Before I go into the relationship between amino acids and corals, I would first like to address the following question: what are amino acids? Basically, amino acids belong to a class of organic compounds that is essential to all life. Amino acids form the building blocks of proteins, which in turn have essential functions in living organisms. Twenty two different amino acids exist, but only 21 are found in eukaryotes, i.e. animals, plants, fungi and protists. Although bacteria, plants, and fungi can synthesize most amino acids, animals cannot produce several of them in sufficient quantities to meet their metabolic needs (Shinzato et al. 2014). For example, humans can synthesize 11 amino acids in sufficient quantities, whereas the other 9 must be acquired through the diet. These 9 amino acids are therefore known as essential amino acids, and include histidine, isoleucine, leucine, lysine, methionine, phenylalanine, threonine, tryptophan and valine.
Essential | Non-essential |
---|---|
Histidine | Alanine |
Isoleucine | Arginine* |
Leucine | Asparagine |
Lysine | Aspartic acid (Aspartate) |
Methionine | Cysteine* |
Phenylalanine | Glutamic acid (Glutamate) |
Threonine | Glutamine* |
Tryptophan | Glycine |
Valine | Ornithine* |
Proline* | |
Selenocysteine* | |
Serine* | |
Tyrosine* | |
Pyrrolysine** | |
*Essential only in certain cases (Reeds 2000; Fürst and Stehle 2004), **Found only in certain bacteria and archaea (Srinivasan et al. 2002). |
Although each amino acid is chemically different, they all have the same basic structure. On one side of the molecule, they have what is called an amine group (NH2), hence their name. On the other side, we find a carboxyl group (COOH), which gives amino acids their acidic properties. The amine and carboxyl groups are fused with a carbon atom, together with a variable side chain (R) that is different for each amino acid. The common chemical formula for amino acids is R-CH(NH2)-COOH.
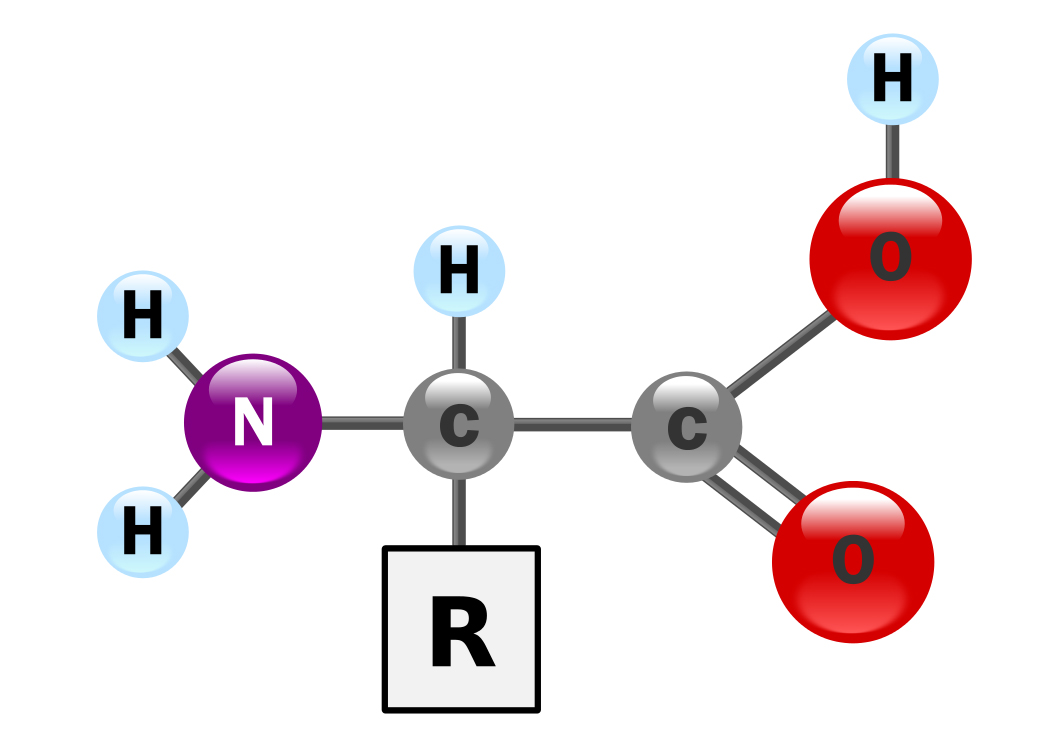
Basic structure of an alpha amino acid, with the amine group (NH2) shown on the left, and the acidic carboxyl group (COOH) on the right. The R indicates a side chain which varies between different amino acids (image: Y. Mrabet).
In living cells, amino acids can be linked together to form proteins, a process known as protein biosynthesis (also called biogenesis or anabolism). This is done by linking the amine and carboxyl groups of two amino acids together, where a hydrogen atom (H+) is split from the amine group, and a hydroxyl group (OH–) is removed from the carboxyl group. Thus, this reaction generates water (H2O). Protein biosynthesis occurs when cells translate genetic information into proteins, reading the genetic code stored in DNA letter by letter (or actually, three letters at a time), where each three-letter combination corresponds to a specific amino acid. When several amino acids are linked together in a living cell, this larger molecule is called a peptide. Proteins are larger versions of peptides, in which hundreds of amino acids are linked together. After this linkage, a protein has to be chemically modified and folded into complex shapes to allow it to function inside or outside the cell, as a structural part of a cell membrane, a hormone, an antibody or an enzyme.
Sources of amino acids, with a focus on corals
Now that we know that amino acids are essential components of living systems, the next question is how organisms obtain these. As mentioned above, a source of amino acids is biosynthesis. In addition, amino acids can be acquired from the external environment. For the rest of this article, I will focus on stony, or scleractinian corals (order Scleractinia).
Biosynthesis
Just like proteins can be produced from individual amino acids, so too can amino acids themselves be synthesized by living organisms. The building blocks, or substrates, for amino acid biosynthesis can be other acids (such as 3-phosphoglycerate and oxaloacetate) or amino acids themselves. Microorganisms lie at the basis of this all, as they can use inorganic nitrogen in the form of nitrogen gas (N2) to produce ammonia/ammonium (NH3/NH4+), the basic nitrogen ingredients for global amino acid synthesis. Ammonia and ammonium, in turn, are converted into organic form by higher organisms as the amino acids glutamate and glutamine. Examples of such higher organisms are plants, dinoflagellates including zooxanthellae, and possibly also Aiptasia spp., tropical anemones that are considered pests in marine aquaria (also see Wijgerde 2012).
