Photographs and videos by Tim Wijgerde, unless stated otherwise.
How it all started
In 2002, Charles Delbeek published an article about his experiences with Dendronephthya and other difficult to maintain corals in this magazine (Delbeek 2002). After reading it in 2005, I became excited about setting up an aquarium for difficult to maintain filter feeding animals such as sponges, tunicates, certain bivalves, crinoids, and corals such as Dendronephthya. I was a biology student at the time, and already kept a typical reef aquarium in my dormitory. It was then when I started browsing the scientific literature on reef life. Having access to the university library helped with that, and I soon had many pdf’s piling up on my hard drive.
Due to time, space and financial limitations, I never got to actually building my system. After completing a Master’s programme at Utrecht University, I was able to work with corals at Wageningen University between 2007 and 2009. Having spent all day with my hands in salt water, I was not eager to continue at home. In early 2010, I finally decided to go ahead. However, when my system was almost ready to go, I got the opportunity to return to Wageningen University to work on the effects of climate change and eutrophication on Caribbean corals (Porites porites and Agaricia agaricites). One year later, my work on coral feeding (Galaxea fascicularis) started to develop into a Ph.D. project, and together with the company Eco Deco, I got a grant to build an experimental coral nursery. Working long hours, I would not think about my filter feeder system again until after I finished my Ph.D. project in October of 2013.
In February 2014, I became restless again, and decided to push through this time. I designed an aquaculture system specifically for Dendronephthya corals, and with the help of Michaël and Manon Laterveer of Blue Linked, I launched a crowd funding campaign on Indiegogo.com. The goal of the project was to establish a culture protocol for Dendronephthya corals. This could benefit the sustainable coral trade and elevate the aquarium hobby to a higher level. Although we did not raise sufficient funds to carry out the project as planned, several corporate sponsors helped out by donating equipment. This allowed me to set up one basic culture system to conduct some preliminary experiments. Below, I will describe the findings of these experiments.
Meeting the needs of Dendronephthya corals
Dendronephthya corals are notorious for being difficult to maintain in aquaria, and success so far has been limited. Even though people have managed to keep these corals alive for over a year, growth rates do not compare to those measured in the wild. For example, in Israel, Dendronephthya corals can grow 30 cm (12 inches) in length in just a few months (Fabricius et al. 1995a), which is amongst the fastest coral growth rates ever recorded.
The works of Katharina Fabricius and coworkers (1995a,b) provide important clues about the proper care of Dendronephthya corals. It seems that three factors are essential; food, flow and filtration. Below, I will deal with these factors one at a time, and detail the materials and methods used to address them.
Food
Fabricius et al. (1995a,b) have showed that the Nephtheids Dendronephthya hemprichi, D. sinaiensis and Scleronephthya corymbosa mainly feed on phytoplankton, which in the laboratory includes Nannochloropsis, Isochrysis and Tetraselmis spp. Zooplankton capture is selective for weakly swimming bivalve and gastropod larvae, and contributes less than 5% to the corals’ growth and energy demand, at least in terms of organic carbon. Dendronephthya seem to have only few and small cnidocytes, making it difficult to them to paralyze and trap zooplankton prey. Video recordings have shown that Dendronephthya polyps have trouble with strongly swimming prey such as copepods (Fabricius et al. 1995a), which could explain their underrepresentation in polyp coelenterons (Table 1). Bivalve and gastropod larvae are weakly swimming and more easily captured, which may explain their overrepresentation in polyp guts (Table 1). Even though zooplankton does not seem a relevant food source for Dendronephthya corals in terms of organic carbon, it may provide essential nitrogenous components for growth, such as specific amino acids and proteins (see discussion).
The ability of octocorals to feed on phytoplankton is probably related to the narrowly spaced pinnules (side branches) on their tentacles. In addition, sclerites-calcareous needles embedded in soft tissue that provide colony stiffness-allow these coralsto live in strong flow. This enables them to filter significant amounts of particles from the water every day (Fabricius 1995a).These characteristics match well with the fact that plant-digesting carbohydrases (amylase and laminarinase) have been found in soft corals (Elyakova et al. 1981).
Table 1. Relative abundance of zooplankton in the coelenteron (± s.d.) of Dendronephthya hemprichi polyps, compared to the relative zooplankton availability (± s.d.) near a coral reef in Eilat, Israel (below). Data are based on 8,625 polyps from 30 field-collected colonies. Chesson’s (1978) index of prey preference, or , shows that D. hemprichi has a preference for bivalve and gastropod larvae (<700 µm body length), although the dominance of copepods in reef water results in highest carbon gain from this source. It is important to note that the total energy gained from zooplankton is only marginal. Data are based on Fabricius et al. (1995a).
Prey type | % in polyp coelenteron | % present in water column | Index of prey preference | Carbon gain (µg polyp-1) |
---|---|---|---|---|
Bivalves | 47.6±24.2 | 10.1±4.7 | 0.68 | 0.014 |
Gastropods | 15.3±7.3 | 9.0±4.0 | 0.25 | 0.011 |
Copepods | 34.4±18.7 | 68.9±6.9 | 0.07 | 0.028 |
Krill | 0 | 2.7±1.0 | 0 | 0 |
Tunicates | 0 | 2.1±1.9 | 0 | 0 |
Other | 2.6±3.2 | 7.2±3.6 | – | <0.001 |
Sum | 100 | 100 | 1.0 | 0.053 |
Table 2. Food availability in particles per liter and µg carbon per liter, and daily carbon gain per polyp per day (± s.e.) for Dendronephthya hemprichi (below). Phytoplankton is much more abundant than zooplankton, which is exploited by the efficient capture of small particles by D. hemprichi. As a result, carbon gain from phytoplankton is 50-200 times higher when compared to zooplankton. The higher the flow rate, the higher the carbon gain from phytoplankton, up to about 20 cm s-1. Data are based on Fabricius et al. (1995a).
Food type | Particles liter-1 | µg carbon liter-1 | Flow (cm s-1) | Carbon gain (µg polyp-1 day-1) |
---|---|---|---|---|
Phytoplankton | 1,000,000 | 15.0 | 4-6 | 9.0±4.8 |
6-8 | 15.0±4.1 | |||
8-10 | 41.0±11.4 | |||
Zooplankton | 1.1 | 3.5 | 1-18 | 0.2 |
Phyto:zoo carbon ratio | 4.3:1 | 50:1 – 200:1 |
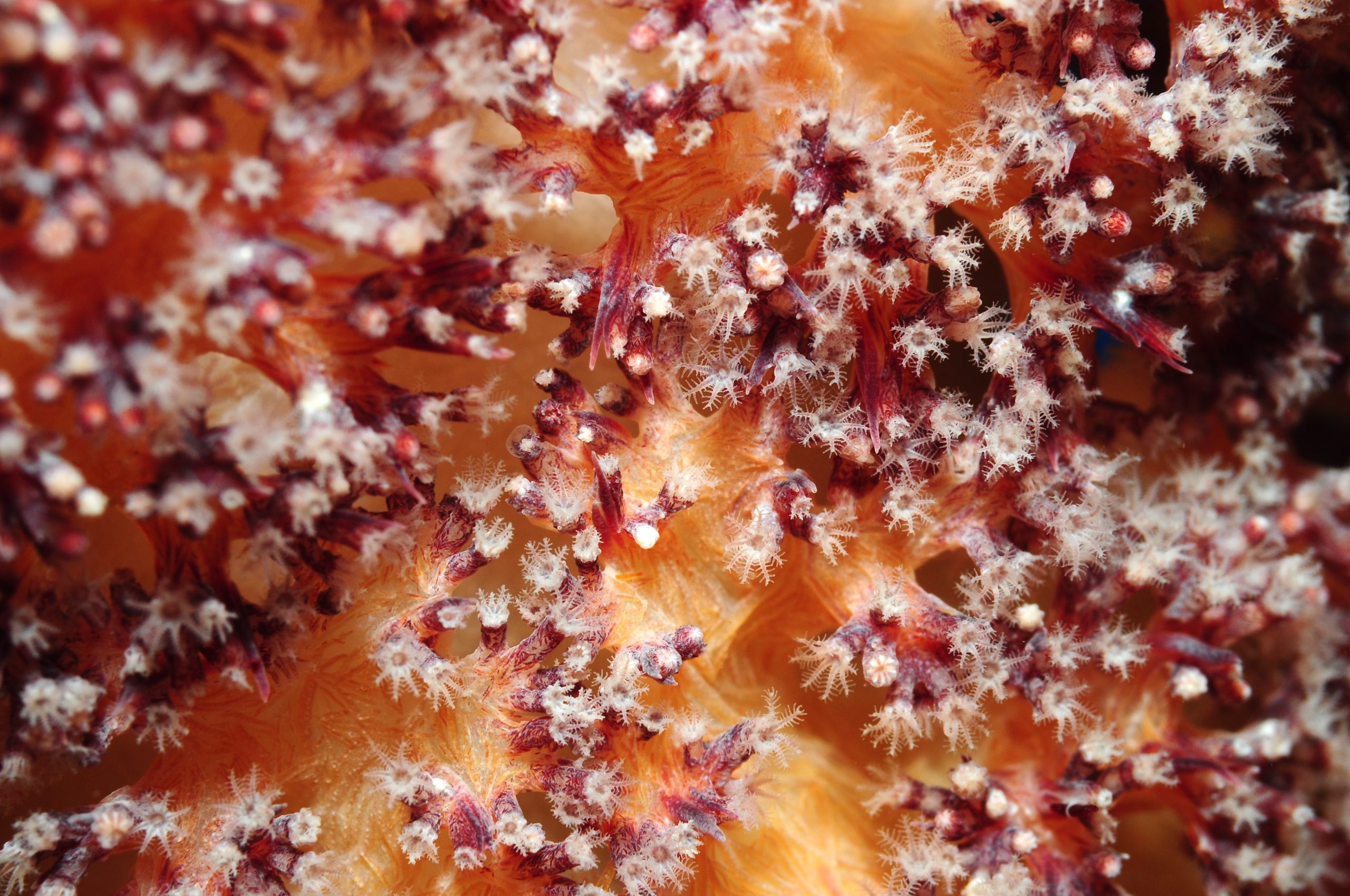
Dendronephthyapolyps have pinnulated tentacles, allowing them to sieve small plankton from the water.
Based on the assumption that both phytoplankton and zooplankton constitute important food sources for Dendronephthya, a feed mixture was prepared, containing Reed Mariculture’s Phyto Feast Live, Roti Feast and Oyster Feast, and freshly harvested Rhodomonas algae. Phyto Feast Live contains live green algae (Nannochloropsis and Tetraselmis), diatoms (Thalassiosira), haptophytes (Isochrysis and Pavlova) and cyanobacteria (Synechococcus), with a particle size range of 0.6-20 µm. As Nannochloropsis, Tetraselmis and Isochrysis have been confirmed to be consumed by Dendronephthya in the lab, this makes Phyto Feast Live an excellent candidate for feeding trials. Roti Feast contains L-type rotifers (Brachionus plicatilis) and their eggs, with a particle size range of 130-340 µm. This is also appropriate for Dendronephthya polyps, which mainly capture zooplankton less than 700 µm in size (Fabricius et al. 1995a). Oyster Feast contains oyster eggs and ovarian tissue, with 1-200 µm particle size, and may benefit all marine filter feeding organisms. The Rhodomonas cells are typically 16-30 µm in length.
This mixture was dosed in the aquarium from a refrigerator with an SP 3000 peristaltic pump (Aqua Medic). This peristaltic pump was connected to a timer, and was switched on briefly every three hours, or eight times per 24 hours. The table below summarizes the daily feeding quantities of experiment 1, which were dosed in eight batches each day.
Table 3. Daily feeding regime for experiment 1 (below). Feeds were dosed every three hours, or eight times per 24 hours. Over the course of two months, feeding quantities were increased. Phyto Feast Live contains live green algae (Nannochloropsis and Tetraselmis), diatoms (Thalassiosira), haptophytes (Isochrysis and Pavlova) and cyanobacteria (Synechococcus). Roti Feast contains L-type rotifers (Brachionus plicatilis) and their eggs. Oyster Feast contains oyster eggs and ovarian tissue. Quantity in grams / ounces was estimated by taking 9% as dry weight for Reed Mariculture’s products. The dry weight of the Rhodomonas culture was estimated at 0.08%, based on an average cell count of 8,000,000 cells per mL and a biomass of 100 pg per cell (Seixas et al. 2009). Natural plankton concentrations are based on Ayukai (1995), Heidelberg et al. (2004, 2010), Holzman et al. (2005), Yahel et al. (2005a,b) and Palardy et al. (2006).
Feed | Quantity (mL / fl oz.) | Quantity (g / oz.) dry weight | Final concentration per 3h batch (particles L-1) | Times reef concentration per 3h batch |
---|---|---|---|---|
Phyto Feast Live | 15-50 / 0.51-1.69 | 1.35-4.50/0.05-0.16 | 1,781,250-5,937,500 | 0.08-0.26 |
Roti Feast | 25-50 / 0.85-1.69 | 2.25-4.50/0.08-0.16 | 35-69 | 5.51-11.02 |
Oyster Feast | 1-10 / 0.03-0.34 | 0.09-0.90/0.003-0.03 | – | – |
Rhodomonas sp. | 200-1,000 / 6.76-33.81 | 0.16-0.80/0.006-0.03 | 142,857-714,286 | 0.14-0.71 |
Total | 241-1,110 / 8.15-37.53 | 3.85-10.70/0.14-0.38 | 1,924,142-6,651,855 | 5.73-12.00 |
For the second experiment, the same feeding mixture was used. However, based on the results of the first experiment, larger quantities were used. In addition, the mixture was always diluted with seawater to an end volume of approximately 1.2 liter, to allow larger volumes to be pumped in the aquarium every three hours. As the feed mixture that remains in the hose of the peristaltic pump fouls in between feedings, reducing the feed concentration results in less wasted plankton. In addition, the hose length was decreased considerably by placing the mixture in a box cooler, located on top of the aquarium, together with eight cooling packs. The cooling packs were replaced every day, and the temperature remained between 5 and 10°C (41-50°F) for 24 hours. The table below summarizes the daily feeding quantities of experiment 2, which were again dosed in eight batches every day.
