Last time we commenced to explore the significance and upkeep of the rarer trace elements of seawater in recirculating systems because ionic speciation is different from the wild. Herein we continue opting for the safest and most natural replenishment strategies, while we strive to keep the terminology within the realm of the hobbyist without impinging on the author’s style.
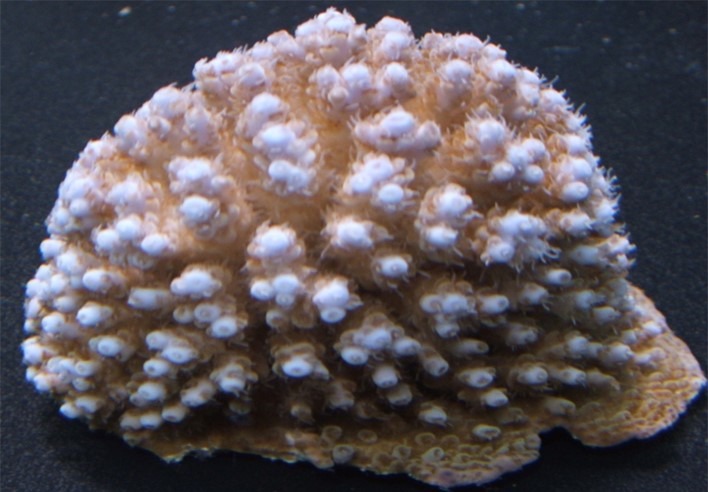
Fig 1. Sky blue Acropora cf. verweyi featuring a wave action-resilient, light harvesting-ineffectual, branching configuration. Photograph courtesy of the Reef RanchTM ©. The colony was perhaps acquired from the crest of an eastward reef front. Branching corals with diminished surface area to volume ratios were indigenous inshore and offshore yet were lacking mid-shelf (Doszpot et al. 2019).
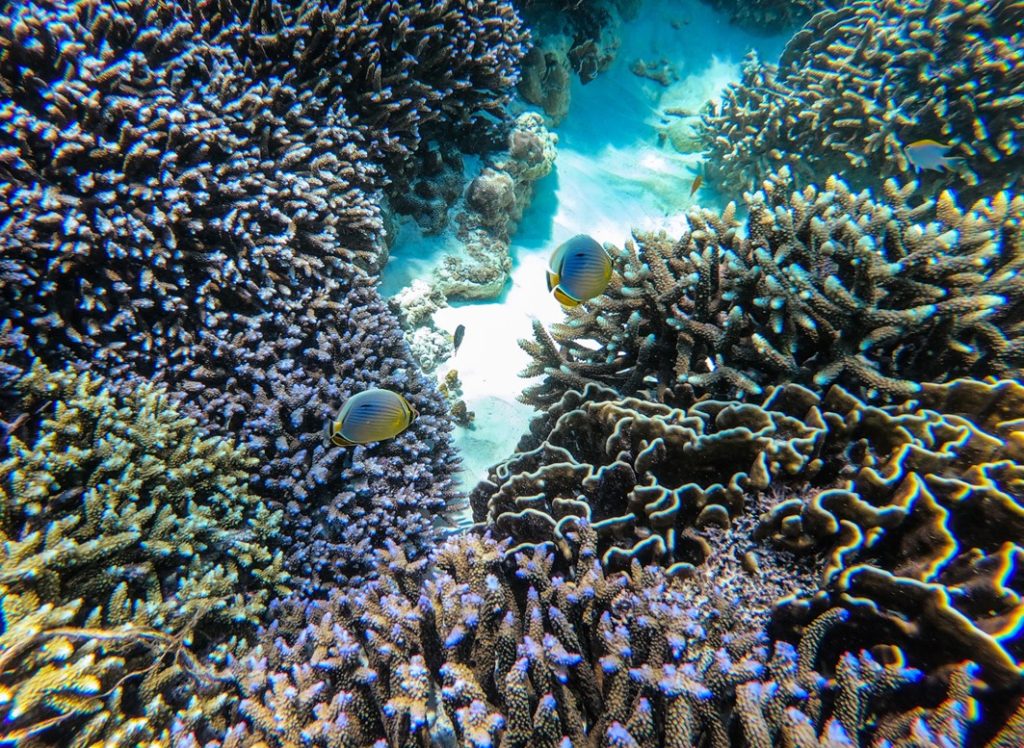
Fig 2. Obligate corallivore blacktail butterflyfish (Chaetodon cf. austriacus) “nibbling” at wild SPS colonies flanking a substrate channel in a pristine Red Sea environment (Adams 2018a).
