In our efforts to replicate nature, it is only natural that hobbyists consider intense lighting a prerequisite to success with symbiotic invertebrates. In fact, there is often a mindset among many hobbyists that there is no such thing as too much light, and that an aquarium cannot be over-lighted, especially when maintaining small-polyp stony corals (or ‘SPS’ genera, such as Acropora, Stylophora, Pocillopora, etc.). The lighting industry has happily responded with fixtures housing 250, 400 and even 1,000-watt lamps. We are led to believe that if a little light is good, then a lot of light is even better! Hence, truly ‘industrial’ types of lamps have been tried, such as the 1000-watt Fusion sulfur lamp that produces light intensity at least one magnitude higher than the most intense sunlight! It would seem that our concerns about lighting are finally over; that it is just a matter of time before these 400 or 1000-watt lamps find their way to our tanks, and our corals will respond in a positive manner, literally growing out of the water to bask under an unblinking artificial sun. We have not only mimicked nature – we have improved upon it!
We take this information and combine it with anecdotal evidence from hobbyists and make sweeping statements, such as:
- Small-polyp corals require intense lighting!
- Soft corals require less light than stony corals!
- Lighting systems utilizing metal halide lamps are necessary to maintain SPS corals!
It appears as if we have it all figured out. We forgot to ask the corals.
This article will examine the optimal amounts of light required for 5 Hawaiian stony corals, 1 soft coral and 1 Tridacna clam, and is intended to complement a previous article “Too Much Light!” (Riddle, 2004a). Those proponents of unlimited photoacclimation by symbiotic zooxanthellae, and hence extreme lighting, will likely be surprised by these findings. And, as a reference, a quick listing of photosynthetic data for other coral/zooxanthellae symbioses from various references is offered at the conclusion of this article.
The Photosynthesis Meter
How do we ‘ask’ corals how much light they (and their symbiotic zooxanthellae) prefer? Fortunately, we can assess light requirements of symbiotic zooxanthellae through use of a ‘photosynthesis meter’ – a Pulse Amplitude Modulation fluorometer, or PAM meter for short. Previous articles (see Riddle, 2004a) have described in some detail the functions of this instrument. Suffice it to say that a PAM meter can determine which photosynthetic pathways are followed by absorbed photons/electrons. In essence, this device, so eloquent in its simplicity, enables us to understand the lighting requirements of any photosynthetic organism, including zooxanthellae within coral tissues.
Basically, photosynthesis and fluorescence of chlorophyll a (chl a) are processes competing for light particles (photons). Generally, when the rate of photosynthesis is high, chl a fluorescence is low. Conversely, high fluorescence of chlorophyll usually means a low rate of photosynthesis. The PAM meter is able to distinguish between these processes and, through use of filters, a photo-amplifier and a microprocessor (along with a quantum meter) can accurately estimate not only the number of photons available for photosynthesis, but how they are used (or not used) in the photosynthetic process.
This particular meter, a PAM-210 (Teaching PAM), is manufactured by Heinz Walz, Effeltrich, Germany. Since the instrument’s housing isn’t waterproof, a fiber optic cable connected to the meter allows in situ measurement of the rate of photosynthesis in submerged organisms. This meter does have its limitations – it cannot determine the minimum amount of light needed (the compensation point); however, it can determine the light intensity required for optimal photosynthesis (the saturation point). Further, it can determine when there is too much light – a situation potentially harmful to the coral/symbiont (known as photoinhibition), as well as the hobbyist’s bank account (in a process called bankruptcy).
Photons are absorbed by two ‘photosystems’ within zooxanthellae. Each photosystem contains chlorophylls, as well as other photopigments (some pigments are exclusive to one photosystem – see Riddle, 2004a). These absorbed photons, now in the form of electrons, power photosynthesis. In order for photosynthesis to proceed smoothly, there must be an equal number of ‘donor’ and ‘acceptor’ molecules; if not, there is a ‘traffic jam’ of electrons, potentially resulting in damage or destruction of one (or both) of the photosystems. Stressful conditions or damage to photosystems can result in expulsion or death of symbiotic zooxanthellae, which, of course, is called ‘bleaching’.
