With full realization that I’ll get email stating that ‘clams are not corals’, I can not be content to leave it at that. So, I will make an even bolder statement – Tridacna clams’ tissues (along with certain other genera) are colorless and contain no spectacular pigments. You are paying good money for an optical illusion. But what an illusion!
This month, we will continue our observations of marine invertebrate coloration with a slightly different subject – that of the impressive appearance of photosynthetic clams (mostly Tridacna and Hippopus species, but also including Fragum, and Corculum specimens). Strangely enough, and contrary to information in many hobbyist references, these clams do not contain colorful pigments. The ‘metallic’ iridescent colors we often see are caused by light reflected from tiny internal structures. Also involved are refraction, light interference and possibly diffraction. To understand why these colors are apparent necessitates a review of a few optical physics terms.
Definitions and Examples
Coral coloration (due to reflection and fluorescence) is relatively simple when compared to the science involved with clam coloration. Take a deep breath, and we’ll get started.
Diffraction: Bending of light waves around an object. The colorful surfaces of CDs and DVDs are good examples of diffraction.
Hue: One of three attributes of color (along with chroma and lightness). This attribute is described with names such as ‘red’, ‘yellow’, etc.

Figure 1. A common example of interference. These bubbles do not, nor do Tridacna clams, contain pigments that make them iridescent. Light reflected from the inside and outside surfaces of the bubble recombine and, if out of phase, cause constructive interference and different hues. If in phase, no color is apparent due to destructive interference.
Interference: A characteristic of light waves under certain circumstances to reinforce or cancel hue. Reinforcement is called ‘constructive‘ interference while cancellation is called ‘destructive‘ interference. Interference is caused by light waves traveling at different speeds and staying in or out of phase. If the light beams are of the same orientation after reflection/refraction, no color will be apparent. However, certain colors will be amplified if beams are out of phase (within a very small range).
An example of interference can be seen when two pebbles are dropped into a tub of water. Some waves will combine and become larger while others’ energies meet and ‘cancel out.’ Basically, the same thing can happen with light waves during constructive or destructive interference.
The colors of soap bubbles or oil on water (and Tridacna iridescence) are due to constructive and destructive interference (See Figure 1).
For a tutorial on interference, see:
http://www.glenbrook.k12.il.us/gbssci/phys/class/waves/u10l3c.html
Iridophore: For our purposes, iridophores are the iridescent patches of color scattered across the mantle of Tridacna clams. An iridophore consists of numerous smaller bodies or cells called iridocytes. In turn, iridocytes contain uniform, regularly spaced stacks (‘laminate’ iridosomes) of sulfur-rich protein called iridosomal platelets. See Figure 2.
Iridophores and their various substructures have been described in T. maxima (Fankboner, 1971; Farmer et al., 2001; Kawaguti, 1966); T. gigas (Griffiths et al., 1992); T. crocea (Griffiths et al., 1992); T. squamosa (Fankboner, 1971); Hippopus hippopus (Griffiths et al., 1992); and T. tevoroa (Klumpp and Lucas, 1994). It is believed other photosynthetic clams contain them as well.
Note that clam iridophores are made of different proteins than many other animals’ iridophores. For instance, cephalopod (squid, octopus, etc.) iridophores are based on a different material (guanine, which is why their iridophores are sometimes called guanophores. If that sounds a little odd – it is. Guanine was originally isolated from guano, or bird poop. This little known fact can make you the life of the next black tie cocktail party you attend…and make you wonder about the motivation of some scientists!).

Figure 2. Tridacna reflective (and refractive/diffractive) proteins are arranged in neat rows. After a photomicrograph by Griffiths et al., 1992.
Iridescence: An optical phenomenon in which hue (‘color’) changes with the viewing angle.
Reflection: The ‘return’ or ‘bouncing back’ of light waves, sometimes (but not always) without alteration of the light and incident angle.
Refraction: Deviation or ‘bending’ of light waves as they pass from one medium to another of different optical density. The amount of ‘bending’ caused by a material is called its refractive index.