Table 4. Daily feeding regime for experiment 2 (below). Feeds were dosed every three hours, or eight times per 24 hours. Over the course of one month, feeding quantities were increased. Phyto Feast Live contains live green algae (Nannochloropsis and Tetraselmis), diatoms (Thalassiosira), haptophytes (Isochrysis and Pavlova) and cyanobacteria (Synechococcus). Roti Feast contains L-type rotifers (Brachionus plicatilis) and their eggs. Oyster Feast contains oyster eggs and ovarian tissue, 1-200 µm particle size. Quantity in grams / ounces was estimated by taking 9% as dry weight for Reed Mariculture’s products. The dry weight of the Rhodomonas culture was estimated at 0.08%, based on an average cell count of 8,000,000 cells per mL and a biomass of 100 pg per cell (Seixas et al. 2009). Natural plankton concentrations are based on Ayukai (1995), Heidelberg et al. (2004, 2010), Holzman et al. (2005), Yahel et al. (2005a,b) and Palardy et al. (2006).
Feed | Quantity (mL / fl oz.) | Quantity (g / oz.) dry weight | Final concentration per 3h batch (particles L-1) | Times reef concentration per 3h batch |
---|---|---|---|---|
Phyto Feast Live | 25-100 / 0.85-3.38 | 2.25-9.00 / 0.08-0.32 | 2,968,750-11,875,000 | 0.13-0.52 |
Roti Feast | 25-100 / 0.85-3.38 | 2.25-9.00 / 0.08-0.32 | 35-139 | 5.51-22.05 |
Oyster Feast | 10-25 / 0.34-0.85 | 0.90-2.25 / 0.03-0.08 | – | – |
Rhodomonas sp. | 500-1,000 / 16.91-33.81 | 0.40-0.80 / 0.01-0.03 | 357,143-714,286 | 0.36-0.71 |
Total | 560-1,225 / 18.95-41.42 | 5.80-21.05 / 0.20-0.75 | 3,325,928-12,589,425 | 6.00-23.29 |

The laminar flow system used for the experiments. Two acrylic plates were placed at both ends of the aquarium, creating rounded corners which prevent turbulent water flow.
Flow
Flow is another important aspect of Dendronephthya husbandry. Fabricius and colleagues (1995a) found that Dendronephthya hemprichi feeds and grows optimally within a flow range of 10 to 25 cm s-1. Lower flow speeds result in reduced particle encounter rates, and thus reduced feeding and growth. Higher flow rates cause colonies and polyps to deform due to strong drag forces, resulting in less efficient filter feeding. This bell-shaped relationship between water flow rate and feeding rates has been found for many coral species (Wijgerde et al. 2012, Wijgerde 2013).
Based on the needs of Dendronephthya, a culture system was designed in which laminar water flow could be created. An aquarium was constructed with the dimensions 200 x 100 x 70 cm, or 80 x 40 x 28 inches (length x width x height), and a total volume of 1,400 liters or 370 US gallons.
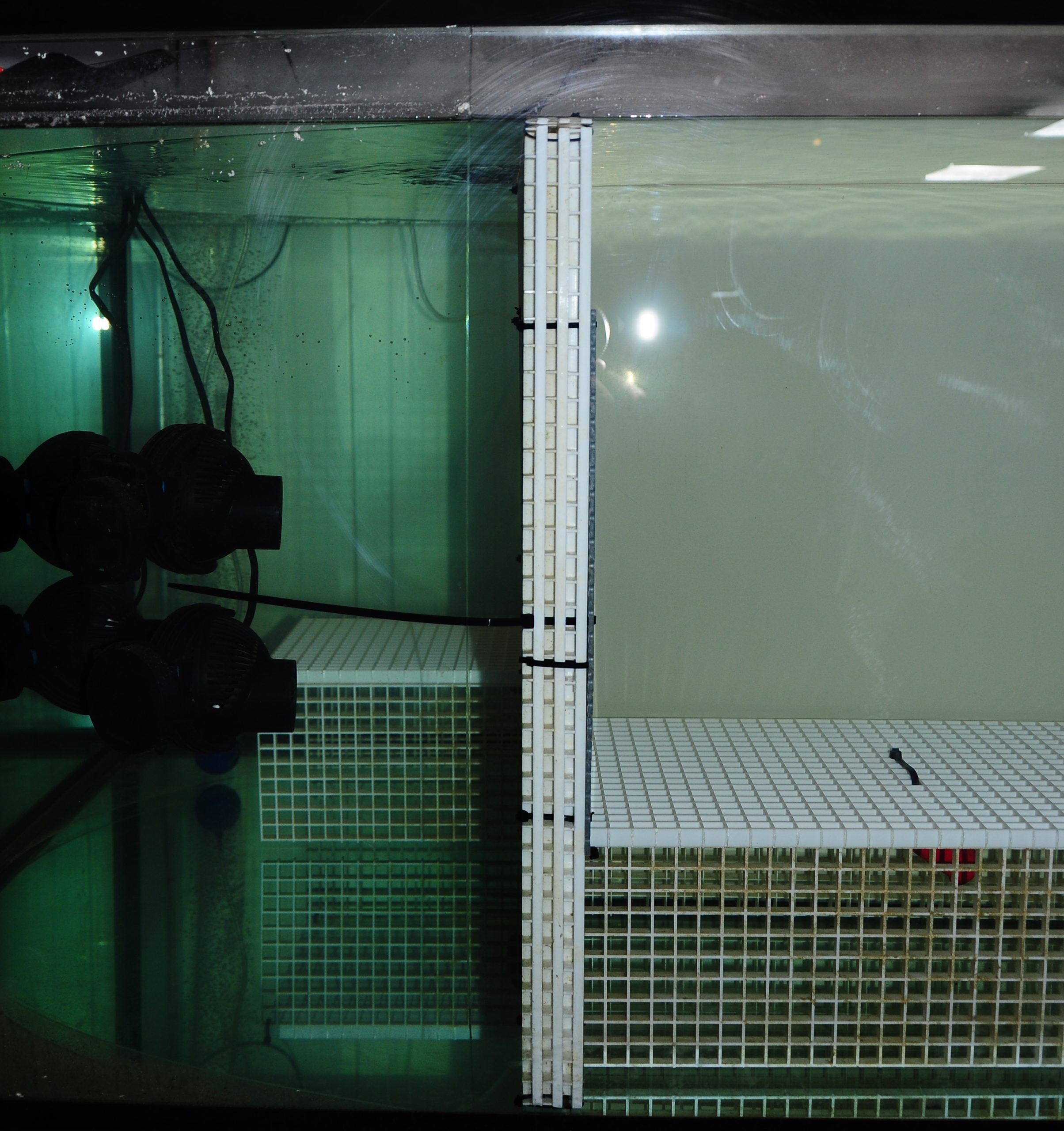
Laminar flow was created with eight tunze 6085 circulation pumps, which forced water through flow laminators positioned on two diagonally opposite sides of the aquarium. This resulted in many small water jets emanating from the plates.
Water flow was provided by eight tunze Turbelle Stream 6085 circulation pumps, with a total capacity of 64,000 liters per hour (16,842 US gallons per hour). The pumps were placed in two groups of four, on diagonally opposite sides of the aquarium. The flow produced by the Turbelle pumps was restricted by two flow laminators, which were positioned directly in front of the pumps. Each flow laminator consisted of three egg crates that were cut into approximate squares and tied together with cable ties, with a perforated PVC plate having 6 mm holes mounted at the front side. The Turbelle pumps produced many small water jets which emanated from the flow laminators. Addition of salt to the aquarium revealed that flow patterns over the coral tables were quite laminar (see video below). Flow rates were measured in different areas over the coral tables, and varied between 14 and 33 cm s-1. Flow rates directly in front of the corals which were closest to the pumps were 21-33 cm s-1. For the corals in the middle of the table, flow rates were 20-23 cm s-1. For those furthest away from the pumps, flow rates were 14-16 cm s-1.
https://youtube.com/watch?v=KijVubLbfuM
Video showing the laminar flow setup.
Filtration
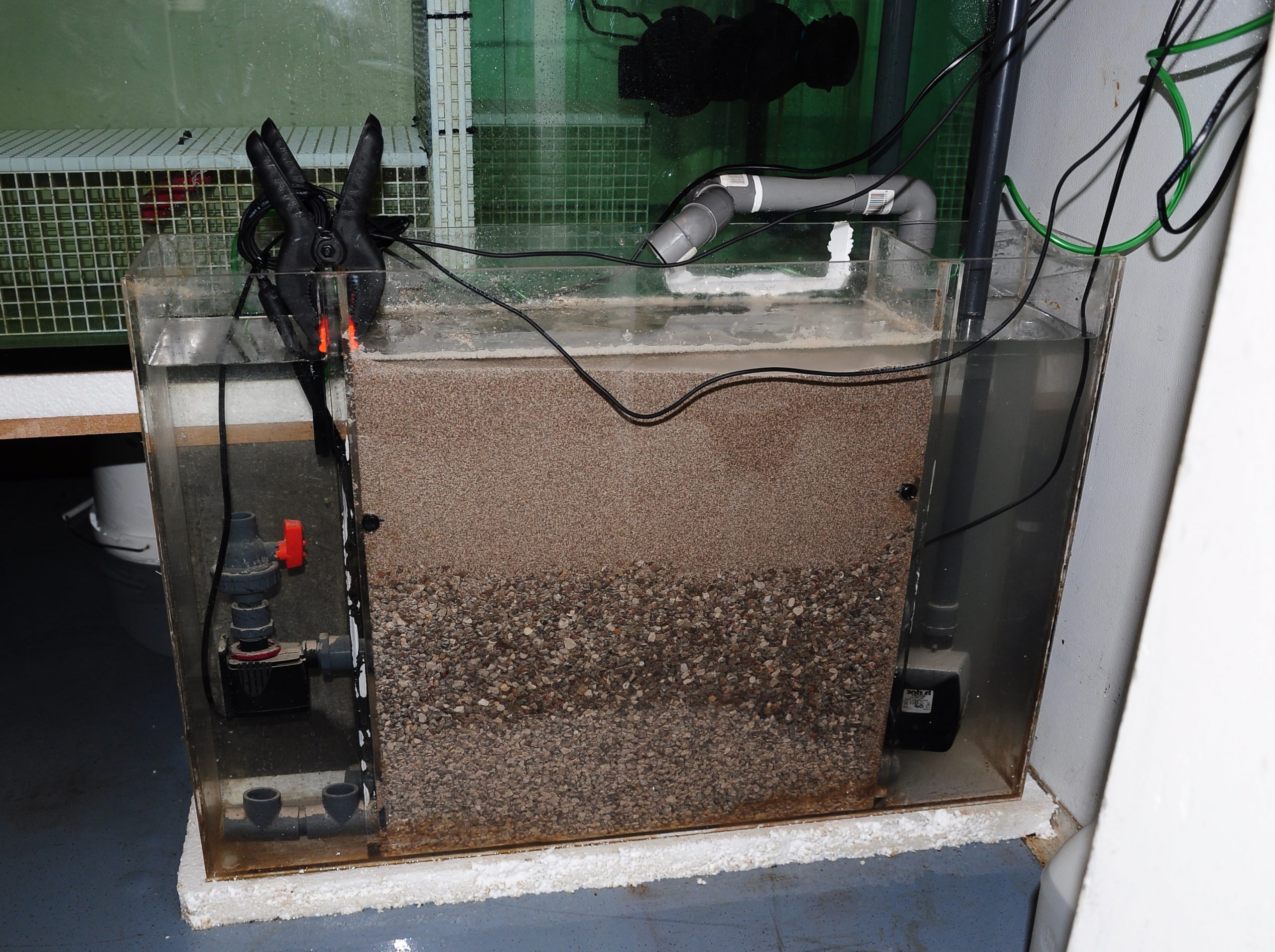
The custom-built DyMiCo (Dynamic Mineral Control) filter that was used to maintain water quality. This filter processes inorganic nitrogen and functions as a calcium reactor.
As aquarists know, food availability and filtration in aquaria are two intertwined topics. Conventional filtration devices such as protein skimmers, fluidized reactors and biofilters are known to trap fine particulates, which is problematic if one aims to maintain high plankton concentrations in aquarium water.
To work around this problem, I built a small version of a DyMiCo (Dynamic Mineral Control) filter and installed it on the aquarium. DyMiCo basically works as a deep sand bed or Jaubert system, in which nitrification and denitrification occur in different layers. The major difference is that an IKS Aquastar constantly measures the redox value of the hypoxic lower layer of the sand bed, and controls three pumps to maintain a relatively stable value between -300 and -100 mV, which results in high denitrification rates. At higher redox values, less water is drawn through the sand bed with the return pump, and more organic carbon is pumped into the filter to stimulate oxygen consumption by bacteria. At lower redox values, the opposite occurs. A third pump constantly mixes the water within the lower layer of the sand bed, to ensure that carbon is evenly dispersed and redox measurements are stable. The constantly low redox value and continued availability of carbon allows heterotrophic bacteria to convert nitrate into nitrogen gas, which is ultimately pumped into the aquarium and removed by water aeration. What makes this system plankton-saving is that it filters the entire system only about once a day. This is sufficient to prevent a build-up of nitrate and phosphate, while at the same time allowing more plankton and artificial feed to remain in the water. Carbon dioxide can also be injected into the sand bed, controlled by the same Aquastar and a pH probe, allowing DyMiCo to function as a powerful calcium reactor. Since I only had a few soft corals in the aquarium, I did not opt for this.