The halogens appear in group 17 of the periodic table which only need one electron to fill their orbitals and attain the configuration of a highly unreactive and grounded noble gas. They thus oxidize other atoms by capturing one of their electrons, however, the autoionization catalyst water reduces these elements to noble gas-like solubilized negatively charged anions while it oxidizes group 1 and 2 sodium, potassium, calcium, and magnesium to the noble gas-like cations Na+, K+, Ca2+, and Mg2+. The foremost and pertinent halogens of seawater are chlorine (Cl), bromine (Br), and iodine (I) which tend to manifest as chloride (Cl–), bromide (Br–), iodide (I–), and iodate (IO3–). Nevertheless, their unstable nature is somewhat retained in their ionic form, inasmuch as they are the first to react when ozone is applied to unreduced seawater. Such total residual oxidants (TROs) can also arise when iodine is supplemented or up to 36 hours after a water change in ozone-utilizing systems (Wilson, personal communication; Latson et al. 2018).
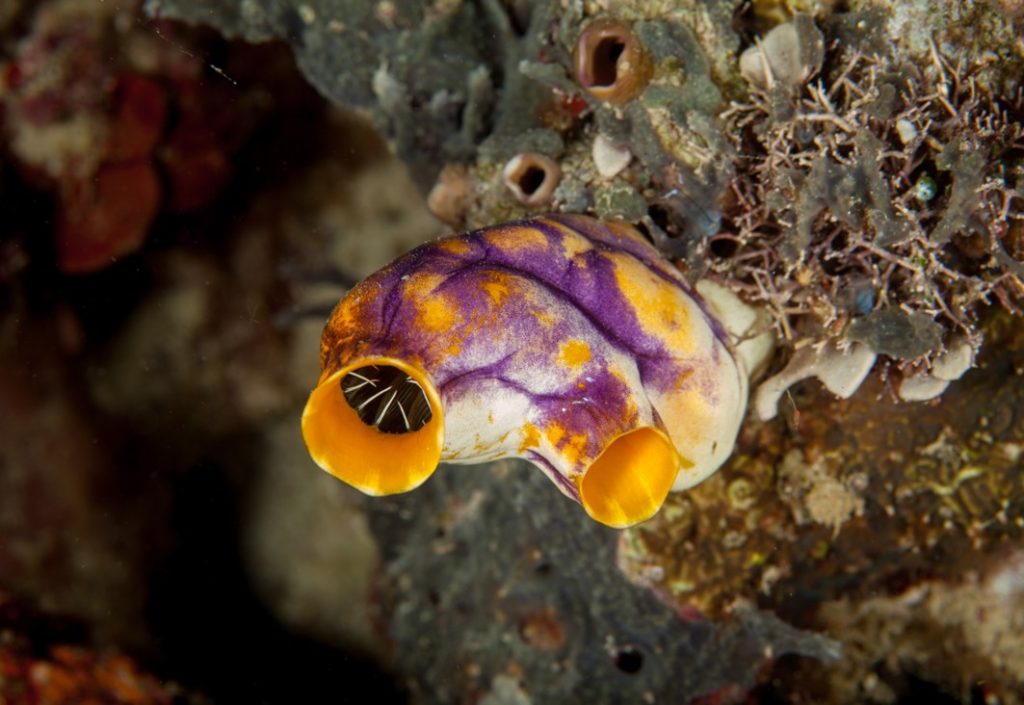
Fig 3. A tunicate or sea squirt (Chordata: Ascidiacea).
Chloride (Cl–) is the most abundant and rapidly moving anion and potent oxidizing-agent that disrupts ionic bonds and readily captures electrons from iodide (I–) and bromide (Br–; Balling et al. 2008).