Mirages are due to refraction caused by layers of cool and warm air.
Schemochromes or ‘Structural Colors’: Those colors caused by presence of tiny light-reflecting structures and optical physics such as refraction, interference and others. Schemochromes are the opposite of biochromes (which contain true colored pigments). Therefore, schemochromes are not actually pigments but only an optical illusion caused by structural properties (and widely seen in many bird feathers, reptile and fish scales and tissues, insects and marine invertebrates).
To stitch this information together – the ‘metallic’, iridescent colors displayed by Tridacna and other taxa are not true pigments (biochromes) – they are schemochromes. Schemochromes are due to physical structures within tissues. In the case of colorful clams, these structures are called iridophores. Iridophores contain uniform, protein-rich cells called iridosomal platelets. When light strikes the iridosomal platelets, it is reflected but not without modification. The color reflected is dependent upon the refractive index of the protein, its orientation, number of platelets in the stack, angle of illumination and other factors. Thus, light falling upon the platelets is refracted (due to different optical densities). Construction and destructive interference now come into play. Again, depending upon the refractive index and possibly the orientation of the proteins, interference can reinforce certain colors, or cancel them. These refracted/reflected light waves can be
very dramatic in appearance if the background is dark.
If the exact refractive index is known (and, to my knowledge, it is not for Tridacna and others), then the apparent color can be predicted. Constructive interference (assuming a refractive index of 1.42) would occur at wavelengths in the range of ultraviolet (355nm) through blue (468nm; Griffiths et al., 1992)*. In other words, the structural colors reflect ultraviolet light (which we cannot see) and those visible wavelengths of violet and blue (which is the color of many clams’ mantles). Different refractive indices could lend any number of visible colors.
* Note: These researchers believed preparation of mantle samples may have caused some shrinkage, thus throwing a small monkey wrench into the works. When the calculation is ‘corrected’ for this potential error, the constructive interference range is extended into the green portion of the spectrum (and, as we know, violet, blue and greens are quite visible in mantles’ reflected light).
Discussion
If we have in mind a concept of what causes clam iridescence, then the next logical question is ‘why?’
The most popular theory seems to be one of photoprotection, where potentially harmful ultraviolet (UV) radiation and Photosynthetically Active Radiation (PAR, especially in the violet/blue/green portions of the spectrum) are simply reflected away from the animal and its symbiotic algae.
A simple test, though certainly not conclusive, would be to compare clam coloration to depth. Theoretically, clams reflecting blue and green light would be found in shallow depths. See Table 1.
Species | Common Depth | Common Colors |
---|---|---|
Tridacna crocea | to ~6m | Blue, Green |
Tridacna maxima | Common to 7m, but to ~20m | Blue, Green, Violet |
Tridacna squamosa | To 15-20m | Golden Brown |
Tridacna trevoroa | 20-33m | Brownish-Gray |
Tridacna gigas | 20m | Green-Brown |
Tridacna derasa | 4-10m, but to 25m | Golden Brown |
Hippopus hippopus | to ~6m | Dull Greenish Brown |
Before continuing, it should be noted that any attempt to associate fashion (coloration, in this case) with function is notoriously difficult. However, with that said, generally we see blue and green coloration associated with clams often found in shallow depths, with yellow-browns at deeper depths. However, there are major exceptions to this apparent trend where clams of a single species at the same depth display radically different coloration (see Fatherree, 2006 for some striking examples). Hippopus specimens also buck the trend.
So, other factors should be considered, such as genetic variability among specimens and possible effects of environmental factors. ‘Types’ (clades) of zooxanthellae found in photosynthetic clams should be studied when examining maximal depth ranges of clams.
Genetic Differences Between Differently Colored Tridacna gigas and Effects of Light Intensity and Quality on T. maxima
Burton (1997) used a laboratory procedure called electrophoresis in an attempt to link mantle ‘coloration’ and genetic variations. No clear relationship was established (although some interesting anomalies were observed in some of his colorful T. gigas specimens). This researcher believed unspecified environmental conditions (perhaps in conjunction with genetic predisposition) could cause ‘expression of color’.