Corals can also produce their own amino acids, and unlike most animals, at least some corals can synthesize the essential ones. The ability to produce (essential) amino acids seems to vary between coral species, however. Fitzgerald and Szmant (1997) conducted tracer experiments with radioactive glucose, and found that five different coral species (Montastraea faveolata, Acropora cervicornis, Porites divaricata, Tubastraea coccinea and Astrangia poculata) were all able to synthesize at least 15 different amino acids, of which 8 essential ones. However, analysis of all the Acropora digitifera genes-the so-called genome-revealed that this species can only synthesize ten non-essential amino acids except cysteine, and not the essential ones (Shinzato et al. 2011). Porites australiensis, in turn, does seem to produce cysteine (Shinzato et al. 2014). The discrepancy between these studies could be explained by the different species used, which may possess different synthesis capabilities. However, Fitzgerald and Szmant (1997) acknowledge that the essential amino acids they found may be partially the result of biosynthesis by bacteria residing in the coelenteron (also known as the coelenteric cavity, gastrovascular cavity, or stomach)of the coral polyps. They ruled out that biosynthesis of the symbiotic zooxanthellae was responsible for the findings, as the pattern of radioactive amino acids detected was similar between the zooxanthellate (M. faveolata, A. cervicornis, P. divaricata) and azooxanthellate species (T. coccinea and A. poculata).
What makes corals interesting and complicating animals is that they host symbiotic zooxanthellae, dinoflagellates with the ability to produce at least 14 amino acids, of which 7 are essential (Shinzato et al. 2014). The capability of coral-associated zooxanthellae to produce lysine and methionine is unclear, as some of the required enzyme-encoding genes were not found in their genome (Shinzato et al. 2014), although lysine synthesis was confirmed for the zooxanthellae of Aiptasia pulchella (Wang and Douglas 1999). Methionine may be produced by a combination of zooxanthellae and coral enzymes, which suggests that both symbiont partners have evolved to complement one another (Shinzato et al. 2014). Von Holt (1969) suggested something similar, as the zoanthid Zoanthus flos marinus (today known as Zoanthus sociatus) can produce glycine, unlike its zooxanthellae, possibly making the latter dependent on the zoanthid.
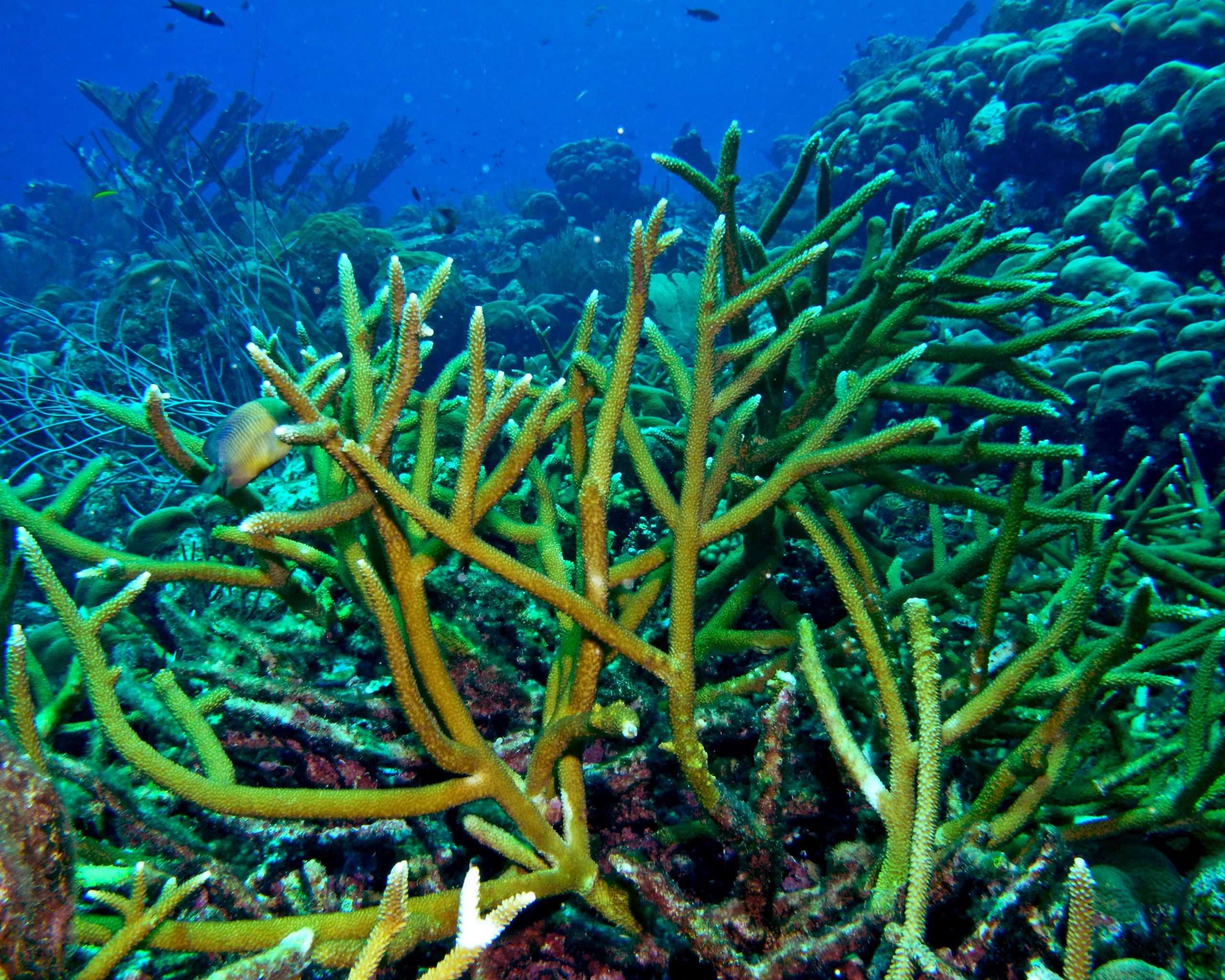
Together, corals and their associated zooxanthellae can produce many amino acids (Acropora cervicornis in the waters off Curaçao, Dutch Caribbean. Image: Benjamin Mueller, Royal Netherlands Institute for Sea Research).
Tracer experiments with radioactive sodium carbonate (Na214CO3) have shown that zooxanthellae also transfer amino acids, or precursors thereof (glycoconjugates), to their host animal (von Holt and von Holt 1968; Markell and Trench 1993; Wang and Douglas 1999). Zooxanthellae convert the radioactive carbonate to amino acids via photosynthesis, after which the 14C-labeled amino acids are translocated to the host coral. This matches with genetic insights, which reveal that zooxanthellae have the necessary genes to produce transporter proteins for translocating amino acids to their coral host (Shinzato et al. 2014). All of this suggests that corals can acquire most, if not all amino acids via biosynthesis, either directly or via their symbiotic zooxanthellae and bacteria. It is also hypothesized that when corals feed on plankton, they gain additional nitrogen which allows the zooxanthellae to produce and translocate more amino acids to their coral host (Swanson and Hoegh-Guldberg 1998; Wang and Douglas 1999).
