately after the full moon albeit there was a nonreproduction-related protracted increase of cry2 and cycle that peaked 10 days later (p<0.05). The F. fragum colonies in this study appeared to have an analogous gametogenesis and spawning rhythm as those previously described by Szmant-Froelich and colleagues in 1985 (Hoadley et al. 2011) which robustly responds to diurnal irradiance (Fritzenwanker & Technau 2002).
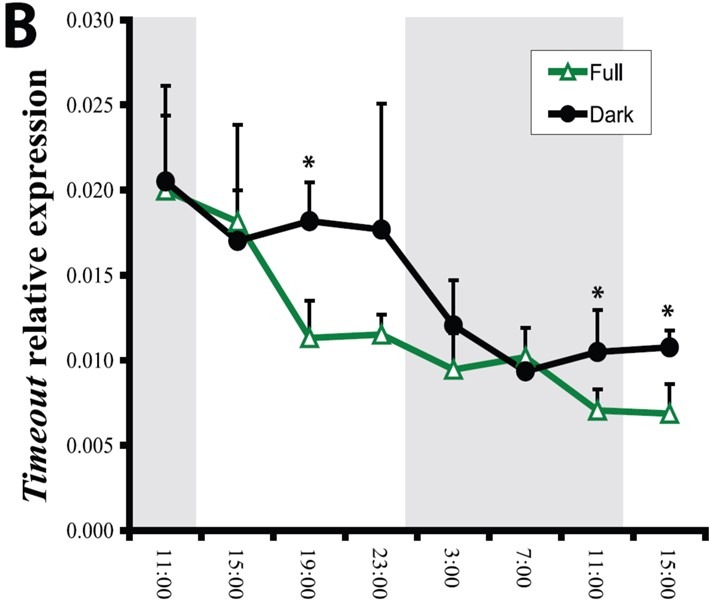
Fig 15. The relative expression of the N. vectensis orthologue, timeout in LD full spectrum and DD groups. Analyses and graph courtesy of Reitzel et al. 2010 and the Creative Commons Attribution License.
The tissues of zooxanthellate Scleractinia undergo a photosynthesis-derived radical downturn in oxygen availability and pH (hypoxia) immediately after they are plunged into darkness (Kuhl et al. 1995). Genes associated with glycolysis in A. millepora are expressed in a cyclic manner during typical day and night illumination yet lose this rhythm in prolonged darkness, which are ostensibly controlled by an orthologue encoding hypoxia-inducible 1α transcription factor (Levy et al. 2011). All things considered, it is likely that coral endogenous “clocks” are entrained or influenced by their tissue REDOX and ultimately their “algal”-partners. Hence comparative analysis of the substantiated circadian genes of F. fragum and N. vectensis in relation to the oxic status of their flesh may prove enlightening (Hoadley et al. 2011).
The transcription patterns of the putative circadian genes of N. vectensis were assayed during a typical light and dark phase where the study determined the emission spectrum that best stimulated differential expression in this azooxanthellate species. Furthermore, the study discovered proof of feedback loop-associated heterodimerization of pivotal bHLH-PAS proteins and canonical E-box motifs in the promoters of the rhythmically transcribed genes: clock and cry1a (Reitzel et al. 2010).
N. vectensis lacks orthologues encoding the nuclear receptors NR1D1 (Rev-Erb) and NR1F1 (ROR) which are key components of mammalian endogenous regulators (Reitzel & Tarrant 2009; Reitzel et al. 2010). clock mRNA cycles rhythmically throughout the day and night yet peaks midday to late evening in N. vectensis. These anemones typically burrow in shallow estuarine environments yet they may be suited to meso- or a-photic depth where there is a profusion of zooplankton, because they do not have zooxanthellae and are thus reliant upon heterotrophy to satisfy their needs. However, clownfish can prove nutritionally advantageous, while the light-responsive transcription of these anemone’s is most pronounced and deferred under blue or blue-comprising full spectrum irradiance (Reitzel et al. 2010).
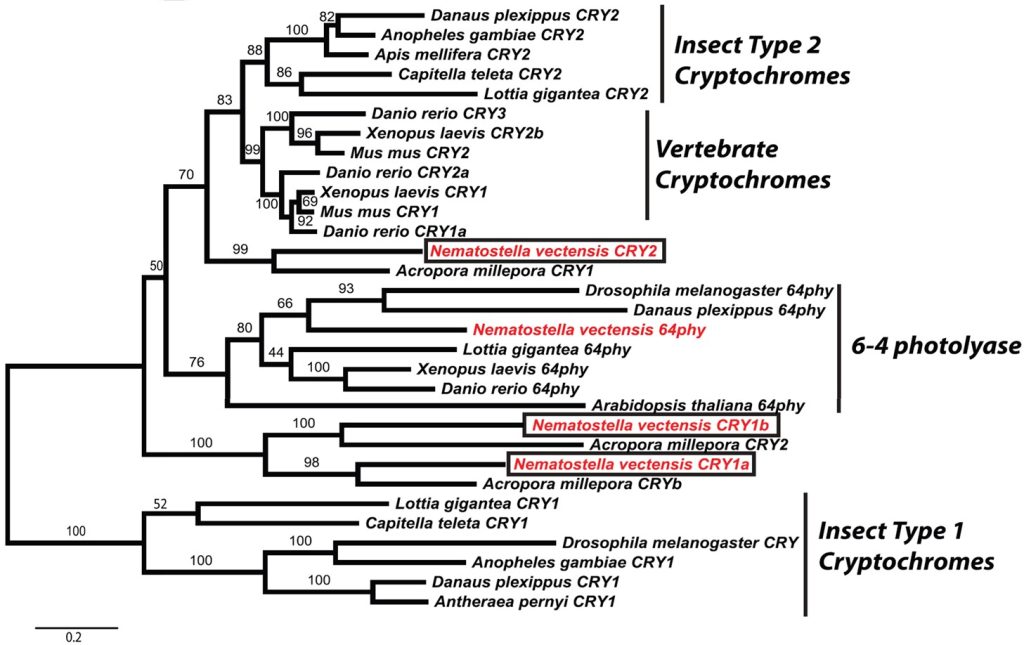
Fig 16. [A] a phylogenetic tree featuring the lineages of conserved circadian orthologues of Nematostella vectensis (red) and boxed cryptochromes: cry1a, cry1b, and cry2 where rigorously verified analyses reveal the percentage of 1,000 bootstraps above each node. Comprises coral, fruit flies, xenopus toads, zebrafish, and mosquitos. [B to D] the relative expression of cry1a, cry1b, and cry2 of N. vectensis irradiated in a daytime and nighttime rhythm (LD) with short and long wavelength and full spectrum light. Each graph traces the relative transcription of each gene in the tissues of anemones kept in utter darkness (DD). Analyses and graphs courtesy of Reitzel et al. 2010 and the Creative Commons Attribution License.