The most common environmental factor mentioned in hobby literature is that of light intensity or spectral quality. Alo (2005), in tests involving almost 400 T. maxima specimens distributed in 12 greenhouse systems and using different lighting setups in each system (‘full greenhouse’ light – peak PAR of 1,300-1,400mmol×m²×sec; ‘shaded greenhouse’ light – peak PAR of 700-1,000 mmol×m²×sec; and 10,000K metal halide light – maximum PAR of 1,450 mmol×m×sec, decreasing to 280 mmol×m²×sec (!) after 13 weeks), found only one T. maxima to shift coloration (from green to yellow) during the experiment’s progress (this specimen was held in a ‘shaded greenhouse’ tank). However, during the clams’ light acclimation period (an initial 5-hour photoperiod increased by 30 minutes daily until a 12-hour photoperiod was obtained) fourteen clams changed from green to gold, and two switched from blue to gold.
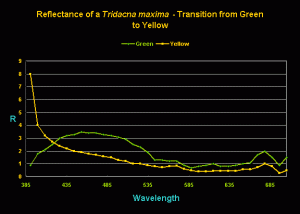
Figure 3. Reflectance of a single T. maxima specimen before and after its transition from ‘green’ to ‘yellow’. (The ‘R’ legend on the y-axis stands for ‘reflectance’.)
Of interest to many hobbyists is one of Alo’s observations – those clams maintained under the 10,000K metal halide lamps never expanded their mantles as fully as those exposed to natural sunlight of any intensity. His work suggests spectral quality was in play, and he attributes statistically significant high clam mortality to the UV-A spike at 365nm in the metal halide’s spectrum (which is an interesting observation, since other researchers have suggested refractive/reflective qualities produced by iridophores protect mantle tissues/zooxanthellae from UV-A radiation. Since the metal halide lamps were suspended 20 cm (8 inches) above the water surface, is it possible that infrared radiation – heat – generated by the lamps was transferred to the clams resulting in stress?). For those wondering, Alo keep careful records of nutrients within his systems.
This researcher also includes some interesting work determining reflectance of clams’ mantles using an Ocean Optics spectrometer and its software (See Figures 3, 4 and 5).
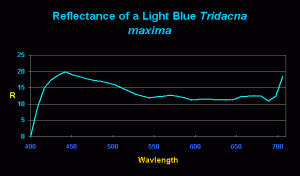
Figure 4. Reflectance of a ‘light blue’ T. maxima. Note the unusual and very strong reflectance at ~440nm – ~20% of the light falling upon the mantle is reflected. There is a muted spectral signature of zooxanthellae seen at 575nm and 650nm.
And… A Final Clue
If Tridacna clams’ coloration plays a part in preventing zooxanthellae from getting too much light, we would see this as lack of photoinhibition in photosynthesis (or specifically, a linear increase of electron flow as light intensity increases – this determined by a PAM fluorometer).
And this is exactly what we see (as shown in Figure 6). However, this is not proof that the mantle coloration is responsible – it could be due to self-shading of zooxanthellae within the relatively thick mantle, or some other factor.
This experiment was conducted while using an Iwasaki 400-watt ‘daylight’ metal halide as the illumination source. No photosaturation (much less dynamic photoinhibition) was noted at PAR values approaching 600 µmol·m²·sec, or ~30,000 lux. This does not mean the clam requires this amount of light; merely that it can tolerate it. T. maxima specimens can do just fine when maintained under much less light (200-300 µmol·m²·sec, or 10,000-15,000 lux, is generally sufficient).
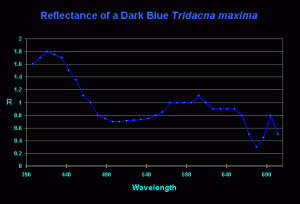
Figure 5. Reflectance of a ‘dark blue’ T. maxima. Compare this weak reflectance to that of the ‘light blue’ clam in Figure 4. Also interesting is the distinct ‘crown’ reflection at ~575nm, 600nm and 650nm- the typical spectral signature of zooxanthellae. Why do reflectances shown in Figures 3 and 4 lack this pronounced feature?