Uptake from the external environment
Next to producing amino acids via biosynthesis, corals can take up a range of amino acids from the seawater (Goreau 1971; Ferrier 1991; Fitzgerald and Szmant 1997; Grover et al. 2008). These include, but may not be limited to, histidine, leucine, methionine, valine, alanine, asparagine, aspartic acid, glutamic acid, glutamine, glycine and serine (Grover et al. 2008). Although the amino acid concentration of reef waters is very low (0.2-0.5 µmol L-1), corals have very efficient transport mechanisms to deal with this (Goreau et al. 1971; Grover et al. 2008). Transport proteins, also called carriers, present in the cell membranes of coral tissue (and all living organisms for that matter) allows corals to take up these scarcely available compounds.
It has been found that amino acid uptake can be a significant source of nitrogen. For Stylophora pistillata, amino acids can account for 21% of the nitrogen uptake (Grover et al. 2008), although this will vary under different environmental conditions, i.e. the concentrations of ammonium, nitrate, urea and amino acids, and plankton abundance. For example, Grover et al. (2008) found that the amino acid uptake rate by S. pistillata increases 6-fold when the total amino acid concentration is artificially increased to 8 µmol L-1. For the corals S. pistillata and Galaxea fascicularis, and the zoanthid Zoanthus sociatus, amino acid uptake and incorporation into protein is higher in light than in darkness, whereas the opposite is true for Pocillopora damicornis (von Holt 1969; Al-Moghrabi et al. 1993; Hoegh-Guldberg and Williamson 1999; Grover et al. 2008). This suggests that photosynthesis plays a role in some species, possibly enhancing protein synthesis by the animal. This mechanism has been called light-enhanced amino acid assimilation (Al-Moghrabi et al. 1993).
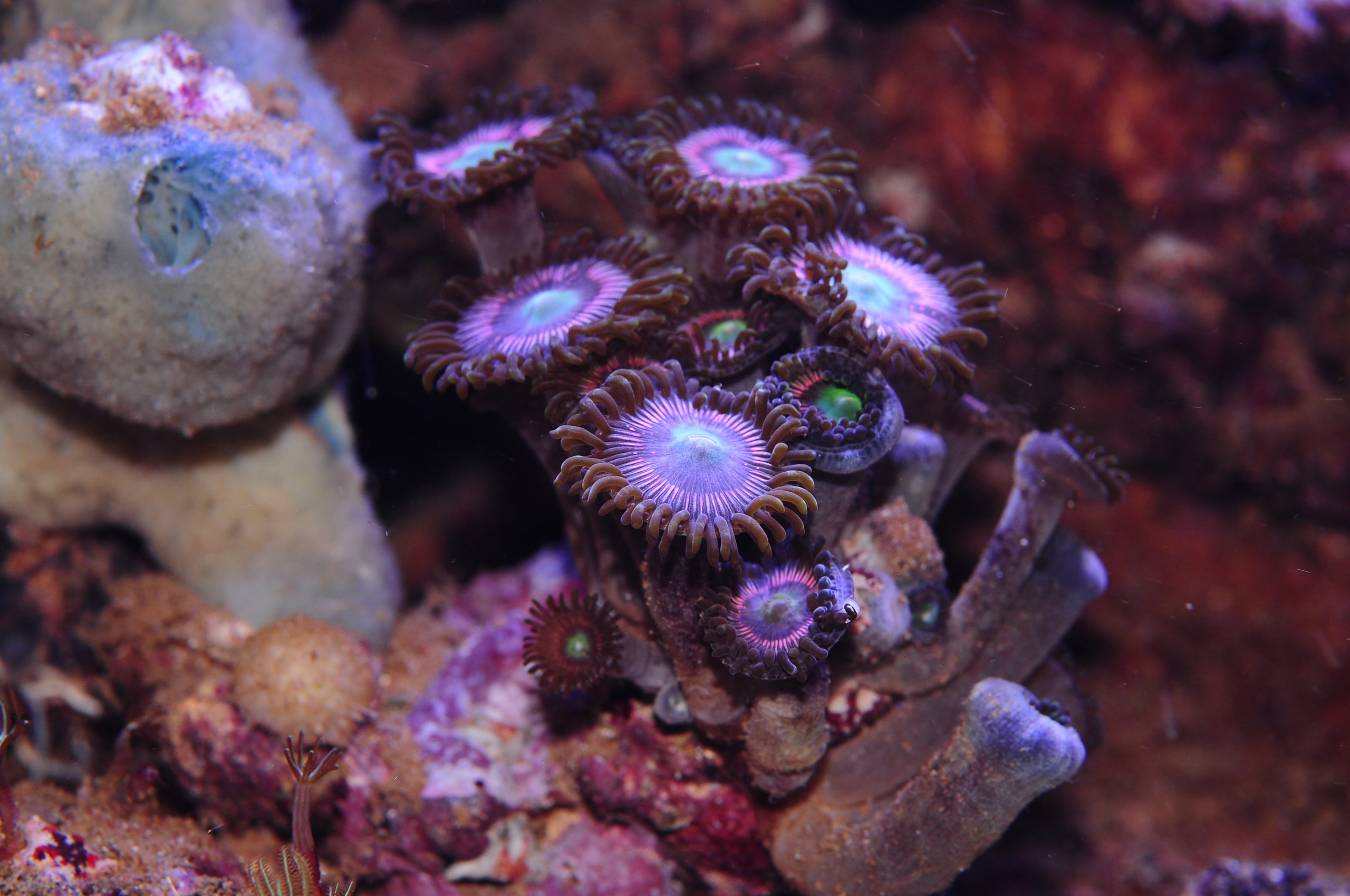
Next to biosynthesis, corals and zoanthids can acquire amino acids from the seawater, in dissolved and particulate form (image: T. Wijgerde).
Normalized to biomass, amino acid uptake can be 5-7 times higher in the zooxanthellae compared to the host coral (Grover et al. 2008), although it is unclear why, as zooxanthellae are able to synthesize most amino acids themselves. The uptake by zooxanthellae is rapid, and occurs within two hours, possibly first via the seawater that fills the coelenteric cavity of coral polyps. This is plausible, as the zooxanthellae reside in the gastroderm which lines this cavity.
Plankton also is an important source of amino acids, as bacteria, phytoplankton and zooplankton are all rich in protein. When corals feed on plankton, they can break down its proteins via digestion and liberate the amino acids. This digestive process is known as protein hydrolysis (breakdown of proteins using water), and is facilitated with enzymes known as proteases. In the same way, detrital matter can be a source of amino acids.