The CLOCK:CYCLE dimer upregulates transcription of period genes in mammals, while it exerts similar effects on period and timeless in insects. Such dimerization occurs in N. vectensis yet no pairing was observed between CLOCK and the bHLH-PAS transcription factor ARNT. Such findings suggest that CLOCK:CYCLE dimeric transcription regulators are ancient and were integral to the circadian rhythms of primordial animals (Reitzel et al. 2010).
Merely one orthologue from the timeless-timeout family occurs in most forms of life, while two are carried by fruit flies (Benna et al. 2000; Rubin et al. 2006, cited in Reitzel et al. 2010). Most genes of this family are orthologues of insect timeout synonymous with the timeless of vertebrates. However, both orthologues occur in the sea urchin Stronglyocentrotus purpuratus (Rubin et al. 2006, cited in Reitzel et al. 2010) and thus a prehistoric duplication followed by divergent evolution may have led to developmental gene reduction. Even so, the timeout orthologue of N. vectensis suggests the gene was duplicated after Cnidaria and Bilateria diverged (Reitzel et al. 2010).
timeout’s expression does not respond cyclically to light in N. vectensis, but appears diurnally rhythmic in Drosophila species due to CLOCK:CYCLE promotion (Fig 15.; Sehgal et al. 1995, cited in Retizel et al. 2010; Lee et al. 1998). timeless is a canonical circadian gene in several insects yet a copy is lacking in others (Reitzel et al. 2010). It is required for chromosome stability in mice (Benna et al. 2010) and the replication fork complex of mammals, where TIMELESS and TIPIN bind cooperatively which is likely to be its original purpose (Gotter et al. 2007; Reitzel et al. 2010). tipin is integral to the N. vectensis genome which suggests its product may lend stability to the replication fork of DNA (Fig 18.; Reitzel et al. 2010).
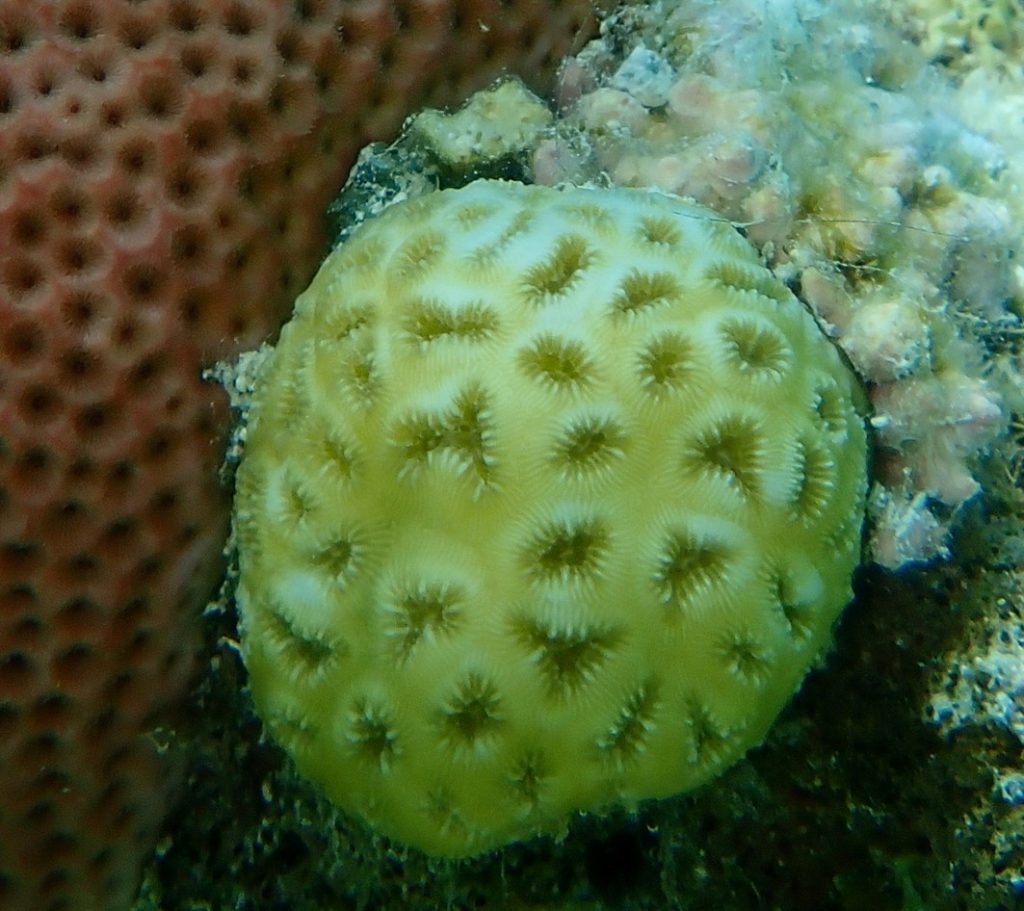
Fig 17. Golf ball coral, Favia fragum.
