I recently authored an article that examined the spectral characteristics of natural sunlight and how water depth of only two inches altered light quality. Selection of the shallow depth was deliberate – the scope of the associated experiments was to examine the maximum amount of each color (spectral bandwidth) that fell upon corals (for instance, were corals receiving more red light in an aquarium than in nature?). After publication of these results (see http://www.advancedaquarist.com/2013/11/aafeature), readers asked obvious questions (why was the depth so shallow?) and requested more information, particularly about spectral qualities at more depth. Logistical challenges would prevent repetition of the initial data gathering procedure – launching floating computer and battery-driven spectrometers and data loggers in deeper portions of the Pacific Ocean while hoping for clear weather throughout the day and preventing loss due to accident or theft is no easy task! After realizing I probably could not overcome the many obstacles preventing the gathering of data at greater depths, I began researching existing literature in order to estimate spectral qualities of light as it penetrated clear oceanic water. This article will present this information, along with the caveats we must realize when interpreting these data.
First, the spectral characteristics of sunlight reaching the earth’s (or ocean’s) surface are similar, but not absolutely uniform. Once light enters a body of water, absorption characteristics of the water column must be considered. In addition, we have to estimate the qualities of aquarium water in order to make meaningful comparisons. Light dosage (Daily Light Integral, or DLI) has to be considered as well. (DLI is calculated by multiplying the instantaneous PAR values (the number of photons falling upon 1 square meter per second, reported as µmol·m²·sec) by the number of seconds in the photoperiod and dividing by 1,000,000 to arrive at mol·m²·photoperiod.) Finally, optical qualities of aquarium water should be considered before meaningful comparisons are made. We will now examine these factors in more detail.
Definition of Spectral Bandwidths
The definition of spectral bandwidths (colors) varies from source to source. Hunt (1998) describes visible light as consisting of 6 bandwidths with a range of 380 to 780, while Wikipedia lists different definitions – this is not to be unexpected. There are no sharp transitions from one color to another, but a gradual change instead. I elected to use bandwidths from one of my old college textbooks but had to modify even those for practical reasons (I’ll explain in the next section). For our purposes, definitions of bandwidths are as follows:
- Violet: 400 – 430 nm
- Blue: 431 – 480 nm
- Green-Blue: 481 – 490 nm
- Blue-Green: 491 – 510 nm
- Green: 511 – 530 nm
- Yellow-Green: 531 – 570 nm
- Yellow: 571 – 580 nm
- Orange: 581 – 600 nm
- Red: 601 – 700 nm
Reason for Using Altered Bandwidths
An astute hobbyist will quickly recognize that bandwidths used in this article are different from most other references. For instance, bandwidths for those colors at opposite ends of the visible (violet and red) spectrum are truncated. The reason is this – calculations of the total dosage of light require knowledge of spectral characteristics as well as light intensity. Many researchers (Jerlov, 1976) and advanced hobbyists (such as Joshi, 2013) have used microwatts to report light intensity. This is perfectly acceptable if we are concerned only with energy produced by a particular light source. However, ‘microwatts’ is a radiometric standard that reports the energy of light according to wavelength – blue light is more energetic than red light. Where photosynthesis is concerned, the energy of a particular wavelength does not matter – the ‘color’ or energy level of a photon is irrelevant where photosynthesis is concerned. If a blue photon is absorbed by a photopigment (chlorophyll, peridinin, etc.), it will promote photosynthesis only as well a ‘red’ photon. Therefore, radiometric information, when plugged into our formulae for use in photosynthesis experiments, is not suitable. We should use another standard, and that is Photosynthetically Active Radiation (PAR). See Kirk (2000) for details. This presents another problem – PAR, or quantum meters, report wavelengths ranging from 400 to ~700nm. Hence I have elected to use quanta measurements to report energy in the range of 400nm to 700nm.
Sunlight Quality
To the human eye, the spectral quality of sunlight might appear to vary little on days of similar weather conditions. However, scientific instruments can detect small but significant changes in light quality. These variations can be due to atmospheric oxygen content (which decreases with elevation), ozone level (often seasonal), and water vapor content (water and water vapor preferentially absorbs wavelengths towards the red portion of the spectrum.) In addition, unusual events, such as volcanic eruptions (that add carbon dioxide, sulfur dioxide, and acids) can also affect light quality (this is the case here on the Big Island of Hawaii – the Kilauea volcano has been in a constant state of eruption for decades.)