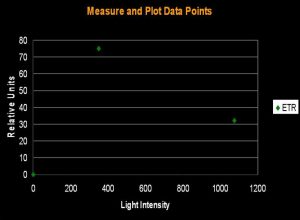
Figure 1. The initial step in determining Photosynthetic Saturation and Photonihibition Points. A PAM Fluorometer measures the Electron Transport Rate (ETR) within symbiotic zooxanthellae. The x-axis is light intensity (PAR), in units of µmol photons ·m²·sec; the y-axis is the Electron Transport Rate, reported in units of µmol electrons·m²·sec. All charts in this article use these units.
The electron shuttle between Photosystem I and Photosystem II is called the Electron Transport Rate (ETR). It is calculated by simply multiplying the Photosynthetic Yield by the amount of available light. ‘Photosynthetic Yield’ is determined by the PAM meter, while, in this case, the ‘available light’ is monitored by a Li-Cor LI-189 quantum meter with a 2 pi, cosine corrected sensor and reported as Photosynthetically Active Radiation (PAR) in units of micromole photons per square meter per second (µmol·m²·sec; as a point of reference, sunlight at noon on a cloudless day in Hawai’i can be as high as 2,200 µmol·m²·sec ). Since we do not actually measure the amount of absorbed light, we are estimating the Relative Electron Transport Rate (simply referred to as ETR in this article). Thus, ETR is an estimate of the number of photons used in the process of photosynthesis. Yield of photosynthesis usually decreases with increased light intensity (due to ‘dumping’ of excess absorbed energy through the Xanthophyll Cycle, a sort of Non-photochemical Quenching, or NPQ for short. In effect, the xanthophyll cycle acts as a ‘safety valve’ when light intensity is excessive, and is in excess of that needed or used in photosynthesis). This process is called Dynamic Photoinhibition (hereafter referred to as simply ‘Photoinhibition’). Photoinhibition is seen as a decrease in the rate of photosynthesis, even as light intensity increases.
Determining Saturation of Photosynthesis
Several methods can be used to determine when saturation has occurred. One method simply states that saturation has happened when photosynthetic activity ‘flat lines’ on a chart. Another method involves determining a certain percent merely by multiplying the photosynthetic maximum by a factor (say, 95%). The method we’ll use here is one described by Kirk (2000): The rate of photosynthesis is linear to light intensity until it approaches the saturation point. If this linear trend were to continue and intersect a horizontal line even with the maximum amount of photosynthesis, we can determine the ‘saturation onset’ by extended a line downwards to the x-axis of the chart (see Figures 1, 2 and 3).
In a similar fashion, we can determine onset of Photoinhibition – it is a mirror-image of onset of Saturation (this method is not shown on Figures 1-3).
The Corals in Captivity
These PAM meter experiments were conducted over a rather lengthy period of time and included many stony corals (Porites spp., Pocillopora meandrina, Pavona varians, and Montipora capitata) plus one soft coral (Sinularia densa). Also tested was a Tridacna maxima clam, donated to Friends of NELHA by the Kona Blue Water aquaculture facility. These corals/clams were maintained at the Natural Energy Laboratory Hawaii Authority (NELHA’s) outdoor systems, They were subjected to light intensities seem only in the most shallow of tide pools, and had sufficient time (months) to adapt to the increased light intensity.
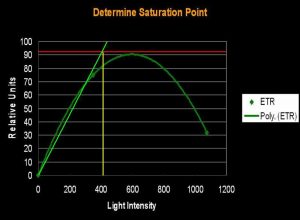
Figure 3. …the method described in the text defines the onset of Photosynthetic Saturation. In this hypothetical situation, the Saturation begins at a light intensity of ~400 µmol·m²·sec (or about 20,000 lux). Using a similar method, onset of Photoinhibition would be about 700 µmol·m²·sec (or about 35,000 lux).
Methods
In order to control light quality and intensity during the experiments, these animals were illuminated with a 400-watt Iwasaki ‘DL’ (daylight) metal halide lamp over a range of known intensities while the PAM meter determined the ETRs. The spectrum of the Iwasaki lamp closely resembles the spectrum of sunlight when filtered through shallow water (see Joshi, 2005, www.advancedaquarist.com/2005/8/aafeature/ ) although the corals, during the indoor experiments, were shielded from the lamp’s ultraviolet radiation (UVR) through use of an appropriate UVR-absorbing clear acrylic sheet. See Riddle, 2004b for info on the effect of lamp UVR on zooxanthellae photosynthesis.
Measurements with the PAM were taken for each coral at various light intensities. Electron Transport Rates were calculated as described earlier, and the Saturation Points were determined as per Kirk, 2000.