The circadian function of TIMELESS in N. vectensis remains enigmatic but like that of mammals and insects, it has domains for cytoplasmic translocation and PERIOD interaction but lacks the DEDD moiety of the former and a nuclear importation domain. DEDD is conserved in insect and mammalian TIMELESS, while the absence of anemone period means there is nil evidence of PERIOD:TIMELESS repression (Reitzel et al. 2010).
Three genes encoding cryptochromes are carried by N. vectensis. Two originate from a putative prehistoric cnidarian duplication that developed into a type 1 (cry1-like) and a 6-4 photolyase-like homologue normally associated with DNA repair. The third appears to be an orthologue of insect and vertebrate cry2 (Reitzel et al 2010).
All three kinds of emissions upregulated cry1a’s expression several fold albeit blue light elicited the most significant response followed by solar-like irradiance. The transcriptional increase commenced just after lights on and continued to climb until lights out whereafter expression declined. The abundance of cry1a mRNA in the DD group somewhat mirrored the expression of the LD groups, however, the curve was so diminished it was concluded the gene was non-circadian (Fig 16.; Reitzel et al. 2010).
According to later research, cry1a of N. vectensis is an orthologue of A. millepora’s cry1 (Fig 6.; Fig 7.; Brady et al. 2011) yet a contrasting study suggested the cry1 of Favia fragum is an orthologue of cry1 of Acropora millepora that are cry2 equivalents in N. vectensis. Moreover, it was suggested the cry2 of F. fragum is a primary homologue of A. millepora’s cry2 and the cry1a and cry1b of N. vectensis (Fig 7.; Fig 8.; Hoadley et al. 2011). The current study proposed that cry2 and cry1b of N.
vectensis are ostensibly the respective homologues of cry1 and cry2 in A. millepora (Reitzel et al 2010). Contradictions such as these likely reflect inaccuracies in gene annotations and sequence tags and underpopulated bioinformatics, which will be resolved in the proceeding editorials when we explore current knowledge. Nevertheless, the author has presented the findings and postulations as they appear.
The cry1b mRNA abundance in N. vectensis (A. millepora subjective orthologue: cry2) generated analogous rhythmic curves in all treatments where short wavelength blue, full spectrum, long wavelength red, and total darkness resulted in the most to the least amplitude. cry1b mRNA upsurges occurred from lights on and peaked from late morning to early afternoon then declined until lights out when they plummeted. Similar plots were recorded for A. millepora’s cry2 by Brady and colleagues in 2011 (Fig 5.) which also responds to moonlight (Levy et al. 2007) which comprises short wavelength blue (Johnsen et al. 2006). The expression of cry1b in N. vectensis and cry2 in A. millepora appear ostensibly upregulated in response to the precise wavelengths reflected by a full moon (Fig 16.; Reitzel et al. 2010).
Conversely, the transcription of cry2 in N. vectensis was entropic and arhythmic which contrasted with the findings of its subjective orthologue cry1 in A. millepora (Fig 5.; Fig 6.; Brady et al. 2011) and cry1 in F. fragum (Fig 7.; Fig 8.; Hoadley et al. 2011) inasmuch as cry1 of F. fragum cycled rhythmically in response to diurnal irradiance and there were pronounced peaks during daylight in A. millepora’s (Figs 5. to 8.; Fig 16.; Reitzel et al. 2010; Brady et al. 2011; Hoadley et al. 2011). The intracellular mechanisms that underpin expression of cry2 in N. vectensis and cry1 in A. millepora appear to have evolved divergently, yet the arhythmic abundances of cry2 mRNA in diurnally lit N. vectensis precludes its role as a photoreceptor (Reitzel et al. 2010).
The sequences of cry2 of N. vectensis and cry1 of A. millepora appear to have evolved from genes encoding 6-4 photolyases akin to the type 2 cryptochromes of insects and vertebrates. 6-4 photolyase homologues are associated with transcription suppression in insects and mammals. The CLOCK:CYCLE dimers of mammalian positive feedback loops are equilibrated by PERIOD:CRYPTOCHROME repression (Reitzel et al. 2010) yet Cnidaria lack period. Hence genes derived from homologues encoding 6-4 photolyase may assist cryptochrome downregulation. Nevertheless, the diurnal expression of cry2 in insects is equally inconclusive where it acts as a suppressor and may thus adopt analogous roles in Anthozoa (Reitzel et al. 2010).