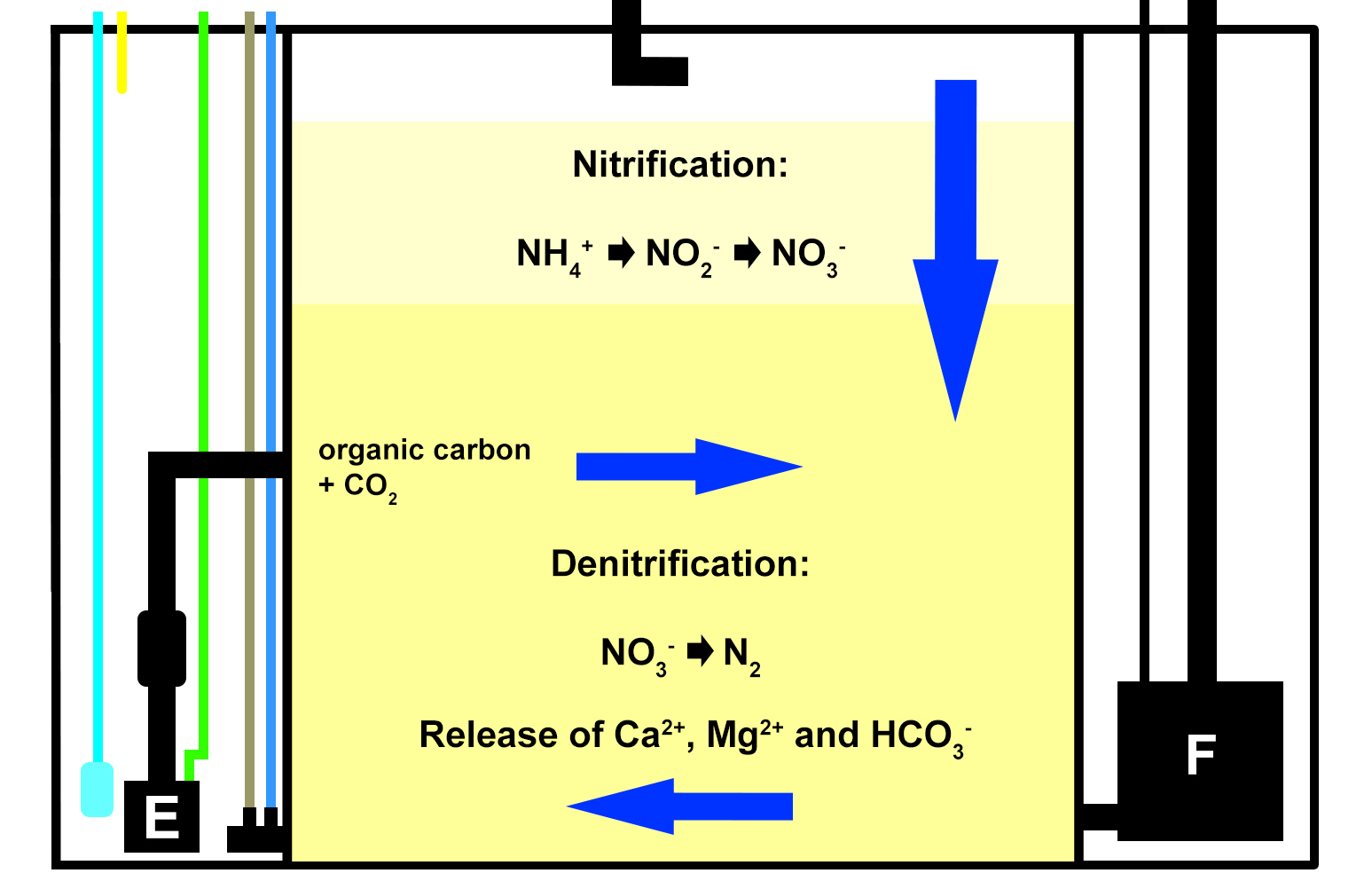
A schematic overview of how DyMiCo works. Blue arrows represent water flow through the sand bed, with vertical flow when the return pump (F) is active, and horizontal flow when the mixing pump (E) is active. The cyan line indicates the CO2 supply (not used for this experiment), the yellow line indicates the organic carbon supply. The brown line represents the pH probe, and the blue line depicts the redox probe, which measure the interstitial water of the sand bed.
Although the filter worked just fine, I used an old acrylic box for the sand bed and pumps, as my budget was limited and I had to improvise. This box was not intended to operate outside water, and it started to bulge and leak after several months. I eventually had to remove it, and switched to regular water changes (see water quality).
To maintain stable pH and oxygen saturation, the main aquarium was constantly aerated with a large air stone. Artificial seawater was prepared using reverse osmosis water (conductivity < 10 µs cm-2) and Tropic Marin PRO-REEF sea salt.
Corals
For the first preliminary experiment, ten Dendronephthya specimens (family Nephtheidae) were acquired via De Jong Marinelife, The Netherlands. I also purchased another specimen that may be a Chironephthya sp. (family Nidaliidae) or Chromonephthea sp. (family Nephtheidae). At this moment, it is impossible to properly identify the various Dendronephthya species used for the experiments, as the genus requires complete revision (van Ofwegen 2015). However, based on morphological differences, it is quite likely that different species were present.
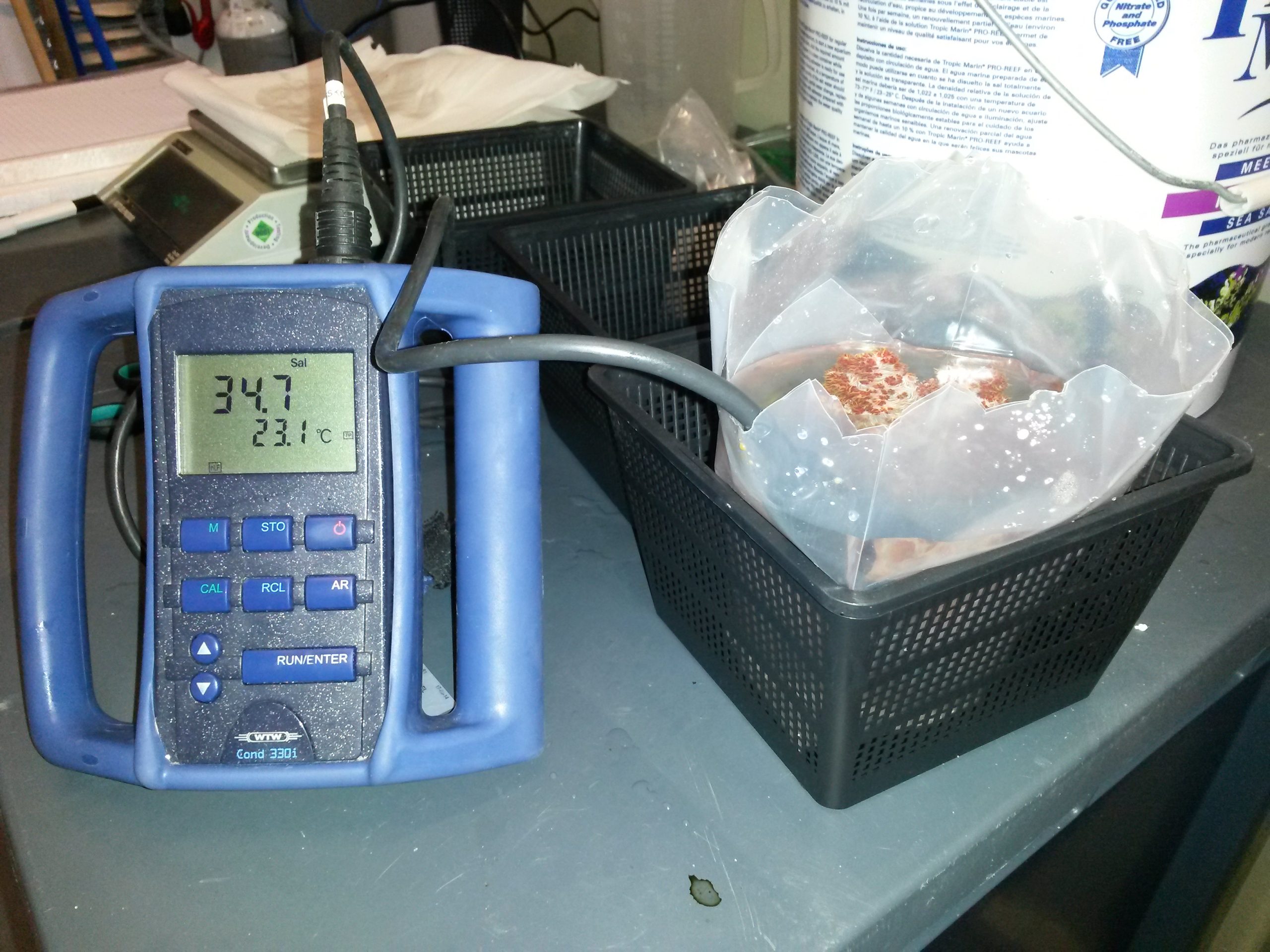
Temperature and salinity were measured upon arrival. All corals were slowly acclimatized to prevent sudden changes in water chemistry.
Before introduction in the aquarium, all corals were weighed above water while still attached to their fragmentation rocks. If the corals had grown, based on visual observation, the intention was to weigh them again after several months to determine weight increases. By removing the corals from their rocks at the end of the experiment, the net starting and final weights could have been calculated. This would allow for calculation of the relative, or specific growth rates. However, since the corals degenerated and did not respond well to air exposure, this method was abandoned during the second experiment.
During experiment 1, all corals were fixed onto horizontal egg crate tables using cable ties, at an approximate height of 20 centimeters from the aquarium bottom. All colonies were positioned perpendicularly to the water flow, allowing for maximal particle capture.
For the second experiment, only five colonies were purchased due to limited availability. Multiple species were most likely used, which presently cannot be identified. During this experiment, all corals were fixed to the vertical surface of the tables, to determine whether this would impact expansion, health and growth. Field observations demonstrate that vertically growing Dendronephthya exhibit much higher survival rates, possibly due to reduced sedimentation (Dahan and Benayahu 1997). The same could be true in an aquarium which is fed heavily, with detritus formation and sedimentation as a result. In addition, Delbeek (2002) observed that these corals may get irritated when touching the bottom of the aquarium, which is especially problematic when the corals are deflated and lying down on their tables.
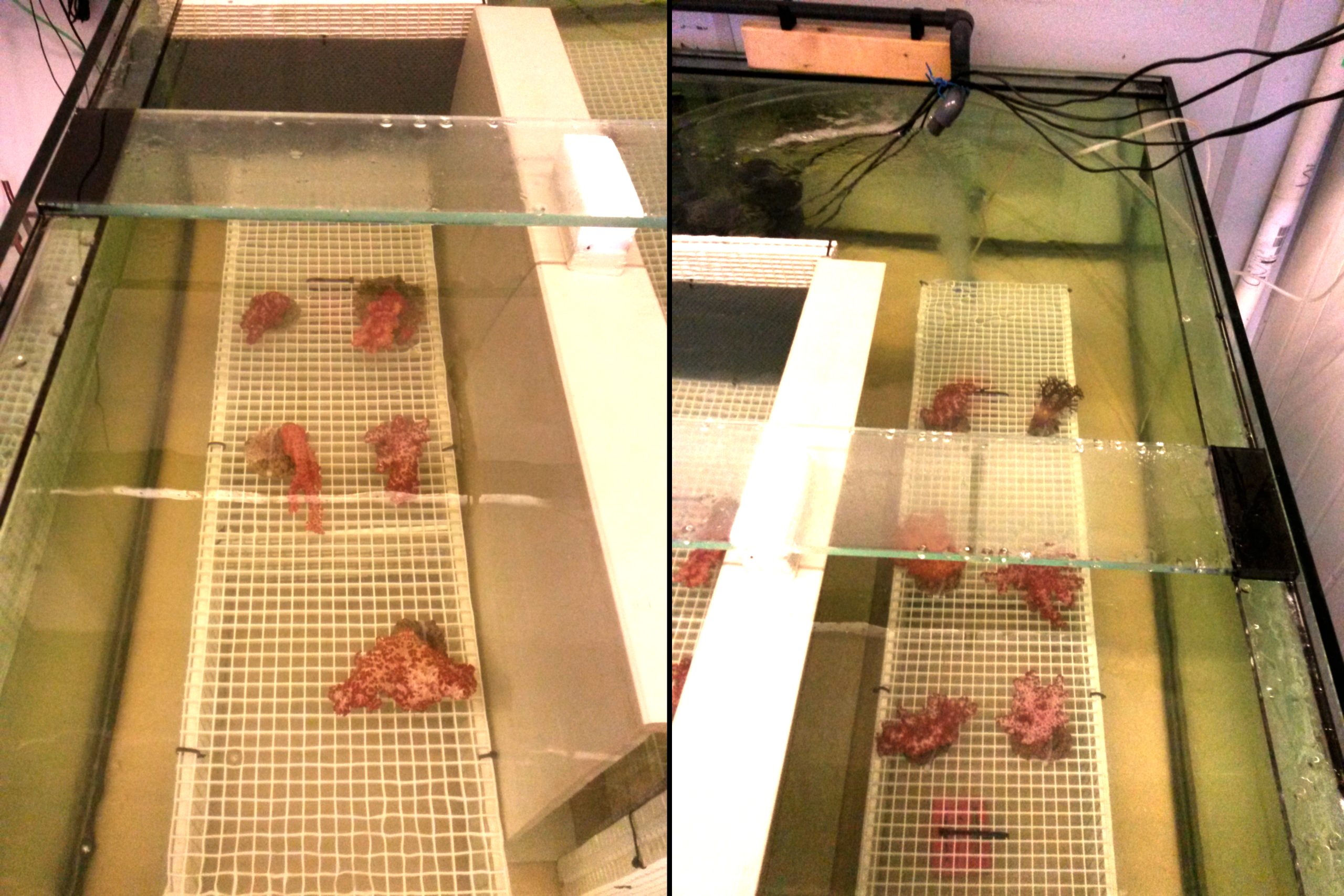
During the first experiment, all corals were placed on top of the tables, oriented perpendicularly to the water current. Photographs show left and right sides of the aquarium.
Water quality was fairly stable over the course of both experiments, even though no water changes were done. When the DyMiCo filter was removed at the 18th of February 2015, and weekly water changes were done, changes occurred. Salinity decreased from 35.6 to 32.7 g L-1 (ppt) over a period of five weeks, as the source water used for changes (a large DyMiCo system) had lower salinity. The oxygen concentration increased from 6.5 to 7.3 mg L-1, partly because water temperature decreased, elevating oxygen solubility. Nutrients increased significantly, especially nitrate-nitrogen, which increased fivefold despite 50% weekly water changes. In the last few weeks, 75% weekly water changes resulted in intermediate nitrate-nitrogen levels. This shows how effective DyMiCo removed nitrate during the first few months. The elevated nitrate and phosphate concentrations did not seem to negatively affect colony expansion. However, during the last few weeks, a 75% water change resulted in increased expansion of all colonies within 30 minutes. Whether this sudden increased expansion occurred due to decreased nutrient levels, other water chemistry changes, or the addition of specific types of plankton is unclear.