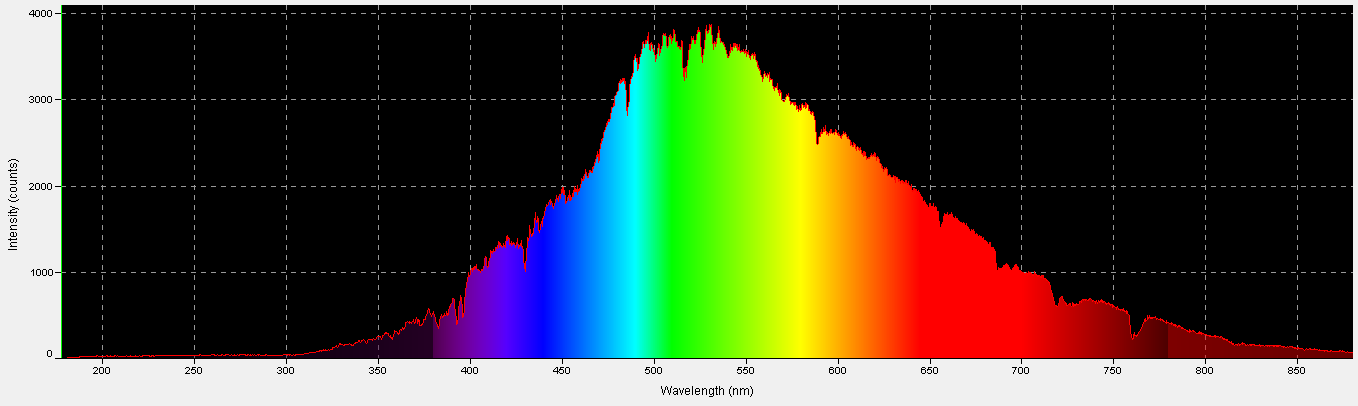
Figure 1. Sunlight quality under these conditions – 12:15pm, November 18, 2013, clear sky, 10 feet above sea level, Winds NNW @ 16mph, Humidity = 53%, Visibility = 10 miles. Vog (volcanic emission and smoke) = light. Kailua-Kona, Hawaii (19 39′ 0″ N, 155 59′ 39″ W)
The human eye is better at detecting sunlight quality when it is affected by the amount of cloudiness. Particulate matter in the air also affects apparent sunlight color (this is vividly demonstrated during sunsets, after winds have carried dusts and smoke into the atmosphere during the day.) The time of year also plays an important part, as at higher latitudes sunlight travels through more of the atmosphere, due to solar elevation. It becomes obvious that many factors affect the true color of sunlight. For these reasons, the time of year, location, and sky conditions (along with any extenuating circumstances) should be reported for reference solar data. See Figure 1.
Color | % |
---|---|
Violet | 4.98 |
Blue | 13.85 |
Green-Blue | 4.49 |
Blue-Green | 9.95 |
Green | 9.98 |
Yellow-Green | 19.25 |
Yellow | 4.13 |
Orange | 7.66 |
Red | 25.71 |
Light Absorption by Pure Water
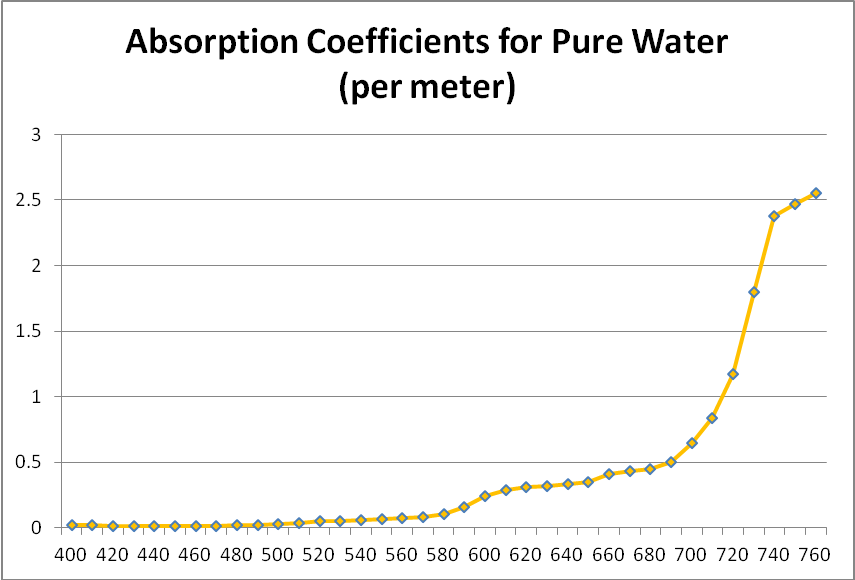
Figure 4. Attenuation coefficients of pure water – violet, blue and green is only weakly absorbed, while red is rapidly attenuated.