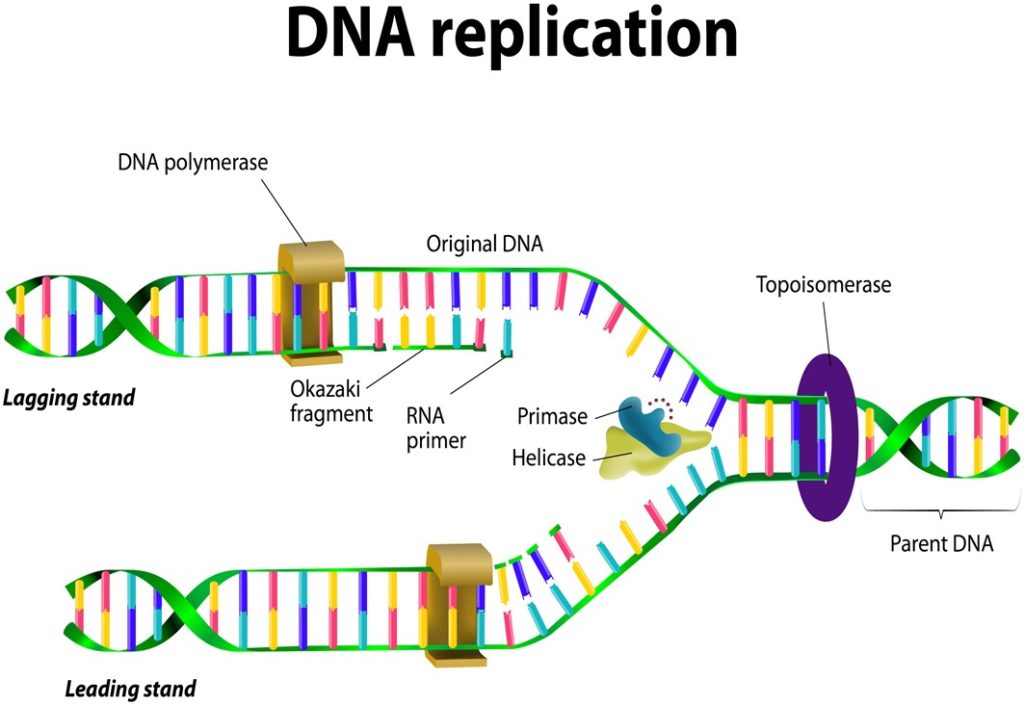
Fig 18. An illustration of DNA replication.
clock, cycle (homologue: arnt), and cryptochromes were discovered in sponges (Simionato et al. 2007; Muller et al. 2010) which also respond to light (Amano 1988). Sponge holobionts are highly diverse where many affiliates are photosynthetic (Munn 2019) where their microclimates ideally range from oxic to microoxic.
CLOCK:CYCLE dimers bind to E-box motifs (CACGTG) to promote downstream transcription in mammals and insects. The experimenters thus searched for upstream E-boxes associated with gene promoters and found two such binding sites: one for clock and the other for cry1a of N. vectensis. cry1b also has an E-box 1,800 base pairs (bp) upstream of its start. Toll-like receptor (TLR) activation leads to the formation of nuclear factor (NF)-kappa-B (kB) which enters the nucleus and promotes transcription (Miller et al. 2007) whereas one study discovered the specificity of the NF-kB binding motif is extraordinarily conserved in Nematostella compared with other animals. The NF-kB dimer binds to the Rel homology domain (RHD) which is encoded on the genomes of N. vectensis and A. millepora which may have evolved over seven-hundred million years ago (Sullivan et al. 2009). These findings implicate CLOCK:CYCLE dimers in the promotion of the rhythmically expressed genes of N. vectensis: clock, cry1a, and cry1b (Reitzel et al. 2010) which associates CLOCK with its bioavailability.
To learn more, visit our website HERE, where we continue to elucidate the underlying mechanisms that control coral biorhythms including a 12.4-hour circatidal “clock” in a- and -zooxanthellate Scleractinia and anemones.
Appendix I Glossary
Allele refers to different forms of a gene.
When symbiotic organisms live separately it is called Aposymbiosis.
Autotrophic organisms satisfy their nutritional requirements from simple inorganic chemicals and usually but not exclusively light.
Azooxanthellate is an organism without symbiotic dinoflagellate “algal”-partners.
The infrakingdom Bilateria is a clade of bilaterally symmetrical animals with an anterior, posterior, dorsum, ventrum, and flanks like dogs, humas, flukes, and mice.
Bootstrap replicates are equal-sized multiple sequence aligned fictional substitute nucleic acids with confidence is proportional to percentage identity.
Canonical means substantiated or acknowledged.
Clade describes a class of organisms comprising only the descendants from a common ancestor.
Codons are triplet bases in mRNA that encode for one kind of amino acid.
Contigs are DNA molecules made up of smaller overlapping oligonucleotides.
Diurnal simply means daily or daytime, albeit contextually it refers to a Diel cycle of typical daylight followed by nighttime analogous darkness.
Endocrine refers to hormone-secreting ductless suprarenal, thymus, thyroid, parathyroid, pituitary, pancreas, ovary, and testis glands.
Endogenous factors originate from within.
Exon is a gene integral coding sequence separated by an Intron (Dale 1998).
Expression see mRNA.
Gonochoric organisms manifest as female or male.
Hermatypic and Ahermatypic Corals – the terms hermatypic and ahermatypic have been misused. Irregularities such as sea fans possessing rigid skeletons are constructive, but they may or may not contain symbionts and do not contribute to reef substratum. Ahermatypes may have large or small polyps and do not contain zooxanthellae. Scleractinian corals are “true” reef builders but may be azooxanthellate or contain symbionts. The terms Scleractinia and hermatype are not interchangeable. Hermatypic corals contain zooxanthellae and are constructive. Ahermatypes are azooxanthellate and non-constructive. This clear definition also applies to non-Scleractinia (Schuhmacher & Zibrowius 1985).
Heterotrophs cannot utilize inorganic carbon for growth so they must dine on organic plant or animal derived nourishment. Their sources include glucose, yet several microscopic taxa retain the capacity to transform inorganic compounds which are frequently used as substrates for chemical reactions.
Intron is a gene-integral interrupting (intervening) sequence which is removed by splicing (Dale 1998).
Mixotrophs utilize both autotrophic and heterotrophic metabolisms.
mRNA stands for messenger ribonucleic acid which is transcribed in the nucleus from an organism’s active genes before translocation to the cytoplasm where a ribosome translates its sequence and concatenates amino acids from transfer ribonucleic acids (tRNAs) into a polypeptide that later folds into a functional protein. See figure 3.