Results
The results suggest that corals’ zooxanthellae exhibit a broad, but sometimes limiting, range of photoadaptive capacities.
The Corrugated Coral, Pavona varians
- Known Zooxanthella Clade (in Hawaii): C27
- Onset Saturation Point: 110 µmol·m²·second (or about 5,500 lux, see Figure 5).
- Onset Photoinhibition Point: ~350 µmol·m²·second (or about 17,500 lux)
Figure 4. Pavona varians. This coral is remarkably adaptable to low light situations and is often found in crevices. Note the host tissue’s delicate blue-green fluorescence.
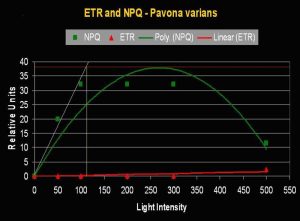
Figure 5. Onset of Photosaturation in this Pavona specimen occurs at ~110 µmol photons·m²·second (or ~5,500 lux).
Mound Coral Porites lutea (formerly P. evermanni)
- Known Zooxanthella Clade (in Hawaii): C15
Figure 6. Formerly called Porites evermanni, this coral is now known as P. lutea. In any case, it is an attractive coral, as well as hardy. These corals (in Hawaii) are known to contain the zooxanthellae clade C15. These symbiotic algae have a high photosynthetic Saturation point and Photoinhibition point, and are thermally resistant, making them an idea candidate for tide pool inhabitation.
- Onset Saturation Point: ~400 µmol·m²·second (or about 20,000 lux, see Figure 7).
- Onset Photoinhibition Point: ~750 µmol·m²·second (or about 37,500 lux).
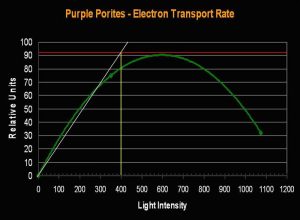
Figure 7. Porites lutea’s Electron Transport Rate and Saturation Point. Compare the number of electrons (about 90 µmol electrons·m²·second) moving through the photosynthetic apparatus to that seen in the Pavona specimen (above, Figure 5).
The Rose Coral Pocillopora meandrina
- Known Zooxanthella Clade (in Hawaii): C1c
Figure 8. A macro shot of the beautiful Rose Coral (Pocillopora meandrina). Note the green fluorescence within the basal portion of the polyp.
- Onset Saturation Point: 275 µmol photons·m²·second (or about 13,750 lux, see Figure 9).
- Onset Photoinhibition Point: 425 µmol·m²·second (or about 21,250 lux).
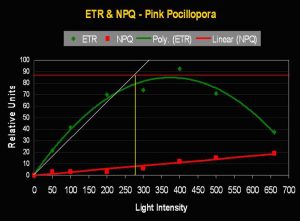
Figure 9. The Saturation Point of this pink Pocillopora meandrina is relatively high (~275 µmol photons·m²·second). Figures 9 and 11 also illustrate Non-photochemical Quenching (NPQ), which demonstrates ‘dumping’ of excessive light energy away from the photosynthetic apparatus via the xanthophyll cycle. Hence, two ‘safeties’ are in play – chlorophyll fluorescence and protective xanthophylls channel energy away from chlorophyll within zooxanthellae, thus braking photosynthesis.
The Mound Coral Porites lobata (yellow-green variant)
- Known Zooxanthella Clade (in Hawaii): C15
- Onset Saturation Point: ~250 µmol·m²·second (or about 12,500 lux, see Figure 11).
- Onset Photoinhibition Point: ~350 µmol·m²·second (or about 17,500 lux).
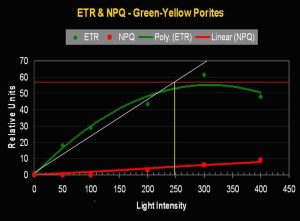
Figure 11. Porites lobata’s (yellow-green variant) Electron Transport Rate. The maximum number of electrons moved is 37% less than seen in the purple P. lutea variant (above). The Saturation Point in the yellow-green Porites is 38% lower than the purple P. lutea. Is this a case of a ‘purple’ pigment in P. lutea acting as a photoprotectant?
Rice Coral, Montipora capitata (formerly M. verrucosa).