We generally think of pure water as a clear liquid when it is, in fact, a blue liquid – it absorbs blue wavelengths weakly but begins to absorb light more strongly at wavelengths above ~550nm. It significantly absorbs red light (about 35% of red light (680nm) is absorbed by a 1-meter deep column of pure water.) See Figure 4 for absorption coefficients.
Seawater, of course, is highly contaminated with a number of salts, metals, suspended solids, dissolved solids, and phytoplankton. The latter three of these can significantly alter the transmission qualities of seawater.
Classifications of Seawater Based on Optical Qualities
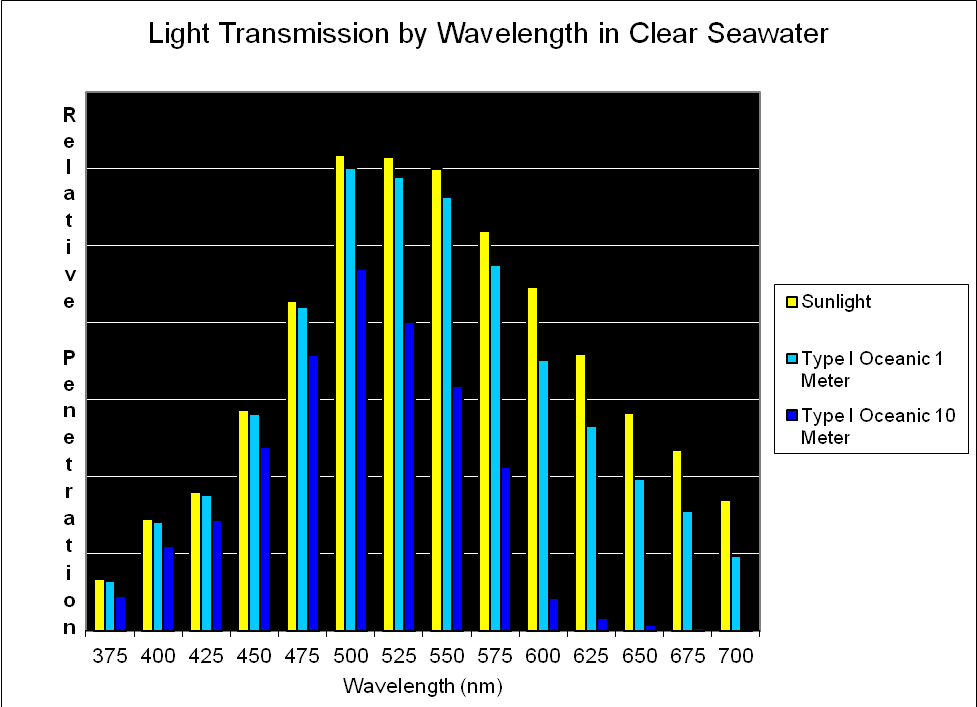
Figure 5. Jerlov reports spectral qualities in 25nm increments. Later in this article, we’ll refine this information in this article and use 10nm increments.