Nucleic Acid is typically molecular ribonucleic acid (RNA) or deoxyribonucleic acid (DNA) which maybe complementary (cDNA), double or single stranded (dsDNA or ssDNA).
An Oligonucleotide is a short, typically synthetic nucleic acid.
Ontogeny/Ontogenesis describes the development of an organism from the moment of fertilization to a mature adult.
Ontology is used contextually to describe the intra- and extra-cellular, ana-, cata-, and metabolic pathways associated with an organisms day-to-day housekeeping, homeostatic, and reproductive processes.
Open Reading Frames (ORFs) are the transcriptional parts of genes that lack start and stop sequences but encode for triplet bases (codons) which correspond to each kind of amino acid.
Orthologue refers to a class of sequenced and characterized genes integral to an elucidated pathway in numerous organisms: orthologues, orthologous.
Paralogues refers to two copies derived from a single ancestral gene residing at two distal loci in the same genome.
Phenotype, see Phenotypic.
Phenotypic Plasticity foregoes the accepted developmental and evolutionary paradigms and expedites swift morphological responses to environmental change.
Phenotypic traits are an organism’s observable characteristics which include how it interacts with its environment, that originate from its epigenotype and genotype.
Photic Zones are sea surface regions extending to approximately 120 m that receive sufficient light to support photosynthetic organisms.
A Phylogenetic Tree “is a graphic representation of the interrelations and evolutionary history of a group of organisms, indicating the relative order of successive divisions of the line of descent, coincident with speciation events” (Wallace et al. 1996a).
Phylogeny facilitates organism classification by comparing the sequences of identical or very similar genes (homologues).
Planulation refers to the release of free-swimming mature larvae from brood colonies.
Plasmid is a self-replicating typically circular and mostly prokaryotic extrachromosomal molecule of ss or dsDNA. See: Nucleic Acid.
Spawning – countless marine organisms broadcast their sperm and eggs into the water that later fuse and ultimately transform into planktonic larvae. Corals are gonochoric or hermaphroditic where brood colonies internalize sperm released by others which fertilizes oocytes (eggs) where blastulas and later planulae are nurtured until free-swimming when they are released. Other corals liberate sperm and egg bundles that float leisurely to the surface where they degrade and release their cargo (Wallace et al. 1986), or colonies broadcast eggs and sperm directly that fuse and develop into zooplanktonic planulae.
Thyroid-stimulating hormone (TSH) beta is manufactured by the pituitary gland in response to low serum concentrations of thyroxine and thus regulates thyroid gland activity.
Transcription see mRNA.
A Transcriptome is the sum of all the messenger ribonucleic acid (mRNA) transcribed from an organism’s active genes (@ORFs; Lexico 2019).
Zooxanthellate is an organism with symbiotic dinoflagellate “algal”-partners.
Zygotes are formed when the nuclei of a sperm and egg fuse which occurs after a spermatozoan penetrates an oocyte (ova) whereafter additional fertilization cannot occur.
References
Abe, V. (1939) On the expansion and contraction of the polyp of a reef coral Caulastrea furcata Dana. Palao Tropical Biological Station Studies. 1, 651-670.
Amano, S. (1988) Morning release of larvae controlled by the light in an intertidal sponge, Callyspongia ramosa. Biol Bull. 175, 181-184.
Aslett, C., G., (2024) Coral Immunity Part II. Reef Ranch Publishing Ltd. https://www.reefranch.co.uk/
Babcock, R., C., Bull, G., D., Harrison, P., L., Heyward, A., J., Oliver, J., K., Wallace, C., C. & Willis, B., L. (1986) Synchronous spawnings of 105 scleractinian coral species on the Great Barrier Reef. Mar. Biol. 90, 379-394.
Bae, K., Lee, C., Sidote, D., Chuang, K., Y. & Edery, I. (1998) Circadian regulation of a Drosophila homolog of the mammalian clock gene: PER and TIM function as positive regulators. Mol Cell Biol. 18, 6142-6151.
Baird, A., H., Guest, J., R. & Willis B., L. (2009) Systematic and biogeographical patterns in the reproductive biology of scleractinian corals. Annual Review of Ecology Evolution and Systematics. 40, 551-571.
Benna, C., Bonaccorsi, S., Wulbeck, C., Helfrich-Forster, C., Gatti, M. et al. (2010) Drosophila timeless2 Is required for chromosome stability and circadian photoreception. Curr Biol. 20, 346-352.
Benna, C., Scannapieco, P., Piccin, A., Sandrelli, F., Zordan, M. et al. (2000) A second timeless gene in Drosophila shares greater sequence similarity with mammalian tim. Curr Biol. 10, R512-R513.
Blau, J. & Young, M., W. (1999) Cycling vrille expression is required for a functional Drosophila clock. Cell. 99, 661-671.
Boden, M., J. & Kennaway, D., J. (2006) Circadian rhythms and reproduction. Reproduction. 132, 379-392.
Brady, A., K., Hilton, J., D. & Vize, P., D. (2009) Coral spawn timing is a direct response to solar light cycles and is not an entrained circadian response. Coral Reefs 28, 277-280.
Brady, A., K., Snyder, K., A. & Vize, P., D. (2011) Circadian Cycles of Gene Expression in the Coral, Acropora millepora. PLoS ONE 6(9): e25072. https://www.doi.org/10.1371/journal.pone.0025072/
Brady, A., K., Willis, B., L., Harder, L., D. & Vize, P., D. (2016) Lunar Phase Modulates Circadian Gene Expression Cycles in the Broadcast Spawning Coral Acropora millepora. Biol. Bull. 230, 130-142.