Iodine is the least abundant and the most readily expended of the three primary halogens in recirculating systems while its gaseous form occurs in air (Dembitsky 2006). “Algae” including zooxanthellae may store iodine as iodate (IO3–) and reduce it to iodide (I–) when required, while it may provide photosynthetic overload protection and thus its dearth may expedite bleaching (Balling et al. 2008), whereas bromine performs a similar role in the kelp Laminaria digitata (Kupper et al. 2013).
Nori seaweed used to wrap sushi comprises several macro- and pico-minerals in comparable ratios to those of seawater, while iodine is significantly enriched. It is a useful supplement for angelfish, tangs, surgeonfish, and hermit crabs; however, exercise caution because it comprises much phosphorus. See: Part IV.
Numerous marine organisms amass iodine to deter predation like sea squirts of the phylum Chordata and subphylum Tunicata, while it remains a prominent constituent of shrimp and worm integuments that may be necessary for the coloration of blue species of Acropora (Fig 1.; Fig 3.; Holmes-Farley 2003).
Oceanic iodine ranges from 0.04 to 0.06 mg l-1 (ppm) of iodate (IO3–) and 0.01 to 0.02 mg l-1 iodide (I–) where its full speciation remains enigmatic, albeit anaerobic bacteria and phytoplankton take up iodate (IO3–) and liberate iodide (I–) which algae preferentially absorb and utilize (Holmes-Farley 2003).
Methyl iodide (CH3I; iodomethane) is the simplest form of organoiodine, whereas the meta- and ana-bolism of several marine organisms manufacture complex kinds. Ultraviolet (UV) sterilizers and ozone oxidize iodide (I–) to iodate (IO3–; Holmes-Farley 2003) which is one of the few reasons to exploit UV sterilization.
Macroalgal reactive oxygen species (ROS) may provoke organoiodine synthesis, while their peroxidases may form the by-products diiodomethane (CH2I2), methyl iodide (CH3I), and iodobutane (C4H9I; Collén et al. 1994; Laturnus 2008) where mono-carbon tri-halogenated hydrocarbons like bromodichloromethane (CHBrCI2) and dibromochloromethane (CHBr2CI) remain rare oddments (Laturnus 2008).
Toxicity
Lugol’s iodine and tinctures contain molecular iodine (I2) which is sterilizing and potentially harmful yet ionic forms are non-biocidal (Holmes-Farley 2003). Coral dips of diluted Lugol’s iodine may partially sterilize the mucosal microbiome which is the colony’s first line of defense (Pantos et al. 2003; Garber 2011; Ho 2013; Soffer et al. 2013; Rivera-Ortega & Thomé 2018; Santoro et al. 2021) which may render corals susceptible to microbial attack (Oakey & Owens 2000; Oakey et al. 2002; Lesser et al. 2007; Hall et al. 2018; Morrow et al. 2018).
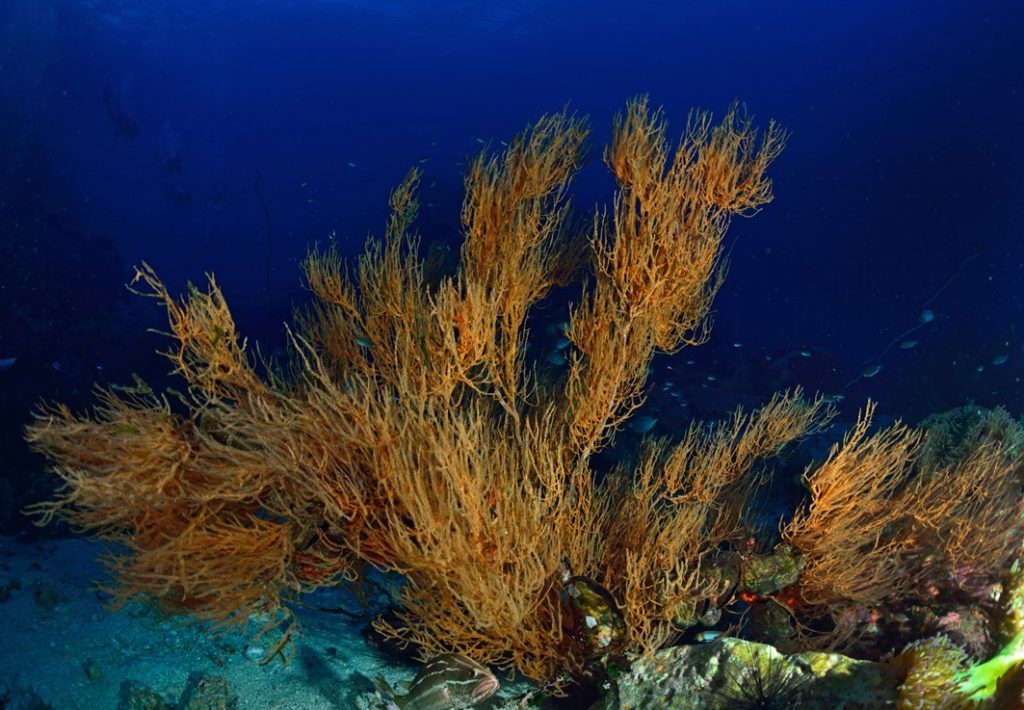
Fig 4. Black coral, Antipathes cf. grandis (Hexacorallia: Antipatharia).