- Known Zooxanthellae Clades (in Hawaii): Seems depth-dependent – Shallow water: C31; Deep water: C26 or C26a; D1a (orange variant).
- Onset Saturation Point: ~135 µmol·m²·second (or about 6,750 lux, see Figure 13).
- Onset Photoinhibition Point: Unknown, but possibly ~250 µmol·m²·second (12,500 lux).
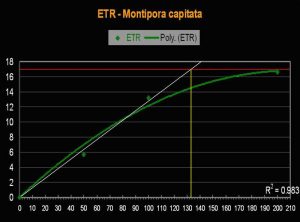
Figure 13. The Electron Transport Rate in this Montipora specimen suggests a remarkably low saturation point.
Why Would a ‘Low Light’ Coral Settle in a Shallow, Well-lighted Tide Pool?
It is not unusual to see ‘misplaced’ corals in the Big Island’s tide pools, especially Pavona and Montipora species – why would this be? Only the coral knows for sure, but we can speculate that the coral planulae larva ‘sensed’ a chemical cue and settled in a spot that seemed suitable. It is believed that specific substances in calcareous algae or bio-films are ‘flypapers’ for coral larvae (Morse and Morse, 1996). In this particular case, it is possible that the Montipora larvae sensed, and settled on, a low-light adapted calcareous algae growing in a crevice on under a shaded ledge. The coral literally grew into a high-light environment, where it survives but doesn’t thrive (but see zooxanthellae growing in the shaded portions of the coral in Figure 12).

Figure 14. The Hawaiian soft coral Sinularia densa. Its shape causes it to be sometimes confused with the stony coral Porites.
The Soft Coral, Sinularia densa (formerly S. abrupta)
- Known Zooxanthella Clade (in Hawaii): Unknown, but probably a ‘C’ Clade.
- Onset Saturation Point: ~207 µmol·m²·second (or about 10,350 lux, see Figure 15).
- Onset Photoinhibition Point: Unknown, but likely ~400 µmol·m²·second (or about 20,000 lux).
The tridacnid clam, Tridacna maxima
- Known Zooxanthella Clade: Unknown, but probably an ‘A’ Clade.
- Onset Saturation Point: No saturation seen at 600 µmol·m²·second (or about 30,000 lux, see Figure 17).
- Onset Photoinhibition Point: No photoinhibition reported at 1,900 µmol·m²·second (or about 95,000 lux; Ralphet al., 1999, but see comments below).
Tridacna clams are popular inhabitants of captive reefs and for good reason – their form and coloration can be in sharp contrast to other invertebrates. They are also quite hardy and can survive for years. It is generally accepted that Tridacna clams (especially T. maxima and T. crocea) need a great deal of light in order to really thrive.
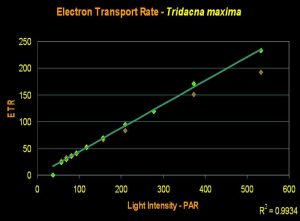
Figure 17. An ETR of Tridacna maxima. Even at 600 µmol electrons·m²·sec, we see no indication of the onset of photosynthetic saturation, much less Photoinhibition.
Results from recent experiments suggest that most aquarium lighting systems will not saturate photosynthesis. An Iwasaki 400-watt metal halide lamp with a polished aluminum reflector was used to irradiate the clam with light intensity as high as 600 µmol·m²·second (or about 30,000 lux). Ralph et al. (1999) found no Photoinhibition when determining ‘rapid light curves’ with light intensity to 1,900 µmol photons·m²·second (or about 95,000 lux), but view this information cautiously. ‘Rapid Light Curves’ are determined in short order (a matter of seconds), and this short time frame does not allow time for conversion of xanthophylls (one of the ‘safety valves’ in photosynthesis).
There are several possibilities for the high saturation point. First, tridacnid clams are known to contain light-tolerant zooxanthellae clades (specifically ‘A’ clades). Mantle thickness is also an important factor – it is possible that mature clams’ thicker mantles allow for self-shading of zooxanthellae. A third possibility is, of course, the refractive nature of the mantle. It is believed that the ‘bending’ of light rays within the mantle tissue can alter the amount of photosynthetically usable radiation (PUR).
As a footnote, the Tridacna clam in Figure 16 was once on display at the Natural Energy Laboratory in Kailua-Kona, Hawa’i. This clam, along with others, was recently donated to the Waikiki Aquarium in Honolulu.