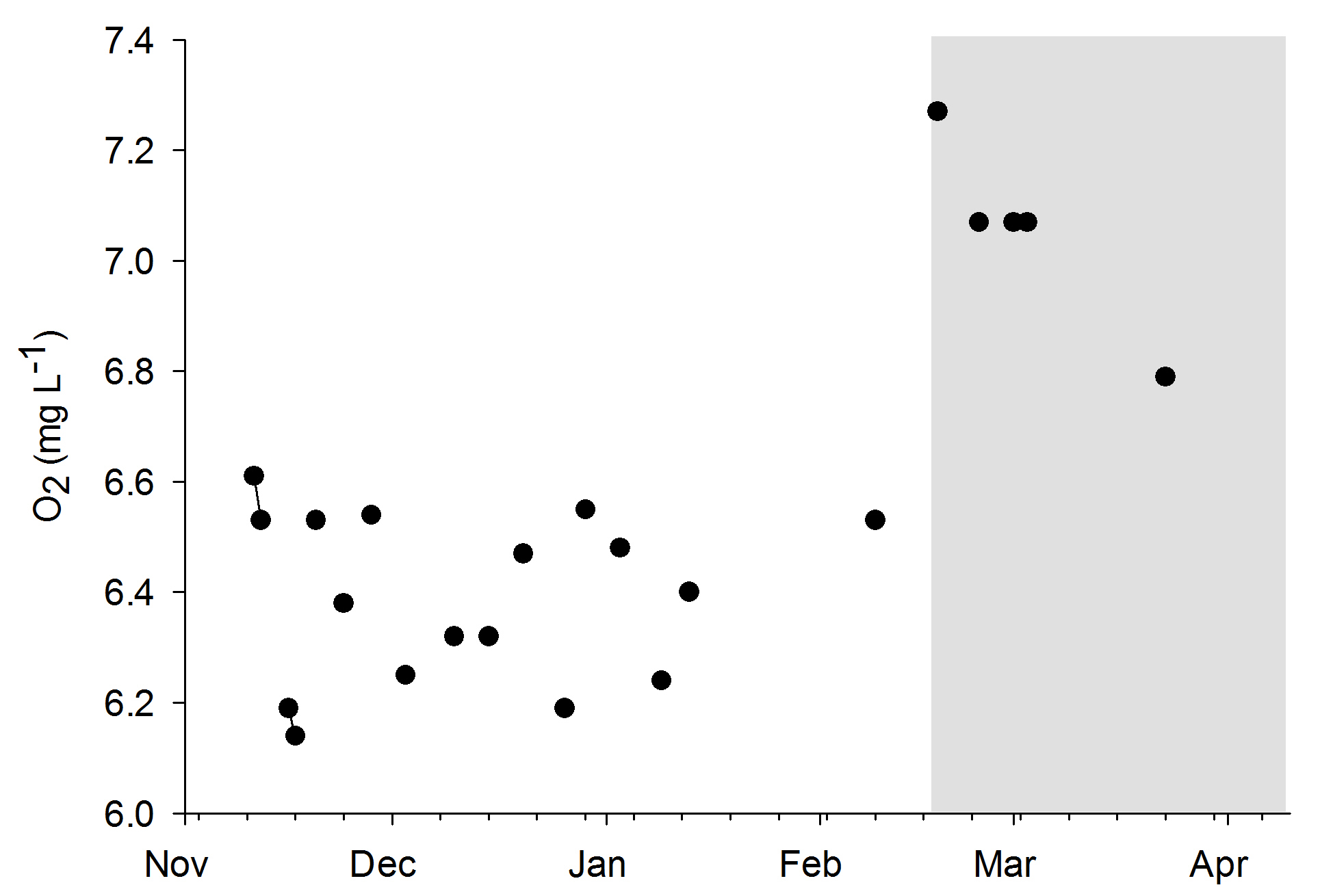
Oxygen levels during the experiments. The grey area indicates the removal of the DyMiCo filter. During this last period, 50-75% water changes were done every week.
Water quality was fairly stable over the course of both experiments, even though no water changes were done. When the DyMiCo filter was removed at the 18th of February 2015, and weekly water changes were done, changes occurred. Salinity decreased from 35.6 to 32.7 g L-1 (ppt) over a period of five weeks, as the source water used for changes (a large DyMiCo system) had lower salinity. The oxygen concentration increased from 6.5 to 7.3 mg L-1, partly because water temperature decreased, elevating oxygen solubility. Nutrients increased significantly, especially nitrate-nitrogen, which increased fivefold despite 50% weekly water changes. In the last few weeks, 75% weekly water changes resulted in intermediate nitrate-nitrogen levels. This shows how effective DyMiCo removed nitrate during the first few months. The elevated nitrate and phosphate concentrations did not seem to negatively affect colony expansion. However, during the last few weeks, a 75% water change resulted in increased expansion of all colonies within 30 minutes. Whether this sudden increased expansion occurred due to decreased nutrient levels, other water chemistry changes, or the addition of specific types of plankton is unclear.
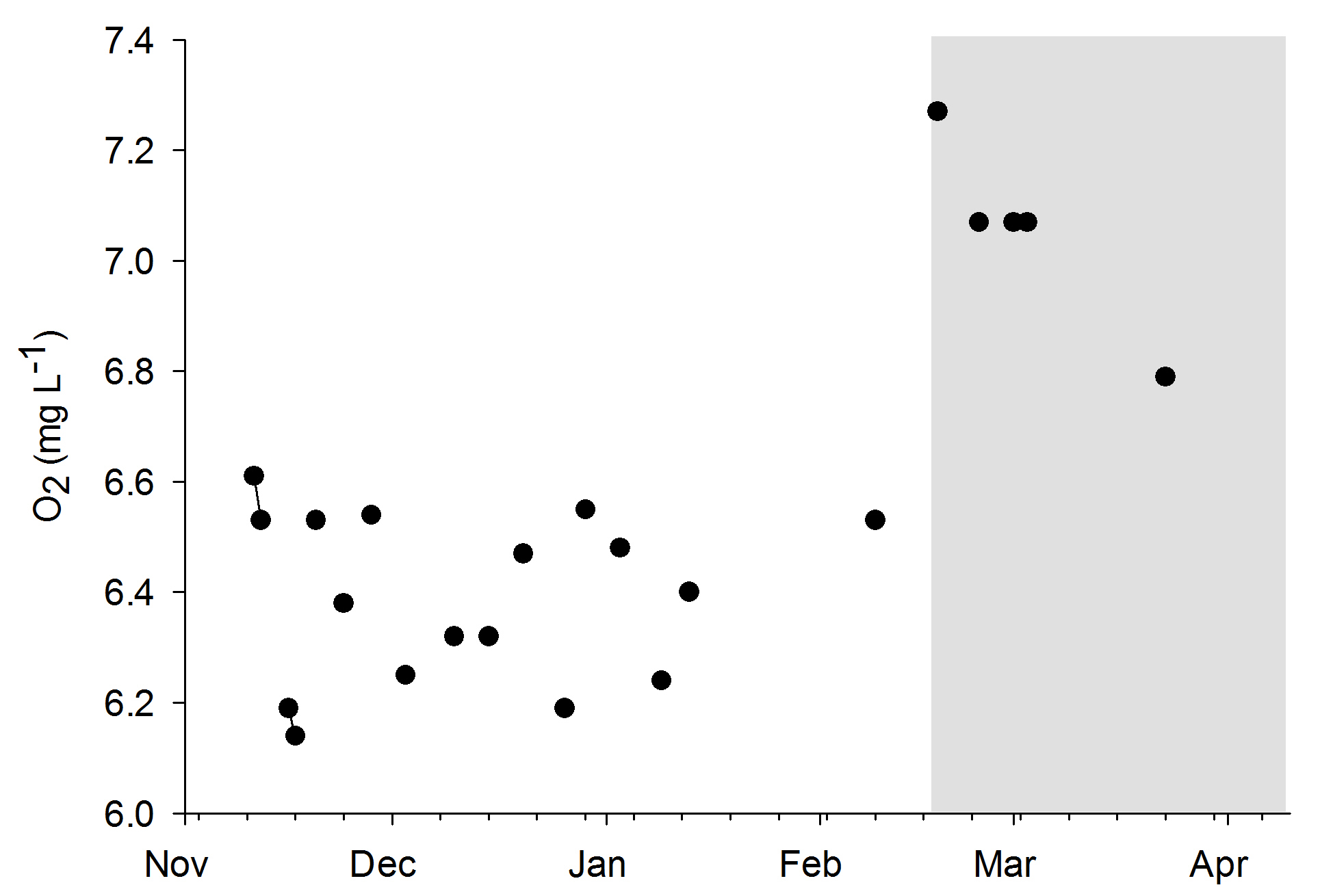
Oxygen levels during the experiments. The grey area indicates the removal of the DyMiCo filter. During this last period, 50-75% water changes were done every week.
Preliminary findings and discussion
Water quality
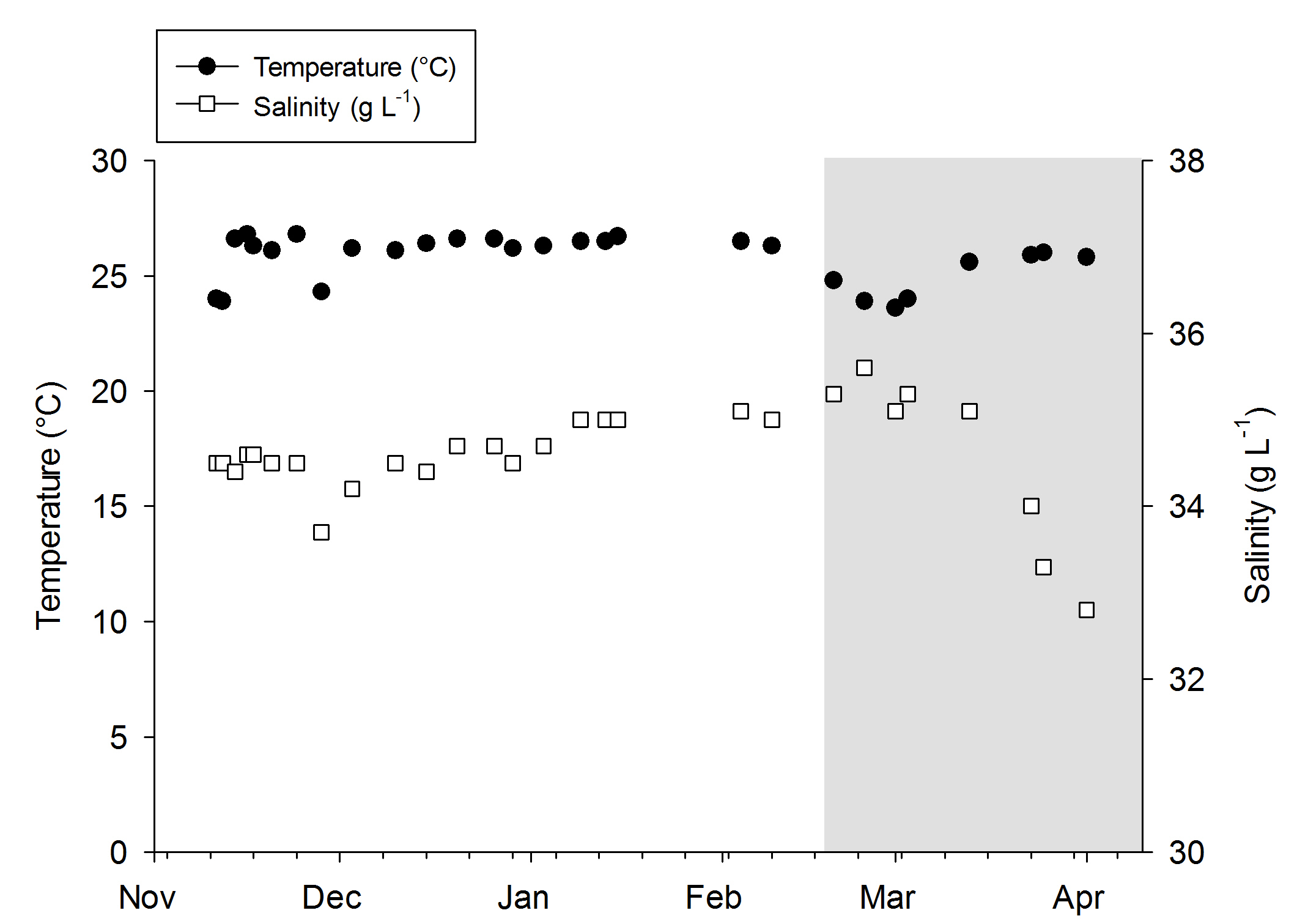
Temperature and salinity values during the experiments. The grey area indicates the removal of the DyMiCo filter. During this last period, 50-75% water changes were done every week.
Water quality was fairly stable over the course of both experiments, even though no water changes were done. When the DyMiCo filter was removed at the 18th of February 2015, and weekly water changes were done, changes occurred. Salinity decreased from 35.6 to 32.7 g L-1 (ppt) over a period of five weeks, as the source water used for changes (a large DyMiCo system) had lower salinity. The oxygen concentration increased from 6.5 to 7.3 mg L-1, partly because water temperature decreased, elevating oxygen solubility. Nutrients increased significantly, especially nitrate-nitrogen, which increased fivefold despite 50% weekly water changes. In the last few weeks, 75% weekly water changes resulted in intermediate nitrate-nitrogen levels. This shows how effective DyMiCo removed nitrate during the first few months. The elevated nitrate and phosphate concentrations did not seem to negatively affect colony expansion. However, during the last few weeks, a 75% water change resulted in increased expansion of all colonies within 30 minutes. Whether this sudden increased expansion occurred due to decreased nutrient levels, other water chemistry changes, or the addition of specific types of plankton is unclear.