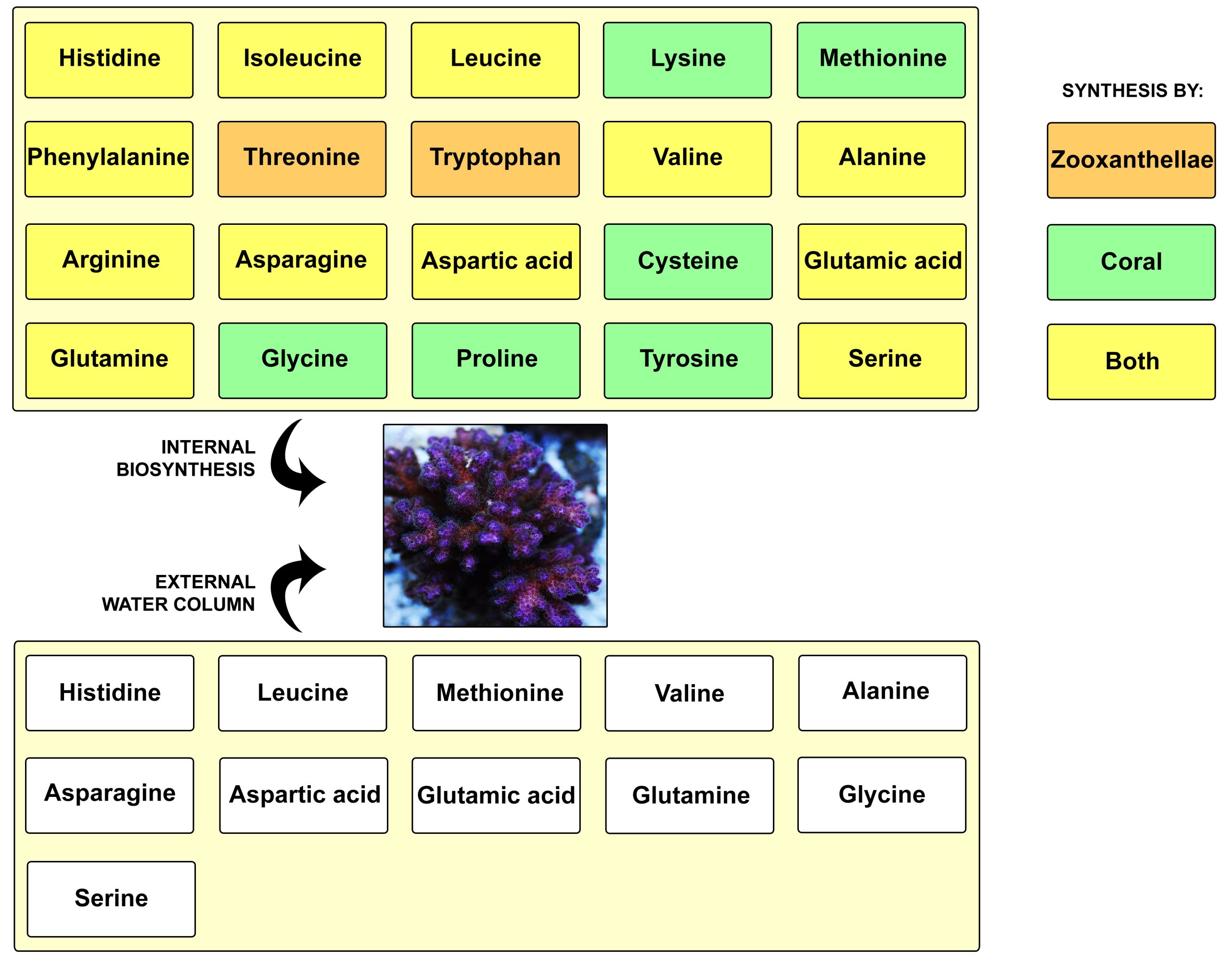
Overview of the two major amino acid sources for scleractinian corals; biosynthesis and uptake via the water column. Together, corals and zooxanthellae can synthesize 20 different amino acids, with some produced by the coral, others by the zooxanthellae, and yet others by both partners. It is important to note, however, that different coral species have different synthesis abilities. Amino acids can also be taken up via plankton, detritus and in dissolved form, which is known as heterotrophic feeding. The acquired amino acids are used by the coral to synthesize proteins for tissue growth, calcification and other processes (image: T. Wijgerde, based on Fitzgerald and Szmant 1997; Grover et al. 2008 and Shinzato et al. 2011, 2014).
When taking all sources together, we can see that corals can obtain all required amino acids, either via biosynthesis or uptake from the water in dissolved or particulate form. The question is, however, whether this amino acid supply is sufficient to maximise growth, i.e. whether it limits coral growth under natural conditions (see below).
The role of amino acids in coral feeding and growth
Feeding
Similar to other animals, amino acids play important roles in the lives of corals, being essential to enzyme production, tissue growth and skeleton formation. In addition, amino acids are known to affect coral feeding behaviour. After the addition of frozen or live feeds to an aquarium, corals are often observed to extend their tentacles, a phenomenon which may be attributed to amino acids. Goreau et al. (1971) found that addition of the amino acids glycine, alanine or glutamate to the water resulted in opening of the mouth, tentacle extension, swelling of tissue and expulsion of digestive filaments for many scleractinian corals. Thus, these amino acids elicit the same feeding response as when zooplankton is supplied. It is possible that corals have receptors which recognize organic compounds, including amino acids. This ability to detect specific chemical compounds, known as chemotaxis, may allow coral polyps to recognize zooplankton and prepare for prey capture.