Molecular iodine (I2) commences to poison teleosts at <1 mg l-1 (ppm) while I2 and I– prove toxic to some invertebrates at nominal concentrations, but only when the latter approaches twenty times that of natural seawater. Culturists of macroalgae will observe enhanced growth during I– and IO3– enrichment which appears the most benign, whereas the former inhibits growth of Caulerpa species at ~13 mg l-1 (Holmes-Farley 2003).
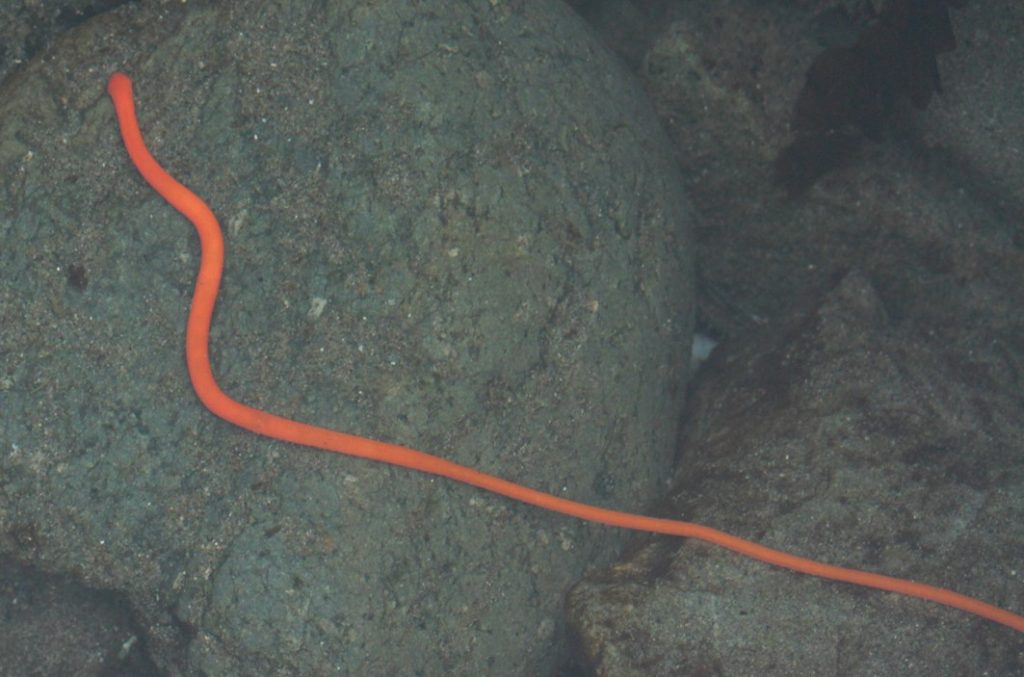
Fig 5. A relative of the rare and elusive ribbon worm, Lineus ruber which reach merely 6 to 7 cm (2.4 to 2.8”).