Discussion
These corals’ relatively low saturation points might come as a surprise as it challenges the concept of corals being insatiable ‘light sponges’. Upon examination, though, the low saturation points make sense in the survival strategies of many corals. Numerous factors influence how much light actually penetrates shallow waters, such as the tide pools. We shall see how many corals are superbly adapted to their environment.
Solar radiation actually reaching the surface of a body of water can vary greatly at fine time scales. Obviously, passing clouds or an overcast sky are the most obvious influences. Another is the ‘shimmer’, ‘flicker’, or ‘glitter’ effect created by water surface motion. On a scale of hours, the altitude of the sun, and the reflection due to its angle of incidence, creates diurnal cycles. Seasonal variations of light intensity is another easily recognizable factor.
If a coral were to require very high light intensity for photosynthesis, how would it survive during prolonged storms with their associated ‘gray days’ (such as the 40-day period of rain recently experienced on the island of Oahu, Hawaii)?
Cloud cover isn’t the only way light intensity can be attenuated – water clarity also affects how much light reaches corals. Our initial inclination is to associate water clarity with turbidity (where suspended particles scatter light) or water color (where compounds are dissolved, not suspended, in the water column). Let there be no doubt that these two factors can and will lessen light transmission. However, there is another possibility, one of entrainment of air bubbles, which would be usually linked to wave action. Any observer of the ocean over a period of time has seen choppy ocean water turn (as is the case in the waters off the west coast of the Big Island of Hawaii) from blue to baby blue and, on really chaotic days, to milk-white. These variations in apparent water color aren’t due to water color but rather mostly to the highly reflective nature of air bubbles. Bubbles often appear very bright, or even ‘silvery’ in color due to their ability to reflect light.
So, the worst case scenario – a stalled weather front brings torrential rains along with rough seas. The violence of the water’s movement re-suspends sediments and terrestrial run-off full of decaying plant material (lending a yellow color to the water, which effectively filters some violet and blue wavelengths) mixes with an already churned ocean. On top of the dreary, gray (but warm) days, the ocean is full of suspended and dissolved matter along with billions of tiny air bubbles. To further compound the problem, coral polyps, in an effort to prevent being ripped to pieces by the violence of the ocean, are retracted. In their efforts to prevent tissue maceration, the polyps become effective at condensing their zooxanthellae concentrations, thus causing self-shading. It is a difficult time for the corals. Think this is a rare occurrence in Hawaii (and in the tropics in general)? Think again.
Light intensity to the corals is often significantly reduced, yet they manage to survive through a strategy of effectively utilizing low light levels (along with feeding when water currents allow).
There are a number of strategies to survive high light intensity, including Dynamic Photoinhibition through the ‘xanthophyll cycle’ or dumping absorbed light energy through chlorophyll fluorescence (the latter likely more effective than the former).
Polyp retraction and the self-shading (mentioned earlier) is also an effective way to reduce light to zooxanthellae. Some corals possibly use light reflection or tissue fluorescence to shield them from high light intensities. And, of course, many corals contain mycosporine-like amino acids (MAAs) which can absorb harmful UV-A and UV-B radiation.
In addition to self-shading, many shallow water corals live in shadows of the benthic landscape. Tops of corals may be exposed to the sun’s full measure (to the extend possible), while the sides are only lighted for brief periods. These shadows only become darker when the ocean conditions mentioned above are in place. The only effective strategy for the zooxanthellae is to have a low compensation and hence saturation point. See examples of shadowing in Figures 6, 10, 12, and 14. ‘Shadowing’ is a complicated topic. If it were not for light reflected from surrounding surfaces, a shadow would appear completely black. A white sand cover of the bottom makes for a good surface, while colors associated with ‘live rock’ are generally less reflective. The same holds true for the color of an aquarium’s internal surfaces – black acrylic obviously reflects little light, a blue background reflects wavelengths wavelengths usable in photosynthesis, though not as well as a white background. It is an interesting and virtually unexplored topic to the true reef aquarium aficionado.
Effect of Coral Color
Before beginning the brief discussion of the ‘coloration’ topic, I must admit that there is a good deal of speculation involved.
If we assume that the ‘purple’ and ‘yellow-green’ Porites lutea/P. lobata specimens listed above contain the same clade zooxanthellae (C15, and, to my knowledge, there is no evidence to the contrary), then we must wonder why the purple specimen has a much higher Electron Transport Rate. Is it because the pigment(s) with the purple coral selectively reflect those very wavelengths known to promote photosynthesis (namely blue and red)?