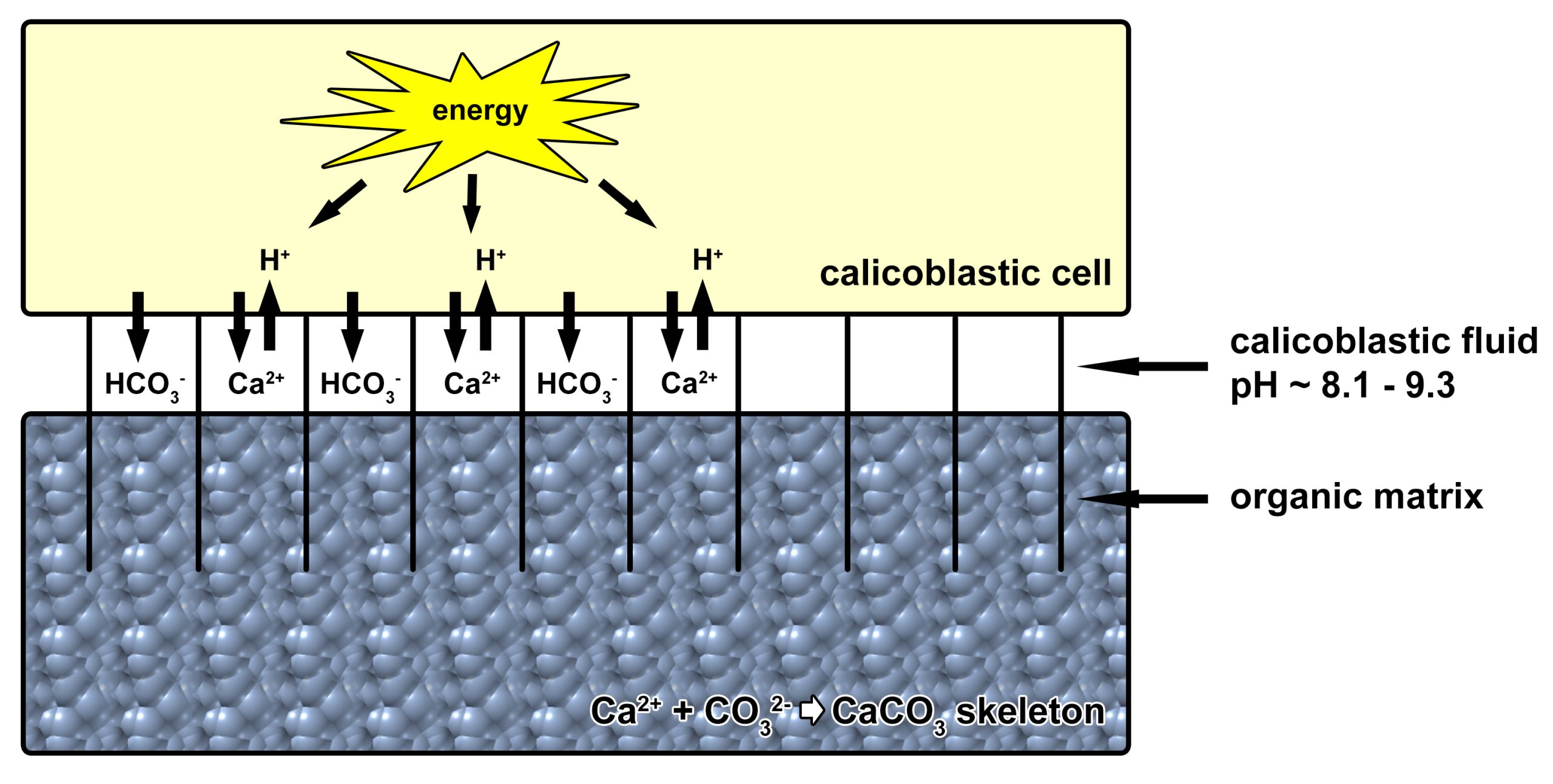
Schematic overview of coral calcification. Energy is produced by calicoblastic cells, which line the coral skeleton at the underside of coral polyps. This energy is used to pump calcium ions into and protons out of a thin layer of fluid between the coral’s tissue and skeleton, known as the calicoblastic fluid (CF). In addition, bicarbonate ions are transported to the CF, and generated in the CF from metabolically derived CO2 by the enzyme carbonic anhydrase (not shown). The high concentration of calcium and (bi)carbonate ions, together with a high pH, results in precipitation of calcium carbonate crystals around the organic matrix. This matrix regulates and accelerates calcium carbonate deposition, and acts as “rebar”, providing strength. An essential component of the organic matrix is the amino acid aspartic acid (image: T. Wijgerde, based on Furla et al. 2000, Al-Horani et al. 2003a,b, Allemand et al. 2004 and Venn et al. 2011).
Skeletal growth
The important role of amino acids in the growth of living organisms is well-documented, and scleractinian corals are no exception. Not only do corals require amino acids to build protein for tissue growth, they also need these for synthesis of a so-called organic matrix (Allemand et al. 2004). This organic matrix is a framework of proteins, polysaccharides, glycosaminoglycans, lipids and chitin, and is essential to biomineralisation (Wainwright 1963; Young et al. 1971; Constanz and Weiner 1988; Falini 1996). In many corals, it forms a physical link between soft tissue and the skeleton, regulates and accelerates calcium carbonate deposition in the skeleton (calcification), and acts as “rebar”, providing strength (Allemand et al. 2004). The building blocks of the organic matrix are secreted by coral cells into the skeleton, and this matrix is similar to that of animal bones and fish otoliths, and the cuticle of chicken eggshells. Organic matrices are also found in the skeletons of black corals (order Antipatharia) and hydrocorals (order Anthoathecata), the internal axis of gorgonians (order Alcyonacea), and the sclerites-needle-like calcium carbonate structures which provide strength-of soft corals (order Alcyonacea). The essential nature of an organic matrix for coral growth was demonstrated by Allemand et al. (1998), who were able to significantly reduce calcification of Stylophora pistillata by inhibiting organic matrix synthesis with specific drugs (emetin, cycloheximide and tunicamycin).
Although its molecular composition is diverse, the protein component of the organic matrix in coral skeletons is dominated by the amino acid aspartic acid. In scleractinian corals, aspartic acid, glutamic acid and glycine make up a significant portion of organic matrix proteins, with aspartic acid accounting for up to 37% (Table 2). Aspartic acid is even more dominant in the spicules embedded in the internal axis of gorgonians, with up to 74.3% contributing to organic matrix proteins. For the hydrocoral Millepora alcicornis, a distant relative of true corals, we find a similarly high figure of 56.4%.