Mammalian biphenyl thyroxine (C15H11I4NO4) is manufactured in the thyroid gland yet it remains a constituent of the supportive spicules of sea fans/sea whips (Octocorallia: Alcyonacea) while its synthesis demands UV radiation (Kingsley et al. 2001). Tunicates manufacture thyroxine and the cytotoxin lukianol B (C25H16INO5) from dissolved inorganic iodine (DIi; Fig 3.; Fig 4.; Goldberg 1978; Yoshida et al. 1992; Goldberg et al. 1994; Holmes-Farley 2003). Marine worms use several toxic compounds to discourage predation (Göransson et al. 2019), while the marine ribbon worm (Lineus ruber) produces pharyngeal thyroid-like hormones and thus was a likely participant in thyroid gland development (Fig 5.; Balfour & Willmer 1967).
- Fig 6. Left – toadstool coral (Sarcophyton species). Right – a colony of pulse xenia, Xenia umbellata.
The bases of antipatharian black corals amass up to 23 percent iodine, while organoiodine may render macroalgal flesh unpalatable (Fig 4.; Holmes-Farley 2003).
Toxigenesis in Geodia species of leathery barrel sponge yields geodiamolide A and B which consist of a tripeptide, iodine,
and bromine (Chan et al. 1987). Iodine represents ~1 percent of dry sponge weight which predators may find foul-tasting (Solimabi et al. 1981), whereas sponges either absorb DIi or acquire dietary organoiodine (Holmes-Farley 2003).
Shrimp anabolism is stimulated by organoiodine-rich diets (Fa-yi et al. 1995) because iodine is assimilated systemically including their shell (Holmes-Farley 2003; Bottaro et al. 2007) while the tissues of Xenia and Sarcophyton species contain ~100 mg kg-1 (ppm; Fig 6.; Shimek 2002).
Reef salts may be a significant source so test everything, while much may be sustained by the ingestion and biomineralization of feeds. Iodine has proven toxic at ionic strengths that barely exceed those of seawater, and several manifestations are unlikely to appear in the results of aquarium assays. It is normally ill-advised to supplement an ion without first ascertaining its dilution and the author advises extreme moderation.
Supplements are usually formulated from dilute solutions of potassium iodide (KI) which may be cycled in our reefs to iodate (IO3–) using UV sterilization but ozone must cease for up to 24 hours post-administration. The author has described a DIY iodine supplement for completeness however…
As with any potentially dangerous procedure, the reader prepares and uses these DIY supplements at their own risk, because the author, Reef Ranch Publishing Ltd, websites hosting this article, and their owners cannot be held responsible for any harm to pets, people, possessions, or properties. Personal protective equipment (PPE) is advised, and immediately cleanse the compound from skin. See: Part VI for stock solution and PPE guidance.
Thoroughly dissolve 50 g of pharmaceutical or food grade anhydrous potassium iodide (KI) in 100 ml (100 g) of R/O water to create a stock concentrate from which 1-ml is added to 199 ml of R/O water to make up the 1.93 g l-1 iodide supplement. Botulinum cook as described in Part VI, clearly label, and store in a dark refrigerator out of reach of children and pets.
Administering four drops (0.39 mg iodide) of the supplement for every 30 liters of system volume creates a systemic fortification of 1.3 x 10-2 mg l-1 wk-1 of iodide, which is appropriate for merely fully stocked aquaria exploiting a UV sterilizer with ample coralline and macroalgae. Remarkably KI increases the efficacy of UV sterilization which appears otherwise non-bactericidal (Huang et al. 2017; Vieira et al. 2018).
Join us next time when we shine a spotlight on strontium, boron, and aluminum which are more than adequately maintained by nominal husbandry.
To learn more, visit Reef Ranch Publishing
References
Adams, J. (2018a) Interesting Ecology Shift of Blacktail and Orangeface Butterflyfish. ReefBuilders.com. https://reefbuilders.com/2018/12/18/blacktail-and-redface-butterflyfish/
Balfour, W., E. & Willmer, E., N. (1967) Iodine Accumulation in a Nemertine,Lineus Ruber. Journal of Experimental Biology. 46(3), 551-556.
Balling, H., W., Janse, M. & Sondervan, P. (2008) Trace elements, functions, sinks and replenishment in reef aquaria. Advances in coral husbandry in public aquariums. Leewis, R., J. & Janse, M. (eds.). Burgers’ Zoo, Arnhem, The Netherlands. pp 143-156.