Butler, M., G., Isogai, S. & Weinstein, B., M. (2009) Lymphatic development. Birth defects research. Part C, Embryo today: reviews. 87(3), 222-231.
Choi, I., Lee, S. & Hong, Y., K. (2012) The new era of the lymphatic system: no longer secondary to the blood vascular system. Cold Spring Harbor perspectives in medicine. 2(4),.
Connelly, M., T., McRae, C., J., Liu, P-J. & Traylor-Knowles, N. (2020) Lipopolysaccharide treatment stimulates Pocillopora coral genotype-specific immune responses but does not alter coral-associated bacteria communities. https://www.sciencedirect.com/science/article/pii/S0145305X20300094
Dardente, H., Wyse, C., A., Birnie, M., J., Dupre, S., M., Loudon, A., S., et al. (2010) A molecular switch for photoperiod responsiveness in mammals. Curr Biol. 20, 21932198.
Dibner, C., Schibler, U. & Albrecht, U. (2010) The mammalian circadian timing system: Organization and coordination of central and peripheral clocks. Annu Rev Physiol. 72, 517-549.
Dolatshad, H., Campbell, E., A., O’Hara, L., Maywood, E., S., Hastings, M., H. et al. (2006) Developmental and reproductive performance in circadian mutant mice. Human Reproduction. 21, 68-79.
Dolatshad, H., Davis, F., C. & Johnson, M., H. (2009) Circadian clock genes in reproductive tissues and the developing conceptus. Reproduction Fertility and Development. 21, 1-9.
Dominoni, D., M. (2015) The effects of light pollution on biological rhythms of birds: an integrated, mechanistic perspective. J. Ornithol. 156, 409-418.
Dunlap, J., C. (1999) Molecular bases for circadian clocks. Cell 96, 271-290.
Fritzenwanker, J, & Technau, U. (2002) Induction of gametogenesis in the basal cnidarian Nematostella vectensis (Anthozoa). Dev Genes Evol. 212, 99-103.
Gotter, A., L., Suppa, C. & Emanuel, B., S. (2007) Mammalian TIMELESS and Tipin are evolutionarily conserved replication fork-associated factors. J Mol Biol. 366, 36-52.
Hardin, P., E. (2009) Molecular mechanisms of circadian timekeeping in Drosophila. Sleep and Biological Rhythms. 7, 235-242.
Harmer, S., L., Panda, S., Kay, S., A. (2001) Molecular bases of circadian rhythms. Annu Rev Cell Dev Biol. 17, 215-253.
Harrison, P., L., Babcock, R., C., Bull, G., D., Oliver, J., K., Wallace, C., C. & Willis, B., L. (1984) Mass spawning in tropical reef corals. Science. 223, 1186-1189.
Hastings, J., W. & Sweeney, B., M. (1958) A persistent diurnal rhythm of luminescence in Gonyaulax polyedra. Biol Bull. 115, 440-458.
Hoadley, K., D., Szmant, A., M. & Pyott, S., J. (2011) Circadian Clock Gene Expression in the Coral Favia fragum over Diel and Lunar Reproductive Cycles. PLoS ONE 6(5), e19755. https://www.doi.org/10.1371/journal.pone.0019755
Hong, Y., Harvey, N., Noh, Y., Schacht, V., Hirakawa, S., Detmar, M. & Oliver, G. (2002) Prox1 is a master control gene in the program specifying lymphatic endothelial cell fate. Developmental Dynamics. 225(3), 351-357.
Houlbrèque, F. & Ferrier‐Pagès, C. (2009) Heterotrophy in Tropical Scleractinian Corals. Biological Reviews. 84(1), 1-17.
Isogai, S., Hitomi, J., Yaniv, K. & Weinstein, B. (2009) Zebrafish as a new animal model to study lymphangiogenesis. Anatomical Science International. 84(3), 102-111.
Johnsen, S., Kelber, A., Warrant, E., Sweeney, A., M., Widder, E., A., Lee, Jr., R., L., J. & Herna´ndez-Andre´s, J. (2006) Crepuscular and nocturnal illumination and its effects on color perception by the nocturnal hawkmoth Deilephila elpenor. J. Exp. Biol. 209, 789-800.
Jokiel, P., L., Ito, R., Y. & Liu, P., M. (1985) Night irradiance and synchronizationof lunar release of planula larvae in the reef coral Pocillopora damicornis. Marine Biology. 88, 167-174.
Joshi, S., Professor of Industrial and Manufacturing Engineering, The Pennsylvania State University, USA.
Koltowska, K., Lagendijk, A., K., Pichol-Thievend, C., Fischer, J., C., Francois, M., Ober, E., A., Yap, A., S. & Hogan, B., M. (2015) Vegfc Regulates Bipotential Precursor Division and Prox1 Expression to Promote Lymphatic Identity in Zebrafish. Cell Reports. 13(9), 1828-1841.
Konopka, R. J. & Benzer, S. (1971) Clock mutants of Drosophila melanogaster. Proc. Natl. Acad. Sci. USA. 68, 2112-2116.
Kuhl, M., Cohen, Y., Dalsgaard, T., Jorgensen, B., B. & Revsbech, N., R. (1995) Microenvironment and photosynthesis of zooxanthellae in scleractinian corals studied with microsensors for 02p, H and light. Mar Ecol Prog Ser. 117, 159–172.
Lang, J. (1973) Interspecific aggression by scleractinian corals. 2. Why the race is not only to the swift. CORAL REEF PROJECT-PAPERS IN MEMORY OF DR. THOMAS F. GOREAU. Bulletin of Marine Science, Miami. 23(2), 260-279.