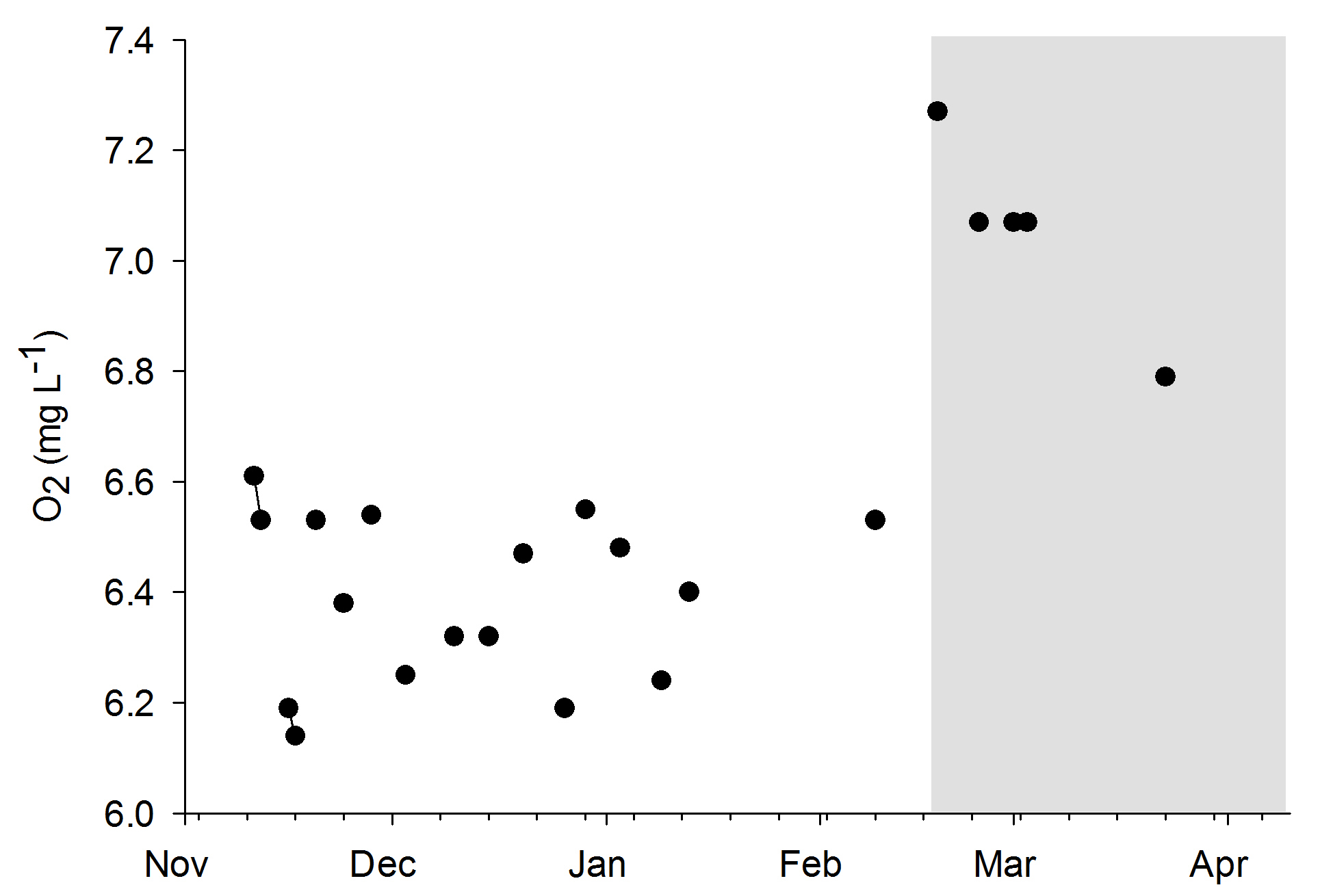
Oxygen levels during the experiments. The grey area indicates the removal of the DyMiCo filter. During this last period, 50-75% water changes were done every week.
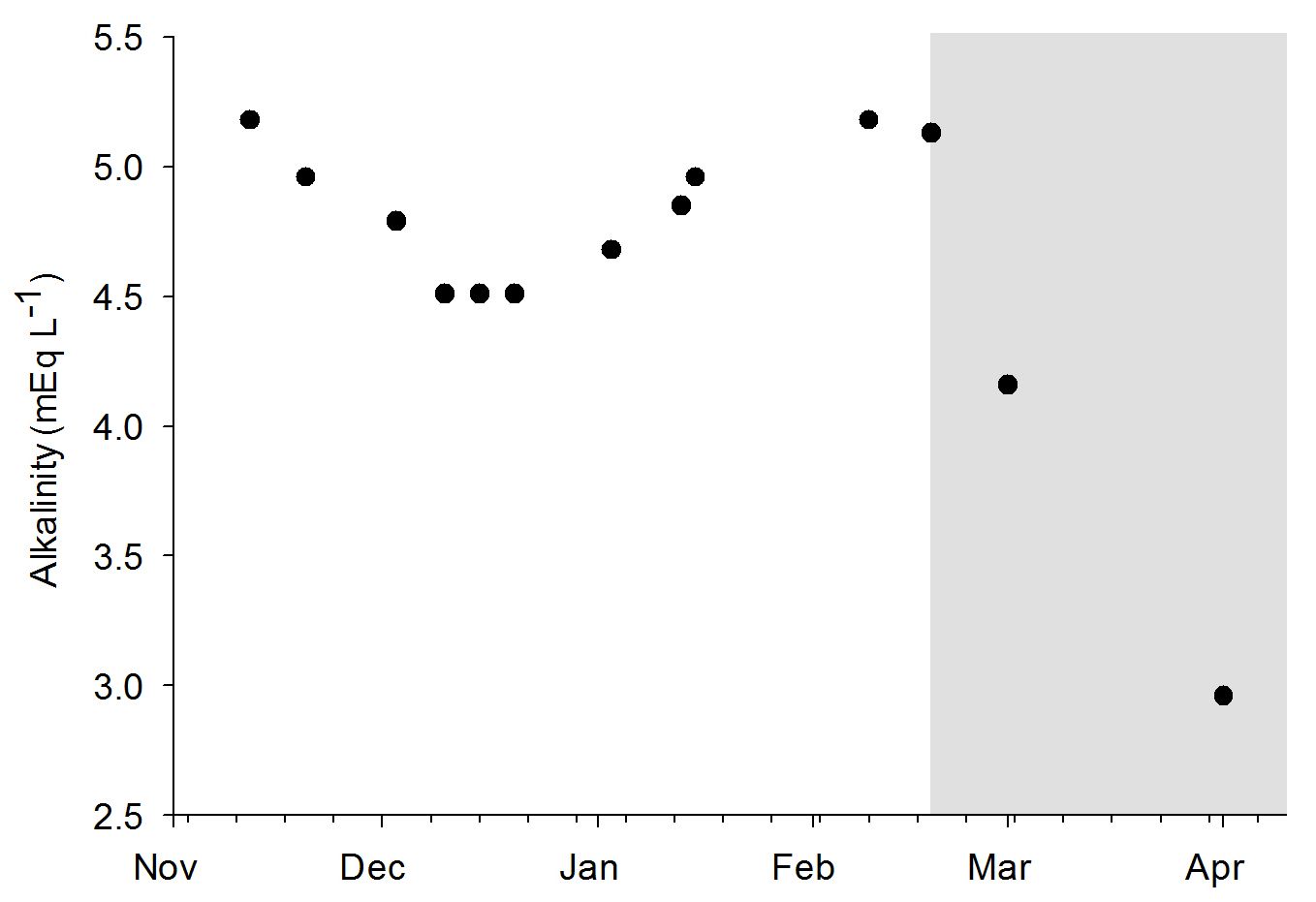
Alkalinity values during the experiments. The grey area indicates the removal of the DyMiCo filter. During this last period, 50-75% water changes were done every week.
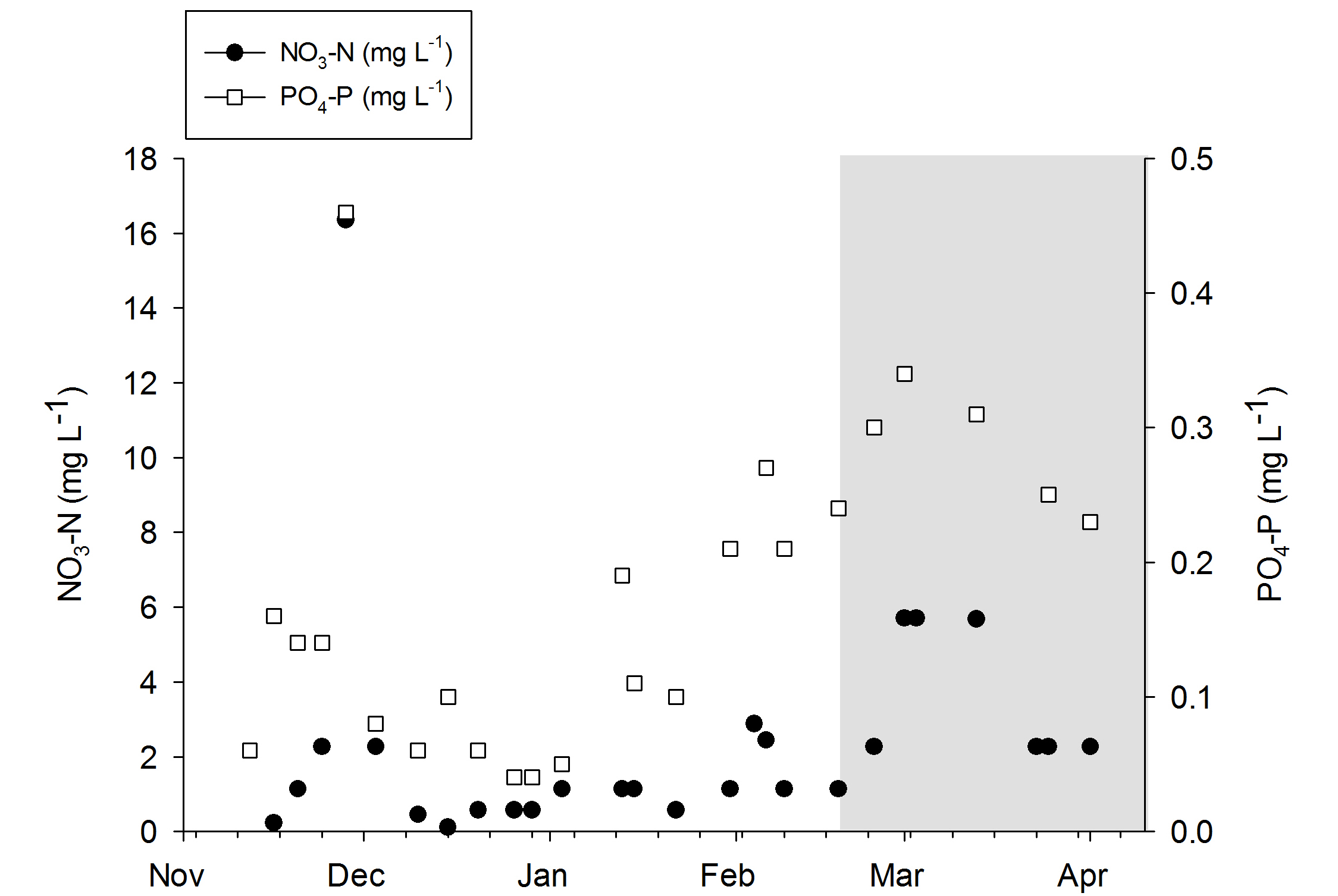
Nitrate-N and phosphorus-P values during the experiments. The grey area indicates the removal of the DyMiCo filter. During this last period, 50-75% water changes were done every week.
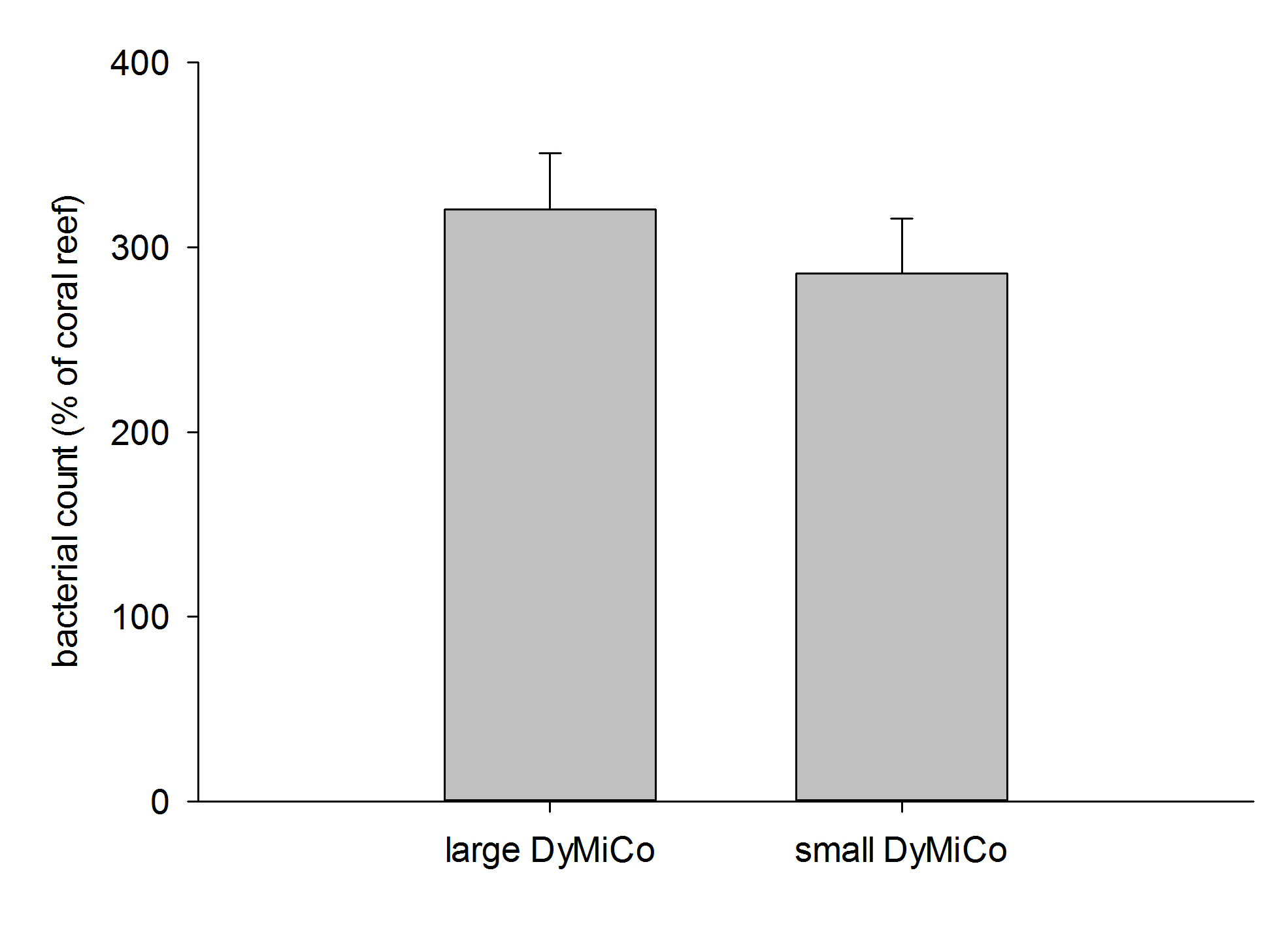
Heterotrophic bacterial counts in both DyMiCo systems relative to a typical coral reef (800,000 bacteria mL-1). Data are means + s.d. (N=10 counts).