Bottaro, C., Kiceniuk, J. & Chatt, A. (2007) Spatial distribution of extractable organohalogens in northern pink shrimp in the North Atlantic. Biological Trace Element Research. 72(1), 149-166.
Chan, W., Tinto, W., Manchand, P. & Todaro, L. (1987) ChemInform Abstract: Stereostructures of Geodiamolides A and B, Novel Cyclodepsipeptides from the Marine Sponge Geodia sp. ChemInform. 18(51), 3091-3093.
Collén, J., Ekdahl, A., Abrahamsson, K. & Pedersén, M. (1994) The involvement of hydrogen peroxide in the production of volatile halogenated compounds by Meristiella gelidium. Phytochemistry. 36(5), 1197-1202.
Dembitsky, V. (2006) Biogenic Iodine and Iodine-Containing Metabolites. Natural product communications. 1(2),139-175.
Doszpot, N., E., McWilliam, M., Pratchett, M., Hoey, A. & Figueira, W. (2019) Plasticity in Three-Dimensional Geometry of Branching Corals Along a Cross-Shelf Gradient. Diversity. 11, 44.
Fa-yi, L., He-fang, L., Hui-liang, W., Liang De-hai, L. & Yu-chuan, T. (1995) Requirements of shrimp, Penaeus chinensis O’sbeck for potassium, sodium, magnesium and iodine. J. Ocean. Limnol. 13(2), 141-146.
Garber, S. (2011) Fighting Fire with Fire: Saving Corals from Bacteria with Bacteria. AdvancedAquarist.com. https://www.advancedaquarist.com/blog/fighting-fire-with-fire-saving-corals-from-bacteria-with-bacteria
Garland, J., A. & Curtis, H. (1981) Emission of iodine from the sea surface in the presence of ozone. J. Geophys. Res. 86(C4), 3183-3186.
Goldberg, W. (1978) Chemical changes accompanying maturation of the connective tissue skeletons of gorgonian and Antipatharian corals. Marine Biology. 49(3), 203-210.
Goldberg, W., M., Hopkins, T., L., Holl, S., M., Schaefer, J., Kramer, K., J., Morgan, T., D. & Kim, K. (1994) Chemical composition of the sclerotized black coral skeleton (Coelenterata: Antipatharia): a comparison of two species. Comparative Biochemistry and Physiology Part B: Comparative Biochemistry. 107(4), 633-643.
Göransson, U., Jacobsson, E., Strand, M. & Andersson, H., S. (2019) The Toxins of Nemertean Worms. Toxins. 11, 120.
Hall, E., R., Muller, E., M., Goulet, T., Bellworthy, J., Ritchie, K., B. & Fine, M. (2018) Eutrophication may compromise the resilience of the Red Sea coral Stylophora pistillata to global change. Marine Pollution Bulletin. 131, 701-711.
Ho, L. (2013) A newly discovered bacterial denitrification symbiosis. AdvancedAquarist.com. https://www.advancedaquarist.com/blog/a-newly-discovered-bacterial-denitrification-symbiosis
Holmes-Farley, R. (2003) Chemistry and The Aquarium: Iodine in Marine Aquaria: Part I. AdvancesAquarist.com. https://www.advancedaquarist.com/2003/3/chemistry
Huang, L., Szewczyk, G., Sarna, T. & Hamblin, M., R. (2017) Potassium Iodide Potentiates Broad-Spectrum Antimicrobial Photodynamic Inactivation Using Photofrin. ACS infectious diseases. 3(4), 320-328.
Küpper, F., C., Carpenter, L., J., Leblanc, C., Toyama, C., Uchida, Y., Maskrey, B., H., Robinson, J., Verhaeghe, E., F., Malin, G., Luther, G., W., Kroneck, P., M., H., Kloareg, B., Meyer-Klaucke, W., Muramatsu, Y., Megson, I., L., Potin, P., Martin C. & Feiters, M., C. (2013) in vivo speciation studies and antioxidant properties of bromine in Laminaria digitatareinforce the significance of iodine accumulation for kelps. Journal of Experimental Botany. 64(10), 2653-2664.