Zooxanthellae Clades Found in Giant Clams
As we near the closing of our discussion, we should examine the ‘types’ of zooxanthellae known to infect Tridacna clams. Reasons for including this information are two-fold. First, it establishes that clams of the same species can contain different zooxanthellae ‘types’ (clades). It also suggests that animals in the listings could possibly have the same light requirements.
As to the real question – Is there a genetic link between a particular clam recognizing a specific symbiont clade and a need for photoprotection via optical manipulations?
A3 – Found in a Tridacna clam (species unreported, Baille et al., 2000), Tridacna crocea, T. maxima, T. derasa, T. gigas, and another ‘giant clam’ (Hippopus hippopus; LaJeunesse, 2001). Clade A3 is tolerant of higher light levels (Hennige et al., 2006). Known hosts include the jellyfish Cassiopeia mertensii from Hawaii (LaJeunesse, 2001; LaJeunesse et al., 2004), Montastrea faveolata (Belize, across a depth range of 2 to 8m), a Belizean stony coral (Siderastrea intersepta, @ 8-15m; Warner et al., 2006), the anemone Condylactis gigantea, stony corals Acropora palmata, shallow-water Acropora cervicornis, and Stephanocoenia michelini. A3 zooxanthellae are known to produce 1 ultraviolet-absorbing compound – the MAA mycosporine-glycine (Banaszak et al., 2006).
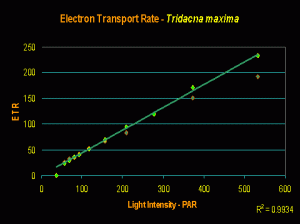
Figure 6. Rate of photosynthesis (Electron Transport Rate, or ETR) of a Tridacna maxima clam under an artificial light source.
C1 – Found in Tridacna derasa (1-8m); T. gigas (1-8m) and T. maxima (1-8m). Clade C1 is considered a ‘generalist’ zooxanthellae in that it infects a large number of photosynthetic hosts in the Pacific as well as the Atlantic, and is distributed over a wide variety of depths. Thus, corals and clams containing this clade could be considered highly adaptive in that they can tolerate high light but are probably best suited for lower light intensities. Clade C1 (along with C3, C21, C3d, C1c and C45) is believed to be an ancestral type from which other clades evolved (LaJeunesse, 2004). Acropora cervicornis (Baker et al., 1997), Acropora divaricata, A. humilis, A. hyacinthus, A. longicyathus (from the GBR; van Oppen et al., 2001), Acropora palifera (from Taiwan, Chen et al., 2005), Acropora sarmentosa , A. tenuis (GBR; Van Oppen et al., 2001), Astreopora (GBR, LaJeunesse et al., 2003), Astreopora myriophthalma (Taiwan; Chen et al., 2005), Caribbean anemones Bartholomea and Condylactis spp. (LaJeunesse et al., 2003), Great Barrier Reef ‘corallimorpharia’ and Coscinaraea, Coscinaraea wellsi and Cycloseris vaughani from Hawaii (LaJeunesse et al., 2004), Cyphastrea (LaJeunesse et. al., 2003), Cyphastrea chalcidicum (van Oppen, 2005), Atlantic and Pacific Discosoma spp. (LaJeunesse, 2005), Echinophyllia orpheensis, Echinophyllia lamellosa (Chen, 2005), Caribbean Eunicea (LaJeunesse et. al., 2003), Euphyllia ancora, Euphyllia glabrescens (Chen, 2005), Favia (LaJeunesse et. al., 2003), Favia favus and Favites abdita from Taiwan (Chen, 2005), Fungia (LaJeunesse et. al., 2003), Fungia crassa (van Oppen, 2005), Galaxea, Goniastrea (LaJeunesse et. al., 2003), Goniastrea rectiformis (Chen, 2005), Goniopora (LaJeunesse et. al., 2003), Goniopora columba, Goniopora lobata (Chen, 2005), Herpolitha, Hydnophora (LaJeunesse et. al., 2003), Hydnophora excessa (Chen, 2005), Icilogorgia, Lebruna, Leptastrea (LaJeunesse et. al., 2003), Leptoria phrygia (Chen, 2005), Leptoseris incrustans (LaJeunesse, 