Various researchers have presented evidence that this is indeed the case. I have some experiments planned to test the efficiencies of variously colored pigments in preventing Photosynthetically Usable Radiation (PUR) from reaching symbiotic zooxanthellae. With any luck, these experiments will be completed by mid-year.
Soft Coral v. Stony Coral Saturation Points
It is an old saw in the hobby that soft corals require less light than small-polyp stony (SPS) corals. The evidence presented in this article contradicts this belief. The relatively high Electron Transport Rate seen in the Sinularia specimen suggests that its Saturation Point is higher than that of a couple of the stony corals. Instead of a broad, sweeping statement that soft coral require less light, we should be more interested in knowing the general saturation points of various zooxanthellae clades, and which lighting setups are appropriate.
Why Do the Tridacna Clam and Soft Coral Have High Saturation Points?
There are several possible answers to this question. First, the saturation point varies among zooxanthellae clades, and ‘A’ clades (such as found in many Tridacna species) are known to be tolerant of high light. Second, tissues of soft corals and clam mantles offer a relatively thick cross section (as compared to many SPS corals), and self-shading from other zooxanthellae or by coral/clam tissues is almost certain to occur. Finally, tridacnid (and some other photosynthetic clams’) mantle tissues are layered and light is refracted. This explains why viewing clams from above gives the most spectacular view.
Which Lighting ‘Setup’ is OK?
After years of examining lighting systems and tinkering with various lighting combinations (and ‘asking’ zooxanthellae how much light they prefer), it seems obvious that relatively low light levels are better than higher levels for most of the animals/zooxanthellae examined. Certainly, it appears that 200-300 µmol photons·m²·second (~10,000 – 15,000 lux) is enough light to saturate photosynthesis in many cases.
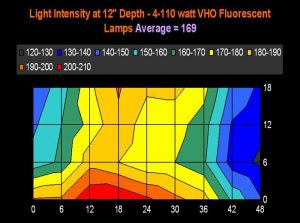
Figure 18. This charts plots 36 lighting data points from a VHO lighting system over a 55-gallon aquarium (48” long, 18” wide, and at a depth of 12 inches). If we could visual light intensity at 12” while looking downwards into an aquarium, this is what we might see. The legend shows lighting increments, and is reported in micromole photons per square meter per second. ‘Averaged’ light intensity was simply figured using the 36 data points.
Figures 17 and 18 demonstrate light intensities generated by two lighting systems at a depth of 12 inches (~5cm). Tridacna clams would likely do well in the upper reaches of these aquaria, while low-light corals, such as Montipora capitata and Pavona varians (and probably other animals containing low light-tolerant ‘C’ zooxanthellae clades) would thrive in the deeper (or shaded) portions of these tanks.
Some might argue that those corals capable of generating colorful fluorescent/reflective pigments in lower light situations. However, preliminary results suggest that fluorescent/reflective pigments are expressed at light intensities close to the coral/zooxanthellae’s Saturation Point. There are exceptions – at least one green and orange fluorescent compounds become apparent in low light, while reds, pinks and other colors are seen in higher light situations.
At this point, it seems that zooxanthellae/hosts requiring really intense light generated by higher wattage (250-400w) lamps are the exception instead of the rule. ‘Blasting’ corals with light could actually inhibit photosynthesis and possibly slow their growth.
Further information on light intensities within aquaria can be garnered from Kirda, 2003 ( http://advancedaquarist.com/issues/august2003/feature.htm ). Note that the Apogee quantum meter used by Kirda does an admirable job at measuring PAR, but probably underreports slightly in many situations (See Riddle, 2005 for details on the performance of two brands of quantum meters: http://www.advancedaquarist.com/2005/7/review/). However, Kirda’s article gives an excellent idea of light intensities in aquaria using different lighting systems.
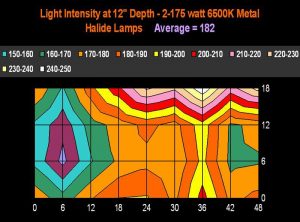
Figure 19. The same concept as in Figure 18, except this time the aquarium is illuminated by 2-175 watt 6,500K metal halide lamps. Depth of measurements were, as in Figure 18, taken at a depth of ~5 cm (12”). The legend shows lighting increments, and is reported in micromole photons per square meter per second.