% of organic matrix proteins | ||||||
---|---|---|---|---|---|---|
amino acid | Porites porites | Acropora palmata | Agaricia fragilis | Eunicea tourneforti | Gorgonia sp. | Millepora alcicornis |
aspartic acid | 34.3 | 37.00 | 30.00 | 74.30 | 65.30 | 56.40 |
glutamic acid | 10.00 | 12.50 | 10.30 | 3.86 | 5.78 | 11.00 |
threonine | 2.09 | 3.09 | 2.54 | 1.29 | 0.63 | 4.41 |
serine | 2.92 | 5.80 | 5.57 | 1.31 | 0.65 | 4.30 |
proline | 3.54 | 3.83 | 3.96 | 1.05 | 1.26 | 1.88 |
cysteine | 0.19 | 0.96 | 0.11 | |||
methionine | 1.14 | 1.21 | 0.10 | 0.17 | ||
glycine | 11.40 | 12.00 | 17.10 | 7.91 | 9.80 | 5.42 |
alanine | 6.68 | 4.73 | 12.20 | 6.02 | 11.00 | 4.31 |
valine | 5.58 | 4.57 | 5.56 | 1.36 | 1.74 | 2.30 |
isoleucine | 4.60 | 2.18 | 2.38 | 0.51 | 0.94 | 1.67 |
leucine | 6.87 | 4.36 | 4.50 | 0.59 | 1.35 | 2.23 |
tyrosine | 0.99 | 0.80 | 0.78 | 0.12 | 0.12 | |
phenylalanine | 3.33 | 2.13 | 2.79 | 0.33 | 0.55 | 0.80 |
histidine | 1.19 | 0.21 | 0.14 | 0.16 | 0.71 | |
lysine | 2.73 | 4.89 | 0.42 | 0.46 | 0.13 | 2.95 |
arginine | 1.32 | 1.17 | 0.38 | 0.43 | 0.30 | 0.64 |
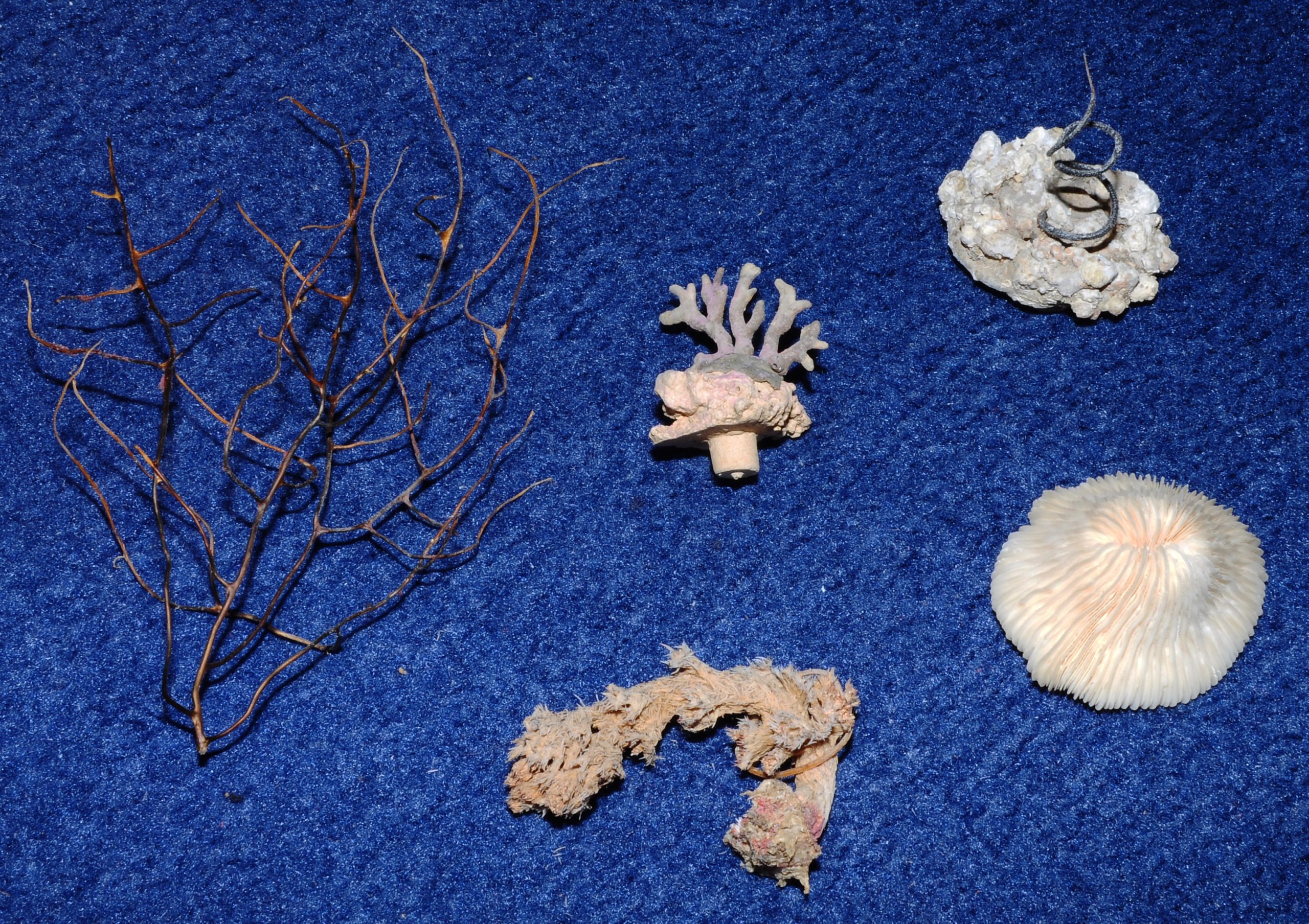
Organic matrices are found in the skeletal components of all corals, including gorgonians (left), hydrocorals (Distichopora sp., upper middle), black corals (Cirrhipathes sp., upper right), scleractinian corals (Cycloseris sp., lower right), and soft corals (Dendronephthya sp., lower middle, image: T. Wijgerde).
As the organic matrix of corals exhibits an abundance of aspartic acid, it stands to reason that its supply is critical to coral growth. Indeed, feeding scleractinian corals with daily batches of zooplankton (concentration range of 1,000-13,000 prey L-1 seawater) has been found to enhance tissue and skeletal growth, which may be partly caused by increased supply of aspartic acid and other amino acids, enhancing organic matrix synthesis and thus calcium carbonate deposition (Lavorano et al. 2008; Houlbrèque and Ferrier-Pagès 2009; Osinga et al. 2011). As providing scleractinian corals with higher than natural food quantities results in increased growth rates, coral growth on reefs is likely limited by food availability, and coupled to that the supply of amino acids, lipids and other organic compounds. In the same way, corals growing in deep waters or low flow areas can be naturally growth-limited by light availability and water flow rate (Wijgerde and Tilstra 2014 and references therein).
Should concentrated amino acids be added to the aquarium?
For aquarists, this is one of those Million Dollar Questions. The answer is not that straightforward, however. On the one hand, just as we humans do not require amino acids in fancy pills to stay healthy, so too can corals make do without amino acid supplements if they follow a natural diet. In the case of corals, a natural diet constitutes regular feedings with live or frozen zooplankton (<10 prey L-1 seawater) and phytoplankton (~1,000,000 cells L-1 seawater), depending on species (Yahel et al. 1998; Heidelberg et al. 2004, 2010; Holzman et al. 2005; Yahel et al. 2005a,b; Palardy et al. 2006). On the other hand, although corals can grow under low amino acid concentrations and only small amounts of zooplankton, they are likely growth-limited under such a natural food availability. Thus, providing corals with higher than natural food quantities can increase growth rates (Lavorano et al. 2008), although this is not always the case (Forsman et al. 2011). In this respect, dosing concentrated amino acids may enhance coral growth, in the same way as batch feeding with zooplankton can do this. This theory is supported by the findings of Grover et al. (2008), who found that amino acid uptake by corals can be enhanced by artificially increasing the amino acid concentration. If an aquarium is heavily stocked with corals, it can be especially useful to feed copious amounts of plankton or dose concentrated amino acids on a daily basis, to compensate for the high grazing pressure.