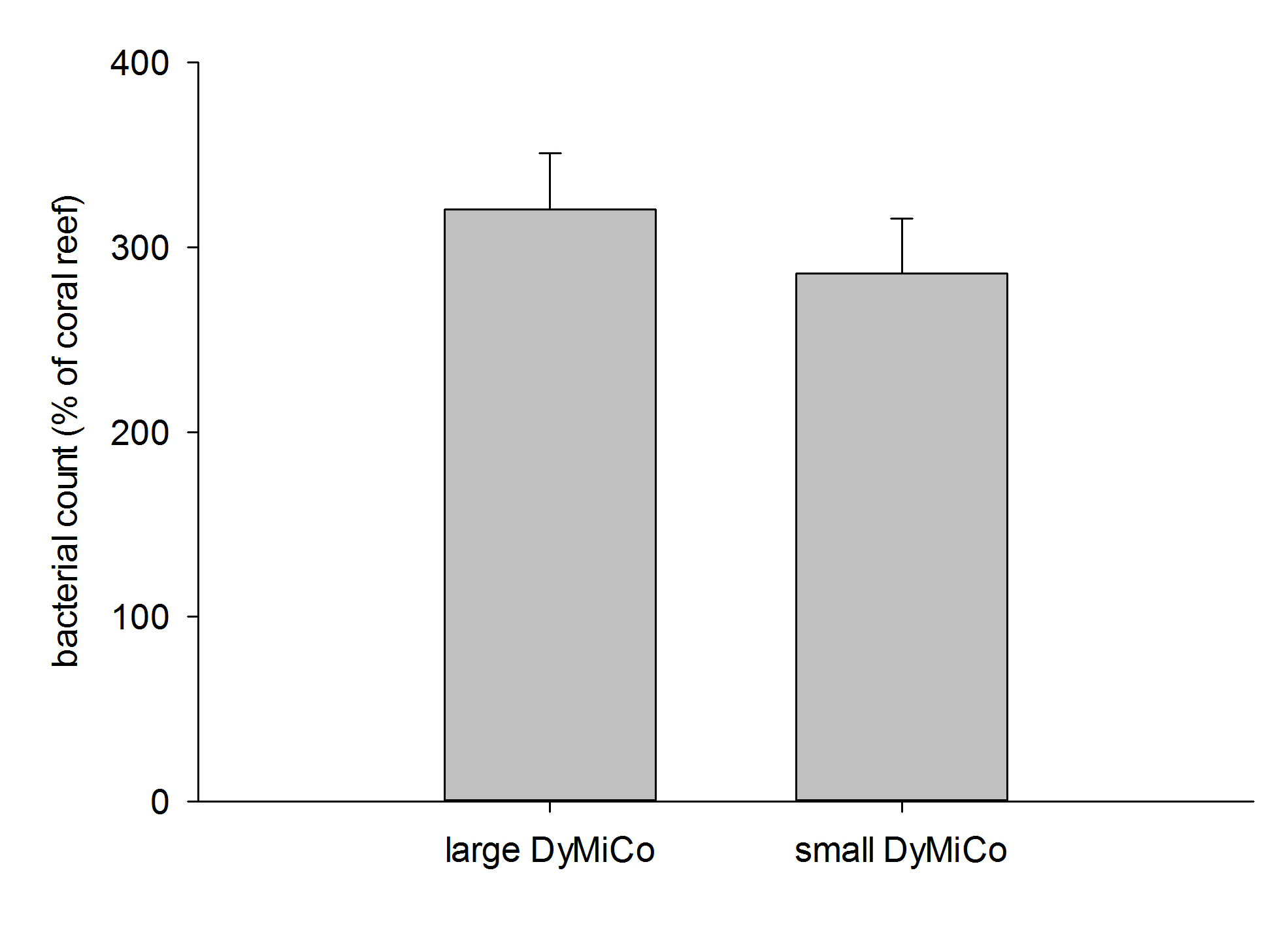
Heterotrophic bacterial counts in both DyMiCo systems relative to a typical coral reef (800,000 bacteria mL-1). Data are means + s.d. (N=10 counts).
Time-lapse video showing the expansion and contraction behavior of Dendronephthya. Time compression is 100-fold, with approximately 12 hours of footage condensed to about 7 minutes. Interestingly, although each colony seems to have its own rhythm, the total time spent in a given state is comparable between corals.
Field observations indicate these corals expand about 15 hours per day, with expansion predominantly occurring during the night (Fabricius 2014). During daytime, these corals expand as well, but possibly less than at night. During experiment 1, the nocturnal expansion and contraction behavior of three corals was recorded with a camera having night vision capability (Sony HDR-CX505), together with a low power aquarium LED light.
Coral health and growth – Experiment 1
Time-lapse video showing the expansion and contraction behavior of Dendronephthya. Time compression is 100-fold, with approximately 12 hours of footage condensed to about 7 minutes. Interestingly, although each colony seems to have its own rhythm, the total time spent in a given state is comparable between corals.
During the first week, coral expansion was limited. Most colonies showed poor hydrostatic pressure and lied flat on the tables. It is known that Dendronephthya spp. are sensitive to air exposure (Fabricius 2014), which is in line with their limited presence in the intertidal zone (Fabricius and Alderslade 2001).
Coral health slowly declined over time. After four weeks, a decrease in polyp density was clearly visible. This was followed by reduced inflation, giving them an emaciated, withered appearance. Without sufficient polyps to draw in water via ciliary currents, soft corals most likely cannot maintain hydrostatic pressure, rendering the colonies unable to stay in an upright position. Reduced polyp count will also result in less effective filter feeding. It is possible that after several weeks, these corals reach a point of no return, after which increased feeding has no beneficial effect anymore.
Two colonies seemed to fare better, and one even showed signs of growth at the branch tips. However, after about three months, even these corals completely degenerated.
During this experiment, two snails known as false cowries were found on two different coral colonies. At least one of these was Diminovula culmen (van Ofwegen 2015), and it clearly left grazing marks on its host coral. It also regularly produced egg clusters. Whether these hatched successfully is unclear, as only one additional cowrie was found. Both snails were removed and preserved in alcohol for future determination.
During this experiment, two snails known as false cowries were found on two different coral colonies. At least one of these was Diminovula culmen (van Ofwegen 2015), and it clearly left grazing marks on its host coral. It also regularly produced egg clusters. Whether these hatched successfully is unclear, as only one additional cowrie was found. Both snails were removed and preserved in alcohol for future determination.
During this experiment, two snails known as false cowries were found on two different coral colonies. At least one of these was Diminovula culmen (van Ofwegen 2015), and it clearly left grazing marks on its host coral. It also regularly produced egg clusters. Whether these hatched successfully is unclear, as only one additional cowrie was found. Both snails were removed and preserved in alcohol for future determination.
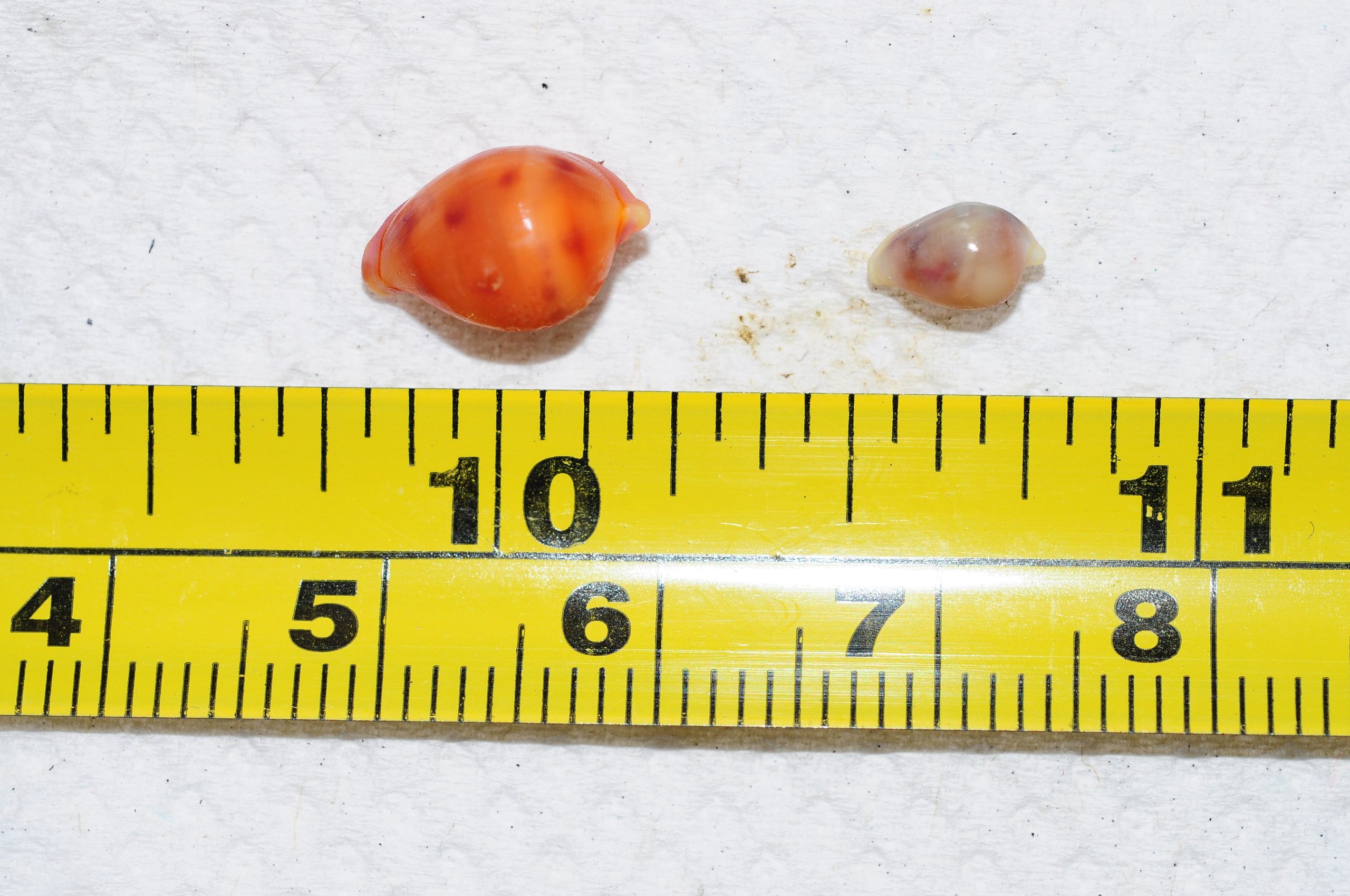
Two false cowries that were found in the aquarium. The shells are visible as the mantle no longer covers it when the snails are exposed to air. The specimen on the right is Diminovula culmen, the one on the left remains unidentified, but possibly is D. culmen as well. Scale bars are in inches (top) and centimeters (bottom).
Video of a Dendronephthya colony with a false cowrie (Diminovula culmen) and egg mass.
What determines success with Dendronephthya?
It is puzzling to see most corals degenerate within three months, despite the significant amount of high quality plankton that was continuously dosed in the aquarium. For example, during the course of experiment 2, over 21 grams of dry weight equivalent was fed every day, quite a large amount. In addition, the density of heterotrophic bacteria in the water column was high, adding to plankton availability. Water quality was also relatively high, with nitrate and phosphate levels remaining within an acceptable range for marine life.
Previously, some aquarists have reported medium-term successes with Dendronephthya (Matthews 2008), keeping them alive with some growth for over a year. There are several possible explanations for why the current experiments failed to produce healthy and fast-growing corals. At this point in time, however, the explanations below remain mere speculation.
Dendronephthyaand Scleronephthya spp. produce calcium carbonate sclerites, providing colony rigidity and resistance to strong water currents. This allows these corals to withstand strong water currents. Photograph by Bart Stanczyk, www.reefhub.pl.
The first possible explanation for the observed coral deterioration is the lack of a natural food web in the experimental aquarium. In order to provide basic, controlled conditions, I set up an aquarium with known water parameters, water flow rate, feeding quantities and amount of coral biomass. By not introducing other marine life, save for that which was present on the small pieces of live rock to which the corals were attached, I tried to keep it “sterile”. It is possible that without the presence of fishes, or other marine life, excretion products are missing that in nature fulfill essential links in the food chain. Perhaps the system was deficient in specific types of DOM (dissolved organic matter), such as urea or amino acids, compounds which are known to be excreted by fish and extracted from the water by corals (Grover et al. 2006, 2008). Although these substances are present on reefs in minute concentrations only, they constitute a significant source of organic nitrogen for corals. It is also known that sponges form a key link in the coral reef food web, recycling dissolved organic matter and converting it to sponge biomass (De Goeij et al. 2013). By constantly shedding filter cells (choanocytes), sponges provide reef life with a constant supply of detrital food. Perhaps a more complete reef assemblage of corals, sponges, fishes and other marine life is required for Dendronephthya to thrive.
Another possible explanation for the lack of success is a plankton deficiency, although tables 3 and 4 indicate that sufficient particles were present during the experiments. In terms of algae and bacteria alone (Phyto Feast Live together with Rhodomonas), an approximate natural plankton concentration was established every three hours, at least during the second half of both experiments. Together with Roti Feast and Oyster Feast, one would expect that sufficient food items were available to the corals. Although these corals can quickly deplete the water of plankton (Fabricius et al. 1995a,b), this was corrected eight times per day by pumping fresh plankton into the aquarium. It is possible that higher food additions would have resulted in more favorable results. This remains to be determined, however.
What determines success with Dendronephthya?
It is puzzling to see most corals degenerate within three months, despite the significant amount of high quality plankton that was continuously dosed in the aquarium. For example, during the course of experiment 2, over 21 grams of dry weight equivalent was fed every day, quite a large amount. In addition, the density of heterotrophic bacteria in the water column was high, adding to plankton availability. Water quality was also relatively high, with nitrate and phosphate levels remaining within an acceptable range for marine life.
Previously, some aquarists have reported medium-term successes with Dendronephthya (Matthews 2008), keeping them alive with some growth for over a year. There are several possible explanations for why the current experiments failed to produce healthy and fast-growing corals. At this point in time, however, the explanations below remain mere speculation.
Dendronephthyaand Scleronephthya spp. produce calcium carbonate sclerites, providing colony rigidity and resistance to strong water currents. This allows these corals to withstand strong water currents. Photograph by Bart Stanczyk, www.reefhub.pl.
Despite the disappointing results of the first experiment, about a month after introduction of the first batch of corals, asexual reproduction was visible. Several small colonies, known as recruits, were found at the base of several colonies. The continuous release of clonal fragments is a natural process for Dendronephthya spp. (Dahan and Benayahu 1997; Fabricius and Alderslade 2001), and apparently occurs with healthy as well as stressed specimens. Eventually these recruits disappeared, most likely due to heavy sedimentation of the culture rocks they were growing on, in accordance with the suggestions of Dahan and Benayahu (1997).