2004), Linuche, Lobophytum, Merulina (LaJeunesse et. al., 2003), Merulina ampliata (Chen, 2005), Merulina scrabicula (van Oppen, 2005), Millepora sp. (LaJeunesse et. al., 2003), Montipora aequituberculata (Chen, 2005), Montipora cactus from Indonesia (van Oppen, 2004), Montipora cactus, Montipora curta from Taiwan (Chen, 2005), Montipora confusa (van Oppen, 2004), Montipora digitata, Montipora effluorescens, Montipora hispida, Montipora sp., Montipora spongodes, Montipora undata from Taiwan (Chen, 2005), Mycedium (LaJeunesse et. al., 2003), Mycedium elephantotus (Chen, 2005), Pachyseris, Paulastrea, Caribbean Palythoa, Hawaiian Palythoa (LaJeunesse et. al., 2003), Pavona desucata, Pavona frondifera, Pavona varians, Pavona venosa (Chen, 2005), the ‘bubble’ coral Plerogyra (LaJeunesse et. al., 2003), Plesiastrea verispora (Chen, 2005), gorgonians Plexaura and Plumigorgia (LaJeunesse et. al., 2003), Taiwanese Pocillopora damicornis (Chen, 2005), Polyphyllia, Porites sp. (LaJeunesse et. al., 2003), shallow-water Porites cylindrica, Porites lutea, Porites solida (from Taiwan; Chen, 2006), GBR Psammocora (LaJeunesse et. al., 2003), Pseudosiderastrea tayamai (Chen, 2005), Rhodactis, Rumphella, Sarcophyton, Pacific Scolymia, Siderastrea, Sinularia (LaJeunesse et. al., 2003), Stylocoeniella guentheri (Chen, 2005), Stylophora sp. ((LaJeunesse et. al., 2003), Stylophora pistillata (Chen, 2005), Turbinaria sp. (LaJeunesse et. al., 2003), Turbinaria mesenteria (Chen, 2005), Pacific and Caribbean Zoanthus spp. (LaJeunesse et. al., 2003).
Clam Coloration – Is It Camouflage?
Reflection of ultraviolet radiation could serve as a photoprotective measure to both host and symbiont, but is a poor idea for camouflage since many fishes can see well into the ultraviolet radiation range. Of course, the same applies to visible radiation. It would seem that clam mantle coloration is just the opposite of the best camouflage strategy – that of invisibility, such as offered by transparency, where background light is transmitted through tissues. Further, the apparent self-protection reaction made possible by clams’ photoreceptors (primitive ‘eyes’) to shadows is well known – the sudden closing of the valves, where water is forced out of internal cavities (usually enough to scare away predator fishes, such as Thalassoma wrasse spp. This, naturally, does not exclude camouflage’s potential of predator avoidance, if you will. However, some researchers believe mantle coloration acting as camouflage to be a remote possibility).
There is also the possibility that physical qualities of the mantle could enhance photosynthesis. However, there is very little evidence supporting this hypothesis and it will not be discussed here.
I present this information for those truly interested in pursuit of answers. Unfortunately, I can no longer work on this since NELHA donated their Tridacna clams to the Waikiki Aquarium.
It should be apparent that we have a fairly good understanding of how clams’ mantles are colorful, but a very poor one of why they appear this way.
This is an excellent project for a dedicated hobbyist to undertake. Quality spectrometers are becoming increasing affordable as diffraction gratings find their way into different applications, and a fair number of instruments are now in hobbyists’ hands. Those hobbyists willing to expend their time and money could be rewarded with unlocking mysteries of clam coloration – how they do it, why they do it – and perhaps turning those less-than-desirable specimens into ‘killer clams’.