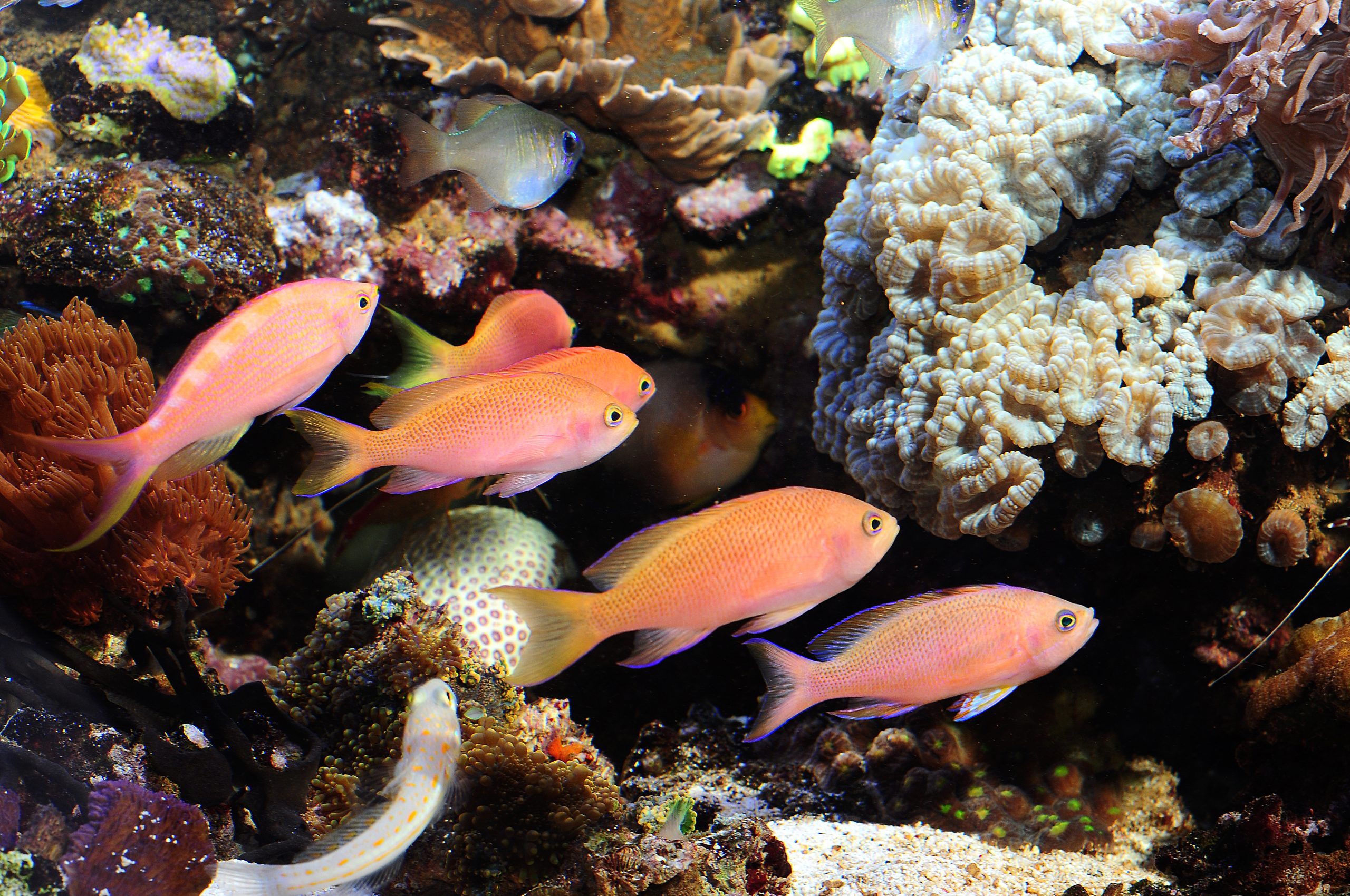
As they usually are well-stocked with fish, most home aquaria receive a significant amount of amino acids via artificial feeds (image: T. Wijgerde).
Despite our scientific knowledge on amino acids and corals, there are no studies that show how dosing concentrated amino acids affects corals. In this perspective, there seems to be uncharted territory for both aquarists and scientists, who can test the effects of concentrated amino acids on coral growth and coloration. Aspartic acid is an interesting candidate to start with, given its important role in organic matrix synthesis and skeletal growth. It is possible that corals which receive a concentrated aspartic acid supplement on a daily basis show faster growth compared to corals fed with zooplankton (which may provide less aspartic acid). The outcome of such an experiment is determined by whether corals fed with zooplankton are growth-limited by their combined internal and external aspartic acid supply. When sufficient amino acids including aspartic acid are available in dissolved or particulate form, it may not matter what the source is. Thus, aquarists who already feed their corals heavily may not see an added effect of concentrated amino acids such as aspartic acid.
For an experiment to have value, it is important to adhere to scientific standards, with sufficient replication and controls for solid conclusions. This means that one would have to use at least two separated aquaria to which amino acids are dosed, with several coral fragments in each aquarium, and at least two additional separate aquaria with fragments from the same parent colony receiving an alternative feed, or no feed at all, as controls to allow for comparison. In addition, all other environmental conditions, such as light intensity and spectrum, water flow rate, and water quality should be highly similar between the four aquaria to prevent confounding effects. Of course, it is possible that different species, and different genotypes (genetically distinct individuals) within species respond differently to concentrated amino acids (Osinga et al. 2011).
Concluding remarks
To conclude, it is fair to say that amino acids are highly important compounds for corals and other (marine) life. Whether you choose to supply your aquarium with amino acids via various feeds or in dissolved form is up to you. Either way, corals are highly adaptive creatures, and in many cases they will find a way to meet their minimum amino acid demand to stay alive and grow, either via biosynthesis or uptake from the water.