Lee, C., Bae, K. & Edery, I. (1998) The Drosophila CLOCK protein undergoes daily rhythms in abundance, phosphorylation, and interactions with the PER-TIM complex. Neuron, 21, 857-867.
Levitan, D., R., Fukami, H., Jara, J., Kline, D., McGovern, T., M., McGhee, K., E., Swanson, C., A. & Knowlton, N. (2004) Mechanisms of Reproductive Isolation Among Sympatric Broadcast-Spawning Corals of Montastraea annularis species complex. Evolution. 58, 308-323.
Levitan, D., R., Fogarty, N., D., Jara, J., Lotterhos, K., E. & Knowlton, N. (2011) Genetic, spatial, and temporal components of precise spawning synchrony in reef building corals of the Montastraea annularis species complex. Evolution. 65, 1254-1270.
Levy, O., Appelbaum, L., Leggat, W., Gothlif, Y., Hayward, D., C., Miller, D., J. et al. (2007) Light-Responsive Cryptochromes from a Simple Multicellular Animal, The Coral Acropora Millepora. Science. 318(5849), 467-70.
Levy, O., Kaniewska, P., Alon, S., Eisenberg, E., Karako-Lampert, S. et al. (2011) Complex diel cycles of gene expression in coral-algal symbiosis. Science. 331, 175.
Lexico (2019) Powered by Oxford Dictionary. https://www.lexico.com/en/definition/transcriptome
Lin, C., T. & Todo, T. (2005) The cryptochromes. Genome Biology 6.
Looby, P. & Loudon, A., S., I. (2005) Gene duplication and complex circadian clocks in mammals. Trends in Genetics. 21, 46-53.
Masumoto, K., Ukai-Tadenuma, M., Kasukawa, T., Nagano, M., Uno, K., D. et al. (2010) Acute induction of Eya3 by late-night stimulation triggers TSHb expression in photoperiodism. Curr Biol. 20, 2199-2206.
Meyer, E., Aglyamova, G., V., Wang, S., Buchanan-Carter, J., Abrego, D. et al. (2009) Sequencing and de novo analysis of a coral larval transcriptome using 454 GSFlx. BMC Genomics. 10, 219-236.
Miller, D., J., Hemmrich, G., Ball, E., E. et al. (2007) The innate immune repertoire in Cnidaria – ancestral complexity and stochastic gene loss. Genome Biology. 8, R59.
Muller, W., E., G., Wang, X., Schroder, H., C., Korzhev, M. et al. (2010) A cryptochrome-based photosensory system in the siliceous sponge Suberites domuncula (Demospongiae). FEBS Journal. 277, 1182-1201.
Munn, C., B. (2019) Marine Microbiology: Ecology & Applications, Third Edition. Munn, C., B. (ed.). CRC Press, Taylor & Francis Group, London. pp 273-326.
NCBI (2019) The National Center for Biotechnology Information, Taxonomy Browser. Faviina. https://www.ncbi.nlm.nih.gov/Taxonomy/Browser/wwwtax.cgi?mode=LinkOut&id=123760&lvl=3&p=has_linkout&p=blast_url&p=genome_blast&srchmode=1&keep=1&unlock
Nicenboim, J., Malkinson, G., Lupo, T., Asaf, L., Sela, Y., Mayseless, O., Gibbs-Bar, L., Senderovich, N., Hashimshony, T., Shin, M., Jerafi-Vider, A., Avraham-Davidi, I., Krupalnik, V., Hofi, R., Almog, G., Astin, J., Golani, O., Ben-Dor, S., Crosier, P., Herzog, W., Lawson, N., Hanna, J., Yanai, I. & Yaniv, K. (2015) Lymphatic vessels arise from specialized angioblasts within a venous niche. Nature. 522(7554), 56-61.
Oldach, M., J., Workentine, M., Matz, M., V., Fan, Y-J. & Vize, P., D. (2017) Transcriptome dynamics over a lunar month in a broadcast spawning acroporid coral. Molecular Ecology, 26, 2514-2526.
Penland, L., Kloulechad, J., Idip, D. & van Woesik, R. (2004) Coral spawning in the western Pacific Ocean is related to solar insolation: evidence of multiple spawning events in Palau. Coral Reefs. 23, 133-140.
Reitzel, A., M. & Tarrant, A., M. (2009) Nuclear receptor complement of the cnidarian Nematostella vectensis: phylogenetic relationships and developmental expression patterns. BMC Evol Biol. 9, 230.
Reitzel, A., M., Behrendt, L. & Tarrant, A., M. (2010) Light entrained rhythmic gene expression in the sea anemone Nematostella vectensis: The evolution of the animal circadian clock. PLoS One. 5, e12805.
Rosbash, M. (2009) The implications of multiple circadian clock origins. PLoS Biology, 7, e1000062.
Rubin, E., B., Shemesh, Y., Cohen, M., Elgavish, S., Robertson, H., M, et al. (2006) Molecular and phylogenetic analyses reveal mammalian-like clockwork in the Sea Anemone Circadian Clock honey bee (Apis mellifera) and shed new light on the molecular evolution of the circadian clock. Genome Res. 16, 1352-1365.
Sandrelli, F., Costa, R., Kyriacou, C., P. & Rosato, E. (2008) Comparative analysis of circadian clock genes in insects. Insect Molecular Biology. 17, 447–463.
Sehgal, A., Rothenfluh-Hilfiker, A., Hunter-Ensor, M., Chen, Y., Myers, M., P. et al. (1995) Rhythmic expression of timeless: A basis for promoting circadian cycles in period gene autoregulation. Science. 270, 808-810.