Kupper, F., C., Kloareg, B., Guern, J. & Potin, P. (2001) Oligoguluronates elicit an oxidative burst in the brown algal kelp Laminaria digitata. Plant physiology. 125(1), 278-291.
Latson, E., F., George, R., LePage, V., Neal, L. & Sowers, K. (2018) Treatment of a Large Multi-species Display Exhibit with Chloroquine and Mortality Associated with Treating the Water Containing Chloroquine with Ozone. IAAAM. https://www.vin.com/apputil/content/defaultadv1.aspx?pId=20778&catId=113378&id=8505022
Laturnus, F. (2008) Marine macroalgae in polar regions as natural sources for volatile organohalogens. Environmental Science and Pollution Research. 8(2), 103-108.
Lesser, M., P., Falcón, L., I., Rodríguez-Román, A., Enríquez, S., Hoegh-Guldberg, O. & Iglesias-Prieto, R. (2007) Nitrogen fixation by symbiotic cyanobacteria provides a source of nitrogen for the scleractinian coral Montastraea cavernosa. Mar Ecol Prog Ser. 346, 143-152.
Morrow, K., M., Muller E. & Lesser M., P. (2018) How Does the Coral Microbiome Cause, Respond to, or Modulate the Bleaching Process? Coral Bleaching. Ecological Studies (Analysis and Synthesis). 233. van Oppen, M. & Lough, J. (eds.). Springer, Cham. pp 153-188.
Oakey, H., Cullen, B. & Owens, L. (2002) The complete nucleotide sequence of the Vibrio harveyibacteriophage VHML. Journal of Applied Microbiology, 93,1089-1098.
Oakey, H., J. & Owens, L. (2000) A new bacteriophage, VHML, isolated from a toxin-producing strain of Vibrio harveyi in tropical Australia. Journal of Applied Microbiology. 89, 702-709.
Pantos, O., Cooney, R., Le Tissier, M., Barer, M., O’Donnell, A. & Bythell, J. (2003). The bacterial ecology of a plague‐like disease affecting the Caribbean coral Montastrea annularis. Environmental Microbiology. 5(5), 370-382.
Rivera-Ortega, J. & Thomé, P., E. (2018) Contrasting Antibacterial Capabilities of the Surface Mucus Layer From Three Symbiotic Cnidarians. Frontiers in Marine Science. 5, 392.
Santoro, E., P., Borges, R., M., Espinoza, J., L., Freire, M., Messias, C., S., M., A., Villela, H., D., M., Pereira, L., M., Vilela, C., L., S., Rosado, J., G., Cardoso, P., M., Rosado, P., M., Assis, J., M., Duarte, G., A., S., Perna, G., Rosado, A., S., Macrae, A., Dupont, C., L., Nelson, K., E., Sweet, M., J., Voolstra, C., R. & Peixoto, R., S. (2021) Coral microbiome manipulation elicits metabolic and genetic restructuring to mitigate heat stress and evade mortality. Science advances. 7(33), 3088.
Shimek, R., L. (2002) It’s (In) The Water. Reefkeeping.com http://reefkeeping.com/issues/2002-02/rs/feature/index.htm
Soffer, N., Brandt, M., Correa, A., M., S., Smith, T., B. & Thurber, R., V. (2013) Potential role of viruses in white plague coral disease. The Isme Journal. 8, 271-283.
Solimabi, B., D., Mittal, P., K. & Kamat, S., Y. (1981) Bromine and iodine content in sponges and algae of the Andaman Sea. Natl. Inst. Oceanogr., Goa, India. Indian Journal of Marine Sciences. 10(3), 301-302.
Vieira, C., Gomes, A., Mesquita, M., Moura, N., Neves, M., Faustino, M. & Almeida, A. (2018) An Insight Into the Potentiation Effect of Potassium Iodide on aPDT Efficacy. Frontiers in Microbiology. 9,.
Wilson, M. (2008) World Feeds Limited, 3b Coulman Street Industrial Estate, Thorne, Doncaster, DN8 5JS, United Kingdom.
Yoshida, W., Lee, K., Carroll, A. & Scheuer, P. (1992) A Complex Pyrrolo‐oxazinone and Its Iodo Derivative Isolated from a Tunicate. Helvetica Chimica Acta. 75(5), 1721-1725.
0 Comments