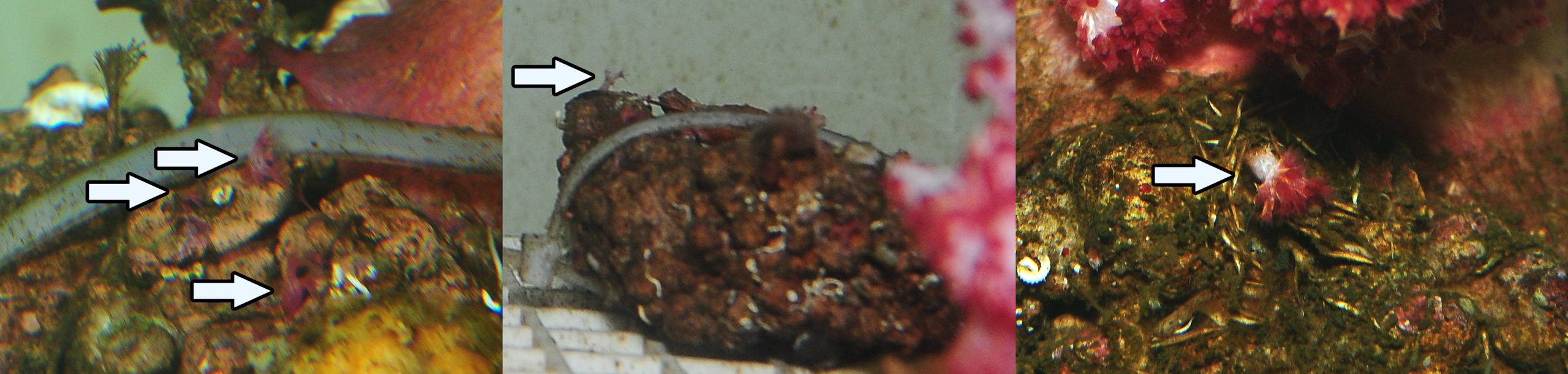
In December 2014, about a month after the first experiment had started, several recruits were found (white arrows). These fragments are released by colonies as a means of asexual reproduction.
Colony expansion behavior – Experiment 1
Field observations indicate these corals expand about 15 hours per day, with expansion predominantly occurring during the night (Fabricius 2014). During daytime, these corals expand as well, but possibly less than at night. During experiment 1, the nocturnal expansion and contraction behavior of three corals was recorded with a camera having night vision capability (Sony HDR-CX505), together with a low power aquarium LED light.
During one 12-hour night, the corals were found to be in an expanded state for about 8.5 hours. Although these data are preliminary, they indicate that in aquaria, Dendronephthya corals during a large part of the night, just like in the field. This suggests it is important to feed these corals both at night and during the day, which was done during the current experiments. In addition, the data suggest it takes these corals much more time to inflate than to deflate. Finally, although the timing of inflation/deflation is different for each individual, the total time spent in a given state is similar between the three colonies.
https://youtube.com/watch?v=s_ClcPsac7M
Time-lapse video showing the expansion and contraction behavior of Dendronephthya. Time compression is 100-fold, with approximately 12 hours of footage condensed to about 7 minutes. Interestingly, although each colony seems to have its own rhythm, the total time spent in a given state is comparable between corals.
Coral health and growth – Experiment 2
The second batch of corals was not subjected to weighing and air exposure, and showed good colony expansion from day one. This supports the view that Dendronephthya spp. are sensitive to air exposure (Fabricius 2014). However, the different orientation of the colonies may also have positively affected colony expansion. During this experiment, all corals were fixed to the vertical surface of the tables. Delbeek (2002) and Fabricius (2014) have suggested these corals get irritated by touching surfaces, which indeed seemed to occur during experiment 1 when they were positioned on top of the tables. Thus, mounting the corals from a vertical surface could have reduced surface irritation and improved expansion. In addition, more plankton was dosed daily compared to the first experiment, which also could have triggered increased expansion.
Whatever the reasons for the improved coral appearance during the second experiment, again all colonies showed clear signs of decline, save for one species, of which only one specimen could be obtained. A similar pattern of decline was observed, starting with loss of polyps, followed by decreased hydrostatic colony pressure.
During this experiment, two snails known as false cowries were found on two different coral colonies. At least one of these was Diminovula culmen (van Ofwegen 2015), and it clearly left grazing marks on its host coral. It also regularly produced egg clusters. Whether these hatched successfully is unclear, as only one additional cowrie was found. Both snails were removed and preserved in alcohol for future determination.
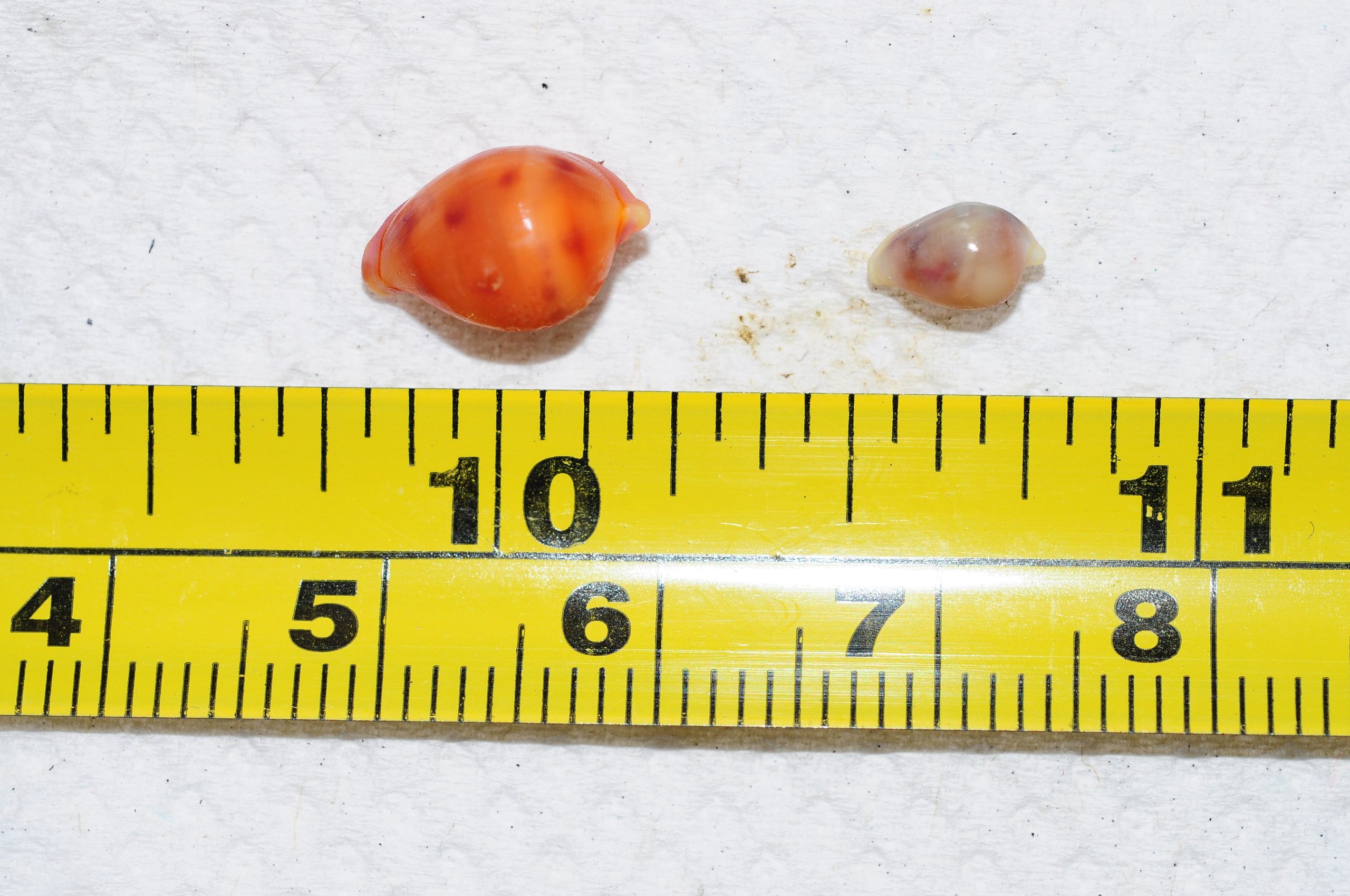
Two false cowries that were found in the aquarium. The shells are visible as the mantle no longer covers it when the snails are exposed to air. The specimen on the right is Diminovula culmen, the one on the left remains unidentified, but possibly is D. culmen as well. Scale bars are in inches (top) and centimeters (bottom).
Next to quantity, food quality may have been insufficient, i.e. the food mixture may have been deficient in specific prey items and thus organic compounds. Fabricius et al. (1995a) have shown that Dendronephthya hemprichi consumes the larvae of bivalves and gastropods, as well as copepods. Together, these prey items constitute over 97% of all zooplankton present in sampled coral polyps collected in their natural habitat. Although the total energy gained from zooplankton is limited, it may provide essential compounds for growth. It is possible that the rotifers provided do not match the nutrition profile of natural prey, which is difficult to replicate. For example, fatty or amino acids may be unbalanced for Dendronephthya growth. A possible candidate would be aspartic acid, which is essential for organic matrix synthesis and sclerite formation (Allemand et al. 1998, 2004; Rahman et al. 2006a,b). Without sufficient quantities of this amino acid, the supporting sclerite bundles around Dendronephthya polyps may not be produced properly. At this point in time, however, this remains conjecture, as the diverse combination of phytoplankton and zooplankton provided should have resulted in a very complete diet. As it more closely resembles the natural zooplankton prey of Dendronephthya, the small calanoid copepod Parvocalanus crassirostris may yield better results than rotifers.
Next to possible deficiencies in particulate or dissolved organic compounds, water flow rates could have impacted the experiment. The colonies placed closest to the flow pumps experienced higher flow rates than the corals placed further downstream, with an overall flow range of 14-33 cm s-1. However, no clear correlation between coral health and flow rate seemed to exist; corals upstream degenerated at a similar rate as those farther downstream. In addition, the flow range applied should be appropriate for these corals (Fabricius et al. 1995a).
It is theoretically possible that oscillating flow would have yielded better results. Shallow growing corals experience regular changes in flow rate and direction due to the tides (time scale of hours) and wave action (time scale of seconds), allowing both colony sides to feed. This may enhance the feeding efficiency of colonial organisms such as corals (Hunter 1989). However, field observations indicate that many Dendronephthya spp. do not experience alternating flow patterns on reefs, as they mostly grow below the intertidal zone at depths of 20 meters (67 feet) and beyond (Fabricius and Alderslade 2001). Thus, the flow rates and patterns used in these experiments are unlikely to have caused the observed colony decline.
https://youtube.com/watch?v=DVjqzOs9l_I
Dendronephthya colonies growing on a reef slope in the Andaman Sea, Thailand, exposed to strong water currents. Turbid water provides the corals with plankton and detrital matter. Video courtesy of Bart Stanczyk, www.reefhub.pl.
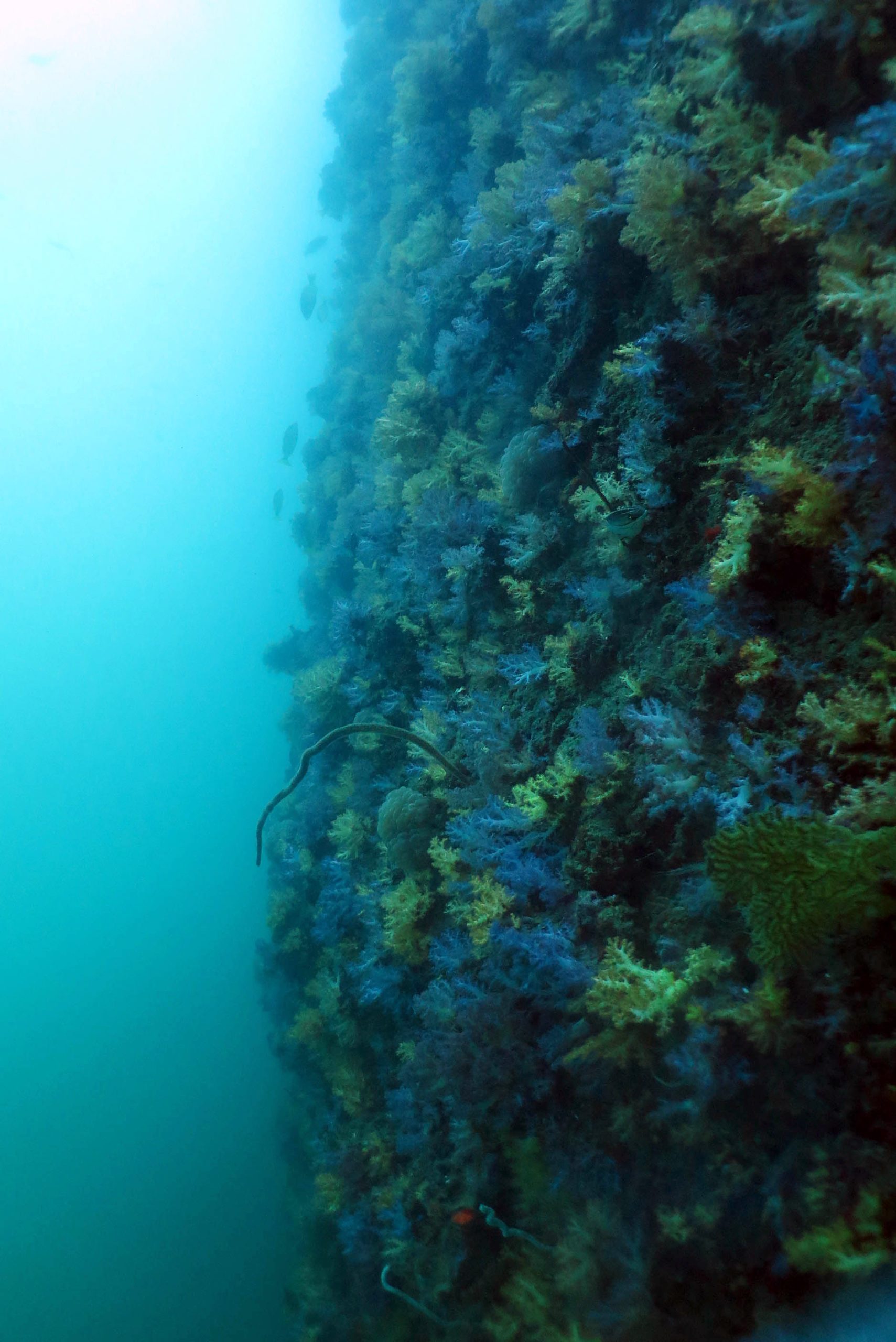
A reef slope with dense Dendronephthya cover in the Andaman Sea, Thailand. Photograph by Bart Stanczyk, www.reefhub.pl.