Shinzato, C., Khalturin, K., Inoue, J., Zayasu, Y., Kanda, M., Kawamitsu, M., Yoshioka, Y., Yamashita, H., Suzuki, G. & Satoh, N. (2021) Eighteen Coral Genomes Reveal the Evolutionary Origin of Acropora Strategies to Accommodate Environmental Changes, Molecular Biology and Evolution. 38(1), 16-30.
Shinzato, C., Shoguchi, E., Kawashima, T. et al. (2011) Using the Acropora digitifera genome to understand coral responses to environmental change. Nature. 476, 320-323.
Shlesinger, T. & Loya, Y. (2019) Breakdown in spawning synchrony: A silent threat to coral persistence. Science. 365(6457), 1002-1007.
Shlesinger, Y., Goulet, T. & Loya, Y. (1998) Reproductive patterns of scleractinian corals in the northern Red Sea. Marine Biology. 132, 691-701.
Shoguchi, E., Tanaka, M., Shinzato, C., Kawashima, T. & Satoh, N., A (2013) Genome-Wide Survey of Photoreceptor and Circadian Genes in the Coral, Acropora digitifera. Gene. 515(2), 426-31.
Schuhmacher, H. & Zibrowius, H. (1985) What is hermatypic? Coral Reefs. 4, 1-9.
Simionato, E., Ledent, V., Richards, G., Thomas-Chollier, M., Kerner, P. et al. (2007) Origin and diversification of the basic helix-loop-helix gene family in metazoans: insights from comparative genomics. BMC Evol Biol. 7, 33.
Sorek, M., Díaz-Almeyda, E., M., Medina, M. & Levy, O. (2014) Circadian clocks in symbiotic corals: the duet between Symbiodinium algae and their coral host. Marine genomics. 14, 47-57.
Sorek, M., Yacobi, Y., Z., Roopin, M., Berman-Frank, I. & Levy, O. (2013) Photosynthetic circadian rhythmicity patterns of Symbiodinium, the coral endosymbiotic algae. Proceedings. Biological sciences. 280(1759), 20122942.
Stryer, L. (1995) Biochemistry 4th Edition. Stryer, L. (ed.). W. H. Freeman and Company, New York. pp 721-722.
Stryer, L. (1995a) Biochemistry 4th Edition. Stryer, L. (ed.). W. H. Freeman and Company, New York. p 1004.
Sullivan, J., C., Wolenski, F., S., Reitzel, A., M., French, C., E., Traylor-Knowles, N. et al. (2009) Two alleles of NF-kB in the sea anemone Nematostella vectensis are widely dispersed in nature and encode proteins with distinct activities. PLoS ONE. 4, e7311.
Szmant-Froelich, A., Reutter, M. & Riggs, L. (1985) Sexual reproduction of Favia fragum (Esper): lunar patterns of gametogenesis, embryogenesis and planulation in Puerto-Rico. Bulletin of Marine Science. 37, 880-892.
Tamai, S., Sanada, K. & Fukada, Y. (2008) Time-of-day-dependent enhancement of adult neurogenesis in the hippocampus. PLoS One. 3, e3835.
Taylor, W., C. (1989) Transcriptional regulation by a circadian rhythm. Plant Cell. 1, 259-264.
Thompson, J., Rivera, H., Medina, M. & Closek, C. (2015) Microbes in the coral holobiont: Partners through evolution, development, and ecological interactions. Frontiers in Cellular and Infection Microbiology. 4,.
Toyama, R., Chen, X., Jhawar, N., Aamar, E., Epstein, J. et al. (2009) Transcriptome analysis of the zebrafish pineal gland. Dev Dyn. 238, 1813-1826.
Urban, A., Zhou, X., Ungoss, J., Raible, D., W., Altmann, C., R. et al. (2006) FGF is essential for both condensation and mesenchymal-epithelial transition stages of pronephric kidney tubule development. Dev Biol. 297, 103-117.
Van Gelder, R., N. (2002) Tales from the crypt(ochromes). J Biol Rhythms. 17, 110-120.
van Woesik, R., Lacharmoise, F. & Koksal, S. (2006) Annual cycles of solar insolation predict spawning times of Caribbean corals. Ecol. Lett. 9, 390-398.
Vize, P., D. (2009) Transcriptome analysis of the circadian regulatory network in the coral Acropora millepora. Biol Bull. 216(2), 131-137.
Vize, P. D., Embesi, J., A., Nickell, M., Brown, D., P & Hagman, D., K. (2005) Tight temporal consistency of coral mass spawning at the Flower Garden Banks, Gulf of Mexico, from 1997-2003. Gulf Mex. Sci. 23, 107-114.
Wallace, C., C., Babcock, R., C., Harrison, P., L., Oliver, J., K. & Willis, B., L. (1986) Sex on the reef: mass spawning of corals. Oceanus. 29, 38-42.
Wallace, R., A., Sanders, G., P. & Ferl, R., J. (1996) Biology: The Science of Life, Fourth Edition, HaperCollins Publishers Inc. New York, USA. G-5; p 782.
Wallace, R., A., Sanders, G., P. & Ferl, R., J. (1996a) Biology: The Science of Life, Fourth Edition, HaperCollins Publishers Inc. New York, USA. G-22; pp 384-385.
Ying, H., Cooke, I., Sprungala, S., Wang, W., Hayward, D., C., Tang, Y.; Huttley, G., Ball, E., E., Forêt, S. & Miller, D., J. (2018) Comparative genomics reveals the distinct evolutionary trajectories of the robust and complex coral lineages. Genome Biology. 19, 175. https://doi.org/10.1186/s13059-018-1552-8
Yuan, Q., Metterville, D., Briscoe, A., D. & Reppert, S., M. (2007) Insect cryptochromes: gene duplication and loss define diverse ways to construct insect circadian clocks. Mol Biol Evol. 24, 948-955.
0 Comments