Jerlov (1976) classified seawater into two categories – Oceanic and Coastal. These categories are based on factors that cause attenuation (weakening) of light by absorption or scattering caused by dissolved substances or suspended solids. Oceanic water is further divided into 5 subgroups (Types I, IA, IB, II and III). Coastal water also has five subgroups (Types 1, 3, 5, 7, and 9). In both cases, the higher the number associated with a type of water, the less transparent it is. Oceanic types are the ‘clearest’ and contain few suspended solids, or dissolved substances that lend a yellow or red appearance. On the other hand, Coastal waters are often, if not constantly, under the influence of runoff (rivers, rainfall) from land masses and may have high turbidity and/or color. To make a very general statement, Oceanic waters might be considered clear and blue, while Coastal waters are cloudy and might be yellow due to dissolved organic matter or greenish due to high populations of phytoplankton.
Jerlov states that the waters around Hawaii are classified as Oceanic Type I; however, the near-shore waters along the Kona coast are undoubtedly influenced by inflow of rain water that has fallen upon beds of decaying vegetation, and then filters through volcanic rock. The shallows might also contain various types of green, brown and red algae along with cyanobacteria. There is little doubt that the waters off shore of Kailua-Kona (Big Island of Hawaii) are ‘Oceanic’. Calculations I performed for this article show water clarity (see Figure 11) are less than Oceanic Type I (an educated guess suggests perhaps Type 1A or 1B classification.)
See Figures 5 – 9 for approximate percentages of light (by color in 25nm increments as reported by Jerlov) at 1 and 10 meter depths for the clearest seawater and the dirtiest, respectively.
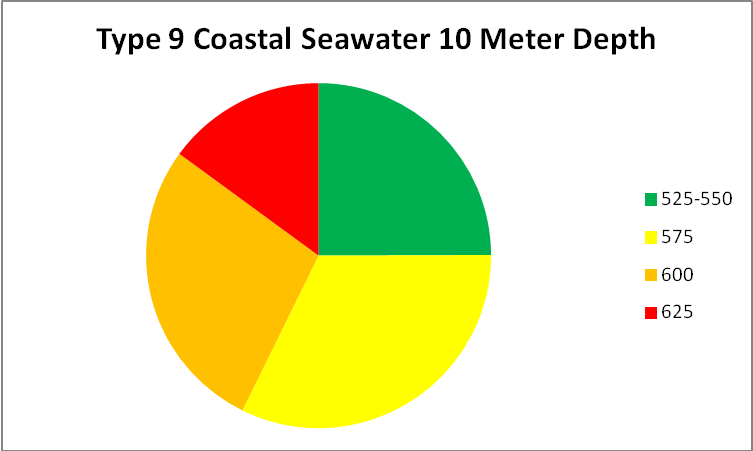
Figure 9. Worst case light quality – Type 9 Coastal Water (in non-scientific parlance, really crappy water.) Yellow water color, phytoplankton, and turbidity have absorbed or scatter all wavelengths below ~525nm and above ~625nm.
Method of Calculating Light Transmission – Jerlov Type I Seawater
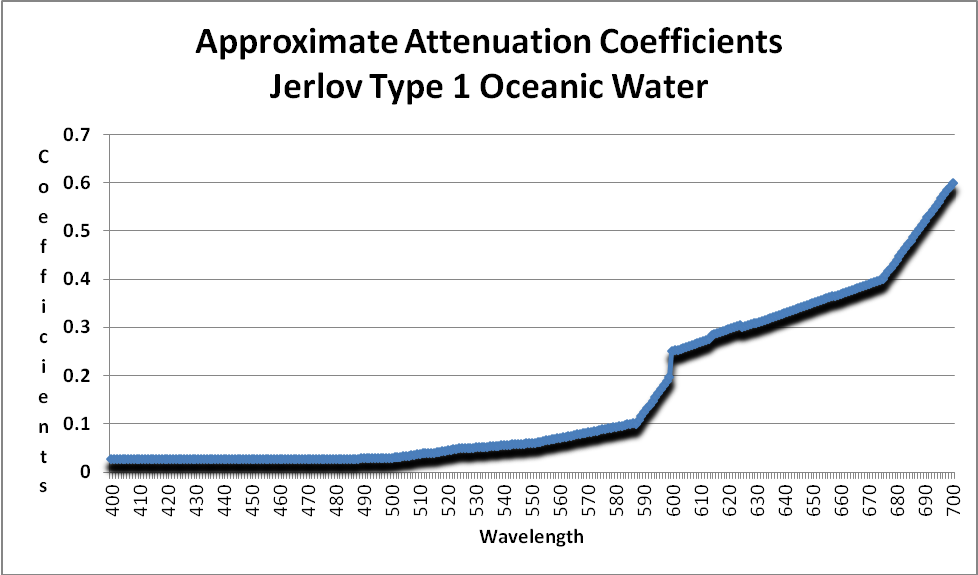
Figure 10. Attenuation coefficients of Mediterranean seawater. These are used to estimate light attenuation on a Hawaiian reef. After Bradner.