Sedimentation clearly played a role during the first experiment. As the corals were positioned on a horizontal substrate, the concave rocks to which they were attached collected significant amounts of detrital matter. This smothered both the base of the parent colonies as well as recruits that formed around the colonies. Sedimentation was much less evident during experiment 2, when all corals were mounted on a vertical surface.
It is interesting to note that one specimen appears to remain in good health. At the time of writing, this coral has remained in a stable condition for four months. This is possibly due to the coral’s different dietary preferences, which may better match the feeds provided during the experiments. This warrants further testing with more colony replicates, under different conditions and using different feeds. Grossowicz and Benayahu (2012) surmise that different species within the genus Dendronephthya feed on different types of plankton, which is reflected by their polyp morphology. For example, D. sinaiensis has tentacle pinnules that are more narrowly spaced than those of D. hemprichi, with the distance between two rows of pinnules at the base of D. sinaiensis‘ tentacles being 28 µm, versus 50 µm in D. hemprichi. This may allow D. sinaiensis to feed on smaller prey items. Grossowicz and Benayahu (2012) theorize that relatively large phytoplankton species in a size-range of 25-50 µm, such as the Red Sea dinoflagellates Ceratium fusus and Ceratocorys sp., are consumed by D. hemprichi. Smaller phytoplankton, such as the green algae Nannochloropsis sp. and Tetraselmis sp., may be captured more effectively by D. sinaiensis as it has longer and denser pinnules. They also state that D. sinaiensis is a more specialist feeder, in contrast to D. hemprichi, which seems to be a generalist feeder. It is possible that most corals used in the preliminary experiments presented here could not effectively feed on the small phytoplankton provided, with a cell size range mostly within 0.6-20 µm. Although Rhodomonas usually is larger, with a cell length of up to 30 µm, the amount fed may have been insufficient.
Conclusions
The preliminary experiments presented here reaffirm the prevailing opinion that Dendronephthya corals are highly difficult to maintain in aquaria. Even though water flow rates and patterns closely matched those found in the natural habitat of these corals, significant amounts of plankton were provided and water quality was maintained adequate, most corals quickly deteriorated. It remains to be determined what factor(s) determine the health and growth of Dendronephthya spp. Food quantity and quality remain key variables to be studied in the future. Future experiments may reveal differences in survival rates and growth between species within the Dendronephthya genus, as different polyp morphologies allow them to feed on different food items.
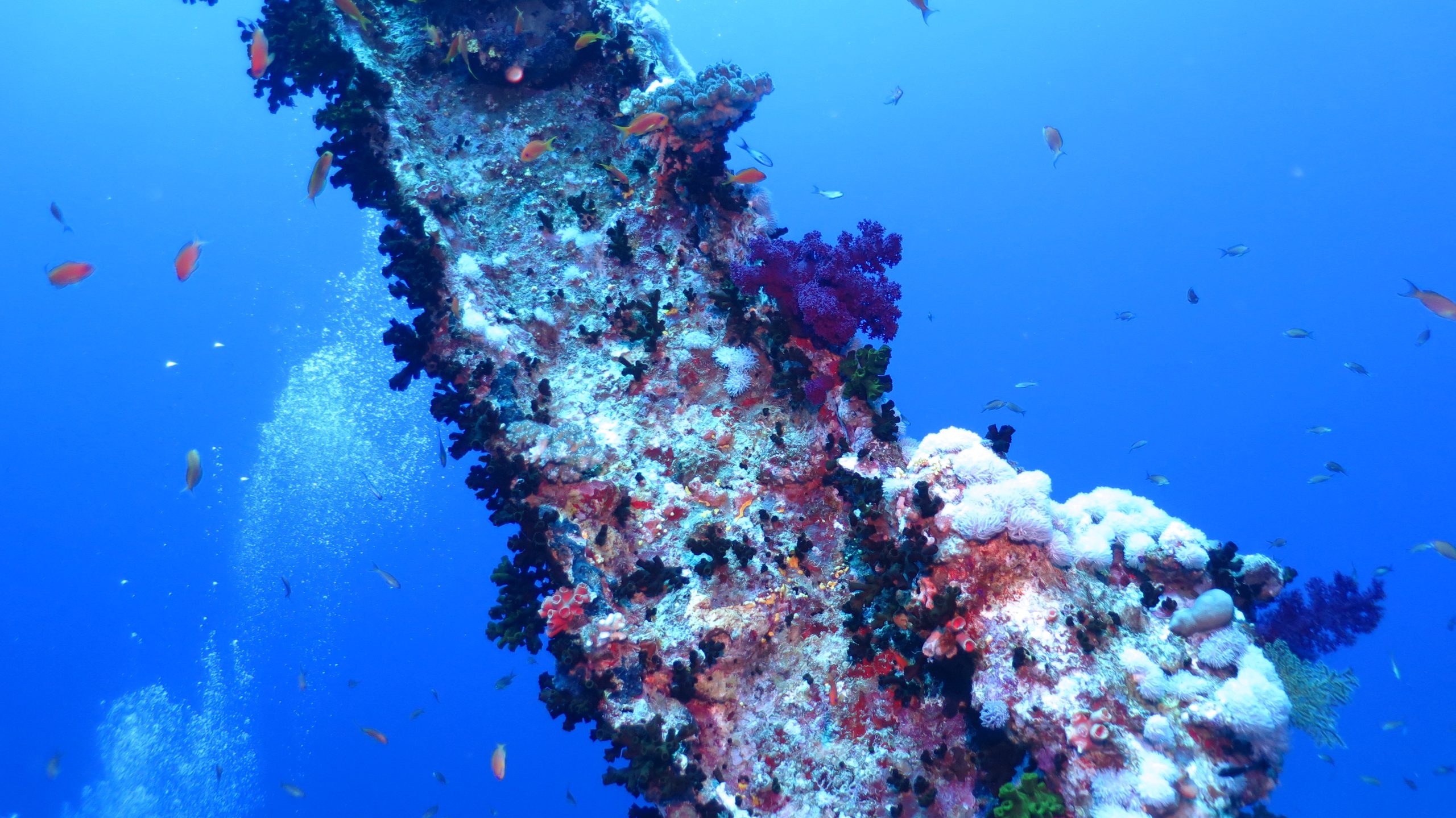
Azooxanthellate corals, here Dendronephthya and Tubastraea, rely on plankton and other organic matter for their survival. Photograph by Bart Stanczyk, www.reefhub.pl.
For now, I recommend that aquarists enjoy these corals in their natural habitat, rather than in a home aquarium. Still, if we are to learn more about the biology and husbandry requirements of these corals, especially their feeding preferences, we will have to continue conducting small scale aquarium experiments. One day, we may be able to truly replicate the biodiversity found on coral reefs, with thriving sponges, tunicates, bryozoans, bivalves, crinoids, (a)zooxanthellate corals and many other invertebrates. Such a reef display would be truly inspiring and have great educational value.
Acknowledgements
I wish to thank the following private and corporate sponsors, whose generous support made this research project possible: Anthony28, kuzia2k, focapa, Fotoservice Ijmond, Ted Bergström, carsten8117, perchikbest, tunixpatriot, valerya.voronina, Sergey Osmanov, Cezet, art186, valensa76, olivervm, Elena Ivanova, norkinoff, gpieterse, martijn.van.beek2, all-safronov, centralreef, Marielle Weisscher, karta-90, Arjen Tilstra, wedi71, lev22, spawn.adt, cyclod, ramsey5, basopotam, Jeffrey, K Ho, ethan073, Josef Barak, Jaap van Wingerden, Michael E., Sophia Thompson, R. Dokter, Morfeus, gregior, arduan77, b_rapacz, nstrochkov, olecha7, Bart Stanczyk, Magdalena Ryba, HetmanPL, irmina, rafalkruczek, koen-hermans, DLTEthomas.hailey, Jack, Bart Jansen, wbuzatto, Glenn Fong, Alexandra Rose, Karin & Hans, Christine Wijgerde, tanuhov, Edo van Bruggen, AquaViva Coral Farm Mexico, ccd.carreira, Lieke Wijgerde, Catarina Silva, saltvattensguiden.se, Advanced Aquarist, Jenlen.ru, ReefHub.pl, Reef Club, Reefcentral.ru, Porifarma, Bioted Marine, Living Reef Orlando, Reed Mariculture, Open Haarden Centrum Vlaardingen, Tropic Marin, EcoDeco, Wageningen UR, Jarathana International, BV van Dijk projectontwikkeling and BlueLinked. Special thanks to Michaël Laterveer (bluelinked.eu), Leonard Ho (advancedaquarist.com), Bart Stanczyk (reefhub.pl) and Walmyr Buzatto (reefclub.com.br) for their wonderful support, and Charles Delbeek for his inspirational 2002 article in Advanced Aquarist.
References
- Allemand D, Ferrier-Pagès C, Furla P, Houlbrèque F, Puverel S, Reynaud S, Tambutté É, Tambutté S, Zoccola D (2004) Biomineralisation in reef-building corals: from molecular mechanisms to environmental control. C R Palevol 3:453-467
- Allemand D, Tambutté E, Girard JP, Jaubert J (1998) Organic matrix synthesis in the scleractinian coral Stylophora pistillata: role in biomineralization and potential target of the organotin tribulyltin. J Exp Biol 201:2001-2009
- Ayukai T (1995) Retention of phytoplankton and planktonic microbes on coral reefs within the Great Barrier Reef, Australia. Coral Reefs 14:141-147
- Dahan M, Benayahu Y (1997) Clonal propagation by the azooxanthellate octocoral Dendronephthya hemprichi. Coral Reefs 16:5-12
- De Goeij JM, van Oevelen D, Vermeij MJA, Osinga R, Middelburg JJ, de Goeij AFPM, Admiraal W (2013) Surviving in a Marine Desert: The Sponge Loop Retains Resources Within Coral Reefs. Science 342:108-110
- Delbeek JC (2002) Non-photosynthetic Corals: They really are hard! Advanced Aquarist 1(1)
- Fabricius KE (2014) AIMS Senior Principal Research Scientist, Australian Institute of Marine Science, Townsville, Australia, personal communication
- Fabricius KE, Alderslade P (2001) Soft corals and sea fans – A comprehensive guide to the tropical shallow water genera of the Central-West Pacific, the Indian Ocean and the Red Sea. Australian Institute of Marine Science, Townsville, Australia. 264 p
- Fabricius KE, Benayahu Y, Genin A (1995b) Herbivory in asymbiotic soft corals. Science 268:90-92
- Fabricius KE, Genin A, Benayahu Y (1995a) Flow-dependent herbivory and growth in zooxanthellae-free soft corals. Limnol Oceanogr 40:1290-1301
- Grossowicz M, Benayahu Y (2012) Differential morphological features of two Dendronephthya soft coral species suggest differences in feeding niches. Mar Biodiv 42:65-72
- Grover R, Maguer JF, Allemand D, Ferrier-Pagès C (2006) Urea uptake by the scleractinian coral Stylophora pistillata. J Exp Mar Biol Ecol 332:216-225
- Grover R, Maguer JF, Allemand D, Ferrier-Pagès C (2008) Uptake of dissolved free amino acids (DFAA) by the scleractinian coral Stylophora pistillata. J Exp Biol 211:860-865
- Heidelberg KB, O’Neil KL, Bythell JC, Sebens KP (2010) Vertical distribution and diel patterns of zooplankton abundance and biomass at Conch Reef, Florida Keys (USA). J Plankt Res 32:75-91
- Heidelberg KB, Sebens KP, Purcell JE (2004) Composition and sources of near reef zooplankton on a Jamaican forereef along with implications for coral feeding. Coral Reefs 23:263-276
- Holzman R, Reidenbach MA, Monismith SG, Koseff JR, Genin A (2005) Near-bottom depletion of zooplankton over a coral reef II: relationships with zooplankton swimming ability. Coral Reefs 24:87-94
- Hunter T (1989) Suspension feeding in oscillating flow: the effect of colony morphology and flow regime on plankton capture by the hydroid Obelia longissima. Biol Bull 176:41-49
- Matthews C (2008) Report On a Successful Husbandry Method for a General Azooxanthellate Reef System Including Dendronephthya. Reefkeeping 7(2)
- Palardy JE, Grottoli AG, Matthews KA (2006) Effect of naturally changing zooplankton concentrations on feeding rates of two coral species in the Eastern Pacific. J Exp Mar Biol Ecol 331:99-107
- Rahman MA, Isa Y, Takemura A, Uehara T (2006a) Analysis of Proteinaceous Components of the Organic Matrix of Endoskeletal Sclerites from the Alcyonarian Lobophytum crassum. Calcified Tissue International 78:178-185
- Rahman MA, Isa Y, Uehara T (2006b) Studies on Two Closely Related Species of Octocorallians: Biochemical and Molecular Characteristics of the Organic Matrices of Endoskeletal Sclerites. Marine Biotechnology 8:415-424
- Seixas P, Coutinho P, Ferreira M, Otero A (2009) Nutritional value of the cryptophyte Rhodomonas lens for Artemia sp. J Exp Mar Biol Ecol 381:1-9
- van Ofwegen L (2015) Senior Researcher – Marine Zoology, Soft corals. Naturalis Biodiversity Center, Leiden, The Netherlands, personal communication
- Wijgerde T (2013) Coral Feeding: An Overview. Advanced Aquarist 12(12)
- Wijgerde T, Spijkers P, Karruppannan E, Verreth JAJ, Osinga R (2012) Water flow affects zooplankton feeding by the scleractinian coral Galaxea fascicularis on a polyp and colony level. J Mar Biol doi:10.1155/2012/854849
- Yahel R, Yahel G, Berman T, Jaffe JS, Genin A (2005a) Diel pattern with abrupt crepuscular changes of zooplankton over a coral reef. Limnol Oceanogr 50:930-944
- Yahel R, Yahel G, Genin A (2005b) Near-bottom depletion of zooplankton over coral reefs: I: diurnal dynamics and size distribution. Coral Reefs 24:75-85
0 Comments