Saturation Points from Various Sources
Fortunately, peer-reviewed literature contains a wealth of information on corals and photosynthesis. Here, we’ll examine a few data points on corals’ Compensation, Saturation and Photoinhibition Points from various sources. See Table 1.
In Closing
A picture is worth a thousand words. Figures 18 and 19 are of SPS corals at relatively great depths. Is this to say that most stony corals can adapt to extremely low light intensities? Of course not. However, it does shoot neat holes in hypotheses claiming SPS corals require high light in order to survive.
These corals contain zooxanthellae clades (probably undescribed clades) that are capable of efficient light collection in environments of greatly diminished intensities.
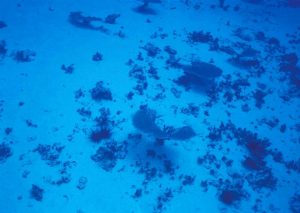
Figure 20. Acropora specimens growing at Enewetak at a depth of 76m (249 feet). Photograph taken from a submersible research craft. Photo courtesy of Hawai’i Undersea Research Laboratory (HURL).
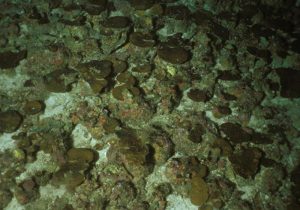
Figure 21. Another small-polyp stony coral (Leptoseris) growing at a depth of 118m (386 feet). Leptoseris is known to inhabit water as deep as 165m (540 feet), making it the deepest known photosynthetic coral. Photograph courtesy of Hawai’i Undersea Research Laboratory (HURL).

Figure 22. The picture the travel brochures do not show. This is a common sight during afternoons on the west coast of the Big Island of Hawai’i (and other ‘tropical’ places). Corals survive because they are able to tolerate extended periods of relatively little light.
Acknowledgement
Mahalo to Dr. Edith Chave for obtaining permission to use the deep-water coral photos from HURL.
References
- Chalker, B.E., W.C. Dunlap and J.K. Oliver, 1983. Bathymetric adaptations of reef-building corals at Davies Reef, Great Barrier Reef, Australia. II. Light saturation curves for photosynthesis and respiration. Mar. Biol. Ecol., 73:37-56.
- Chalker, B.E., 1983. Calcification by corals and other animals on the reef. In: D.J. Barnes, (Ed.), Perspectives on Coral Reefs. Manuka, A.C.T., Australia.
- Chave, E. and A. Malahoff, 1998. In Deeper Waters: Photographic Studies of Hawaiian Deep-sea Habitats and Life-forms. University of Hawaii Press, Honolulu. 125 pp.
- Davies, P., 1984. The role of zooxanthellae in the nutritional energy requirements of Pocillopora eydouxi. Coral Reefs, 2:181-186.
- Dubinsky, Z., P. Falkowski, J. Porter et al., 1984. Absorption and utilization of radiant energy by light- and shade-adapted colonies of the hermatypic coral Stylophora pistillata. Proc. Royal. Soc. London Ser. B, 222: 203-214.
- Falkowski, P. and Z. Dubinsky, 1981. Light-shade adaptation of Stylophora pistillata, a hermatypic coral from the Gulf of Eliat. Nature, 289, 172-174.
- Fenner, D., 2005. Corals of Hawai’i: A Field Guide to the Hard, Black and Soft Corals of Hawai’i and the Northwest Hawaiian Islands, Including Midway. Mutual Publishing LLC, Honolulu. 144 pp.
- Joshi, S., 2005. Underwater light field and its comparison to metal halide lighting. http://www.advancedaquarist.com/2005/8/aafeature
- Kirda, M., 2003. Lighting in reef tanks: Some actual data. http://advancedaquarist.com/issues/august2003/feature.htm
- Kirk, J.T.O., 2000. Light and Photosynthesis in Aquatic Ecosystems. Cambridge University Press, Cambridge. 509 pp.
- Morse, A.N.C. and D.E. Morse, 1996. Flypapers for coral and other planktonic larvae. BioScience, 46(4): 254-262.
- Ralph, P., R. Gademann, A. Larkum and U. Schreiber, 1999. In situ underwater measurements of photosynthetic activity of coral zooxanthellae and other reef-dwelling dinoflagellates endosymbionts. Mar. Ecol. Prog. Ser., 180: 139-147.