References
- Al-Horani FA, Al-Moghrabi SM, de Beer D (2003a) The mechanism of calcification and its relation to photosynthesis and respiration in the scleractinian coral Galaxea fascicularis. Mar Biol 142:419-426
- Al-Horani FA, Al-Moghrabi SM, de Beer D (2003b) Microsensor study of photosynthesis and calcification in the scleractinian coral, Galaxea fascicularis: active internal carbon cycle. J Exp Mar Biol Ecol 288:1-15
- Allemand D, Ferrier-Pagès C, Furla P, Houlbrèque F, Puverel S, Reynaud S, Tambutté É, Tambutté S, Zoccola D (2004) Biomineralisation in reef-building corals: from molecular mechanisms to environmental control. C R Palevol 3:453-467
- Allemand D, Tambutté E, Girard JP, Jaubert J (1998) Organic matrix synthesis in the scleractinian coral Stylophora pistillata: role in biomineralization and potential target of the organotin tribulyltin. J Exp Biol 201:2001-2009
- Al-Moghrabi S, Allemand D, Jaubert J (1993) Valine uptake by the scleractinian coral Galaxea fascicularis: characterization and effect of light and nutritional status. J Comp Physiol B 163:355-362
- Constantz B, Weiner S (1988) Acidic macromolecules associated with the mineral phase of scleractinian coral skeletons. J Exp Zool 248:253-258
- Falini G, Albeck S, Weiner S, Addadi L (1996) Control of aragonite or calcite polymorphism by mollusk shell macromolecules. Science 271:67-69
- Ferrier MD (1991) Net uptake of dissolved free amino acids by four scleractinian corals. Coral Reefs 10:183-187
- Fitzgerald LM, AM Szmant (1997) Biosynthesis of ‘essential’ amino acids by scleractinian corals. Biochem J 322:213-221
- Forsman ZH, Kimokeo BK, Bird CE, Hunter CL, Toonen RJ (2011) Coral farming: effects of light, water motion and artificial foods. J Mar Biol Assoc UK, doi:10.1017/S0025315411001500
- Furla P, Galgani I, Durand I, Allemand D (2000) Sources and mechanisms of inorganic carbon transport for coral calcification and photosynthesis. J Exp Biol 203:3445-3457
- Fürst P, Stehle P (2004) What are the essential elements needed for the determination of amino acid requirements in humans? J Nutr 134:1558S-1565S
- Goreau TF, Goreau NI, Yonge CM (1971) Reef corals: autotrophs or heterotrophs? Biological Bulletin 141:247-260
- Grover R, Maguer J-F, Allemand D, Ferrier-Pagès C (2008) Uptake of dissolved free amino acids (DFAA) by the scleractinian coral Stylophora pistillata. J Exp Biol 211:860-865
- Heidelberg KB, O’Neil KL, Bythell JC, Sebens KP (2010) Vertical distribution and diel patterns of zooplankton abundance and biomass at Conch Reef, Florida Keys (USA). J Plankt Res 32:75-91
- Heidelberg KB, Sebens KP, Purcell JE (2004) Composition and sources of near reef zooplankton on a Jamaican forereef along with implications for coral feeding. Coral Reefs 23:263-276
- Hoegh-Guldberg O, Williamson J (1999) Availability of two forms of dissolved nitrogen to the coral Pocillopora damicornis and its symbiotic zooxanthellae. Mar Biol 133:561-570
- Holzman R, Reidenbach MA, Monismith SG, Koseff JR, Genin A (2005) Near-bottom depletion of zooplankton over a coral reef II: relationships with zooplankton swimming ability. Coral Reefs 24:87-94
- Houlbrèque F, Ferrier-Pagès C (2009) Heterotrophy in tropical scleractinian corals. Biol Rev Camb Philos 84:1-17
- Markell DA, Trench RK (1993) Macromolecules exuded by symbiotic dinoflagellates in culture: amino acid and sugar composition. J Phycol 29:64-68
- Mitterer RM (1978) Amino Acid Composition and Metal Binding Capability of the Skeletal Protein of Corals. Bull Mar Sci 28:173-180
- Osinga R, Schutter M, Griffioen B, Wijffels RH, Verreth JAJ, Shafir S, Henard S, Taruffi M, Gili C, Lavorano S (2011) The biology and economics of coral growth. Mar Biotechnol 13:658-671
- Palardy JE, Grottoli AG, Matthews KA (2006) Effect of naturally changing zooplankton concentrations on feeding rates of two coral species in the Eastern Pacific. J Exp Mar Biol Ecol 331:99-107
- Reeds PJ (2000) Dispensable and indispensable amino acids for humans. J Nutr 130:1835S-1840S
- Reimer J (2013). Zoanthus flos-marinus Duchassaing & Michelotti, 1860. Accessed through: World Register of Marine Species at http://www.marinespecies.org/aphia.php?p=taxdetails&id=590575 on 2014-02-11
- Shinzato C, Inoue M, Kusakabe M (2014) A Snapshot of a Coral ”Holobiont”: A Transcriptome Assembly of the Scleractinian Coral, Porites, Captures a Wide Variety of Genes from Both the Host and Symbiotic Zooxanthellae. PLoS ONE 9(1): e85182. doi:10.1371/journal.pone.0085182
- Shinzato C, Shoguchi E, Kawashima T, Hamada M, Hisata K, Tanaka M, Fujie M, Fujiwara M, Koyanagi R, Ikuta T, Fujiyama A, Miller DJ, Satoh N (2011) Using the Acropora digitifera genome to understand coral responses to environmental change, Nature 476:320-323
- Srinivasan G, James CM, Krzycki JA (2002) Pyrrolysine Encoded by UAG in Archaea: Charging of a UAG-Decoding Specialized tRNA. Science 296:1459-1462
- Swanson R, Hoegh-Guldberg O (1998) Amino acid synthesis in the symbiotic sea anemone Aiptasia pulchella. Mar Biol 131:83-93
- Venn A, Tambutté E, Holcomb M, Allemand D, Tambutté S (2011) Live Tissue Imaging Shows Reef Corals Elevate pH under Their Calcifying Tissue Relative to Seawater. PLoS ONE 6(5): e20013. doi:10.1371/journal.pone.0020013
- von Holt C (1968) Uptake of glycine and release of nucleoside-polyphosphates by zooxanthellae. Comp Biochem Physiol 26:1071-1079
- von Holt C, von Holt M (1968) Transfer of photosynthetic products from zooxanthellae to coelenterate hosts. Comp Biochem Physiol 24:73-81
- Wainwright SA (1963) Skeletal organization in the coral, Pocillopora damicornis. Q J Microsc Sci 104:169-183
- Wang JT, Douglas AE (1999) Essential amino acid synthesis and nitrogen recycling in an alga-invertebrate symbiosis. Mar Biol 135:219-222
- Wijgerde T (2012) Aiptasia, dinoflagellate algae and cyanobacteria – a three-way symbiosis? Advanced Aquarist 11(4)
- Wijgerde T, Tilstra A (2014) Debunking Aquarium Myths. Advanced Aquarist 13(2)
- Yahel G, Post AF, Fabricius K, Marie D, Vaulot D, Genin A (1998) Phytoplankton distribution and grazing near coral reefs. Limnol Oceanogr 43:551-563
- Yahel R, Yahel G, Berman T, Jaffe JS, Genin A (2005a) Diel pattern with abrupt crepuscular changes of zooplankton over a coral reef. Limnol Oceanogr 50:930-944
- Yahel R, Yahel G, Genin A (2005b) Near- bottom depletion of zooplankton over coral reefs: I: diurnal dynamics and size distribution. Coral Reefs 24:75-85
- Young SD, O’Connor JD, Muscatine L (1971) Organic material from scleractinian coral skeletons. II. Incorporation of 14C into protein, chitin and lipid. Comp Biochem Physiol 40B:945-958
0 Comments