I will be glad to assist in any way possible. Contact me at [email protected].
MACNA! Atlanta 2008! www.masna.org
References
- Alo, M., 2005. Survivorship, growth and pigmentation responses of the marine ornamental invertebrate Tridacna maxima to varied irradiance levels in two different culture systems. Master’s Thesis, University of Florida. 79 pp.
- Baillie, B., C. Belda-Baillie and T. Maruyama, 2000. Conspecificity and Indo-Pacific distribution of Symbiodinium genotypes (Dinophyceae) from giant clams. J. Phycol. 36:1153-1161.
- Baker, A. and R. Rowan, 1997. Diversity of symbiotic dinoflagellates (zooxanthellae) in scleractinian corals of the Caribbean and eastern Pacific. Proc. 8th Int. Coral Reef Symp., Panama. 2: 1301-1306.
- Brocco, S., 1977. The ultrastructure of the epidermis, dermis, iridophores, leucophores and chromatophores of Octopus dolfleini martini (Cephalopod: Octopoda) Ph.D. Thesis, University of Washington, Seattle.
- Burton, C., undated. Mantle colouration variation and genetic diversity in Lizard Island giant clams (Tridacna gigas). 14-23.
- Chen, C., Y-W Yang, N. Wei, W-S Tsai and L-S Fang, 2005. Symbiont diversity in scleractinian corals from tropical reefs and sub-tropical non-reef communities in Taiwan. Coral Reefs, 24(1): 11-22.
- Fankboner, P., 1971. Intracellular digestion of symbiotic zooxanthellae by host amoebocytes in giant clams (Bivalvia: Tridacnidae), with a note on the nutritional role of the hypertrophied siphonal epidermis. Bio. Bull. 141: 222-234.
- Farmer, M., W. Fitt and R. Trench, 2001. Morphology of the symbiosis between Corculum cardissa (Mollusca: Bivalvia) and Symbiodinium corculorum (Dinophycae). Biol. Bull., 200: 336-343.
- Fatherree, J., 2006. Giant Clams in the Sea and the Aquarium: The Biology, Identification, and Aquarium Husbandry of Tridacnid Clams. Liquid Medium, Tampa, Fla. 227 pp.
- Griffiths, D., H. Winsor and T. Luong-Van, 1992. Iridophores in the mantle of giant clams. Aust. J. Zool., 40: 319-326.
- Hennige, S., D. Suggett, M. Warner and D. Smith, 2006. Photoacclimation of Symbiodinium revisited: Variation of strategies with thermal tolerance? Natural Environment Research Council, University of Essex.
- Hirose, E., K. Iwai and T. Maruyama, 2006. Establishment of the photosymbiosis in the early ontogeny of three giant clams. Mar. Biol., 148(3).
- Johnsen, S. and H. Sosik, 2003. Cryptic coloration and mirrored sides as camouflage strategies in near-surface pelagic habitats: Implications for foraging and predator avoidance. Limnol. Oceanogr. 48(3): 1277-1288.
- Klumpp, D. and J. Lucas, 1994. Nutritional ecology of the giant clams Tridacna tevoroa and T. derasa from Tonga: Influence of light on filter-feeding and photosynthesis. Mar. Ecol. Prog. Ser., 107: 147-156.
- Knop, D., 1996. Giant Clams: A Comprehensive Guide to the Identification and Care of Tridacnid Clams. Dähne Verlag, Ettlingen. 255 pp.
- LaJeunesse, T., R. Bhagooli, M. Hidaka, L. de Vantier, T. Done, G. Schmidt, W. Fitt and O. Hoegh-Guldberg, 2004b. Closely related Symbiodinium species differ in relative dominance in coral reef host communities across environmental latitudinal and Biogeographical gradients. Mar. Ecol. Prog. Ser., 284: 147-161.
- Yoshihisa, K., undated. Organization and development of reflecting platelets in iridophores of the giant clam Tridacna crocea Lamarck: Developmental biology.
- Zoo. Sci., 7(1):63-72.
0 Comments