- Ralph, P., R. Gademann, A. Larkum and M. Kühl, 2002. Spatial heterogeneity in active chlorophyll fluorescence and PSII activity of coral tissues. Mar. Biol.; 141: 639-646.
- Riddle, D., 2004a. Too much light! http://advancedaquarist.com/issues/july2004/feature.htm
- Riddle, D., 2004b. Playing with poison – Ultraviolet radiation. http://advancedaquarist.com/issues/aug2004/feature.htm
- Riddle, D., 2005. Product Review: A comparison of two quantum meters, Li-Cor v. Apogee. http://www.advancedaquarist.com/2005/7/review/
- Riddle, D., 2006. Lighting by number – Types of zooxanthellae and what they tell us. http://www.advancedaquarist.com/2006/aafeature1/
- Secord, D. and G. Muller-Parker, 2005. Symbiont distribution along a light gradient within an intertidal cave. Limnol. Oceanogr., 50(1):272-278.
- Shick, J., M. Lesser, W. Dunlap et al., 1995. Depth-dependent responses to solar ultraviolet radiation and oxidative stress in the zooxanthellate coral Acropora microphthalma. Mar. Bio., 122: 41-51.
- Smith, D., D. Suggett and N. Baker, 2005. Is photoinhibition of zooxanthellae photosynthesis the primary cause of thermal bleaching in corals? Global Change Biology, 11: 1 – 11.
- Takahashi, S., Nakamura, T., M. Sakamizu, R. van Woesik and H. Yamasaki, 2004. Repair machinery of symbiotic photosynthesis as the primary target of heat stress for reef-building corals. Plant Cell. Physiol., 45(2): 251-255.
Host | Compensation | Saturation | Photoinhibition | Depth | Location | Ref |
---|---|---|---|---|---|---|
Acropora cervicornis | * | 281 | Yes | 17m | Atlantic | 2 |
Acropora cervicornis | * | 331 | * | 17m | Atlantic | 2 |
Acropora digitifera | 82 | 387 | * | 1m | Davies Reef, GBR | 1 |
Acropora divaricata | 10 | 77 | * | 40m | Davies Reef, GBR | 1 |
Acropora formosa | ~170 | ~340 | * | 1m | Davies Reef, GBR | 1 |
Acropora gemmifera | ~170 | ~340 | No | 1m | Davies Reef, GBR | 1 |
Acropora granulosa | ~53 | ~107 | No | 40m | Davies Reef, GBR | 1 |
Acropora microphthalma | * | ~300 | ~600 | * | * | 19 |
Acropora millepora (coenosarc) | * | ~190 | * | <2m | Heron Island, GBR | 13 |
Acropora millepora (polyp) | * | ~230 | * | <2m | Heron Island, GBR | 13 |
Acropora nobilis (coenosarc) | * | ~310 | * | <2m | Heron Island, GBR | 13 |
Acropora nobilis (polyp) | * | ~180 | * | <2m | Heron Island, GBR | 13 |
Anthopleura elegantissima (anemone) | 73 | None @ 540 | * | Cave | NW U.S. coast | 18 |
Cyphastrea serialia (coenosarc) | * | ~150 | * | <2m | Heron Island, GBR | 13 |
Cyphastrea serialia (polyp) | * | ~150 | * | <2m | Heron Island, GBR | 13 |
Montipora tuberculosa (coenosarc) | * | ~180 | * | <2m | Heron Island, GBR | 13 |
Montipora tuberculosa (polyp) | * | ~300 | * | <2m | Heron Island, GBR | 13 |
Pocillopora damicornis (coenosarc) | * | ~225 | * | <2m | Heron Island, GBR | 13 |
Pocillopora damicornis (polyp) | * | ~225 | * | <2m | Heron Island, GBR | 13 |
Pocillopora eydouxi | * | 323 | * | 5m | Guam | 4 |
Porites cylindrica (coenosarc) | * | ~200 | * | <2m | Heron Island, GBR | 13 |
Porites cylindrica (polyp) | * | ~200 | * | <2m | Heron Island, GBR | 13 |
Stylophora pistillata | 350 | 600-2,000 | * | * | Sinai, Egypt | 6 |
Stylophora pistillata (shade) | 40 | 200 | * | * | * | 5 |
Stylophora pistillata (light) | * | ~300 | ~600 | * | * | 5 |
0 Comments