Calculations of light transmission through a particular classification can be made if we know how light is absorbed (or scattered) in a particular classification of seawater. For the purposes of this article, I have elected to use the attenuation coefficients of the most transparent of seawaters – Type I Oceanic.
As Figure 10 shows, absorption of light is minimal at wavelengths of 400 -500 (violet – blue-green) and becomes significant at about 550nm. Orange and red wavelengths are strongly absorbed. This water is classified as Jerlov Type I Oceanic water and was collected at depth in the eastern Mediterranean, where suspended particles (of about 1 micron in diameter) are present in concentrations of ~0.01 mg/liter. In short, this water’s clarity is comparable to distilled water.

Figure 11. Approximate light quality at 10 feet on a sunny July day in Hawaii (Honaunau, Big Island of Hawaii).
Using these coefficients and the sunlight data in Figure 1, we can estimate the spectral quality of light at a depth of 10 feet on a natural Hawaiian reef. See Figure 11.
Quantity of Light Transmitted Through Oceanic Seawater
Our discussion so far has examined factors that can affect both the quality and quantity of light through clear seawater. Quantifying the amount of light is relatively simple though not particularly easy. I am using data I collected some years ago using the following protocol. SCUBA dives were made at Honaunau (City of Refuge, Big Island of Hawaii) at noon in July. Light intensities were made with an Apogee quantum meter (housed in a custom-built waterproof housing.) A BNC connector on the sensor cable allowed it to be removed from the meter and ‘snaked’ through a small bulkhead fitting on the housing. In this manner, the meter remained protected within the housing while the sensor was outside and could be positioned to gather down welling or upwelling light. Water depth was estimated by an Oceanic dive computer. Results were recorded on a dive slate with a pencil. PAR values were recorded on sunny (low vog) and cloudy days. Figures 12 and 13 shows these results.
Interestingly, the summer time cloudy day sunlight intensity is about that seen in Hawaii on a clear winter day. Hence, we have data to estimate light transmission for summer and winter days.
Estimating Light Intensity at Depth Using Maximum (Noon) PAR Values
Since deploying data loggers at depth presented too many obstacles, we will use a simple method to estimate light intensity at depth. This method is obviously not perfect. Maximum PAR values were entered into Excel and a photoperiod was chosen from Figure 17 (in this case, 1300 µmol·m²·sec at noon on a clear day at a depth of 10.5 feet). See Figures 14 and 15.
Calculating Spectral Quality at Various Depths
So far, we have defined sunlight spectral quality, attenuation coefficients for Jerlov Oceanic Type I sea water and summer time light intensity of sunlight (sunny and cloudy) transmitted through some of the clearest seawater in the world. With this information, we can estimate the quality of light at depths up to 40 feet.
Length of Day – Photoperiod
Unless we live at the equator, we should expect the length of day to vary throughout the year. A 12-hour day (photoperiod) at the equator is fairly consistent, while 13 hour photoperiods during the summer at 20 north or south are normal (with days of only ~10.75 hours during the winter). Figures 16 and 17 show monthly photoperiods for latitudes of 10 and 20 north and south of the equator.
Although coral reefs are found to extreme latitudes of 30 north and south, these data will allow us to calculate photoperiods for most of the world’s reefs. But we aren’t through yet. Next we’ll examine how to calculate the total amount of light on a given surface, or the:
Daily Light Integral
The Daily Light Integral is the total amount of light (or Photosynthetic Photon Flux Density, or PPFD) falling on a given area during the entire photoperiod. The best comparison is to compare PPFD to rainfall. An instantaneous PPFD value (the number of photons falling on a given surface area in a given amount of time) is equivalent to the number of raindrops falling on an equal area and time. We would find a weather report presenting rainfall as number of raindrops falling on a square meter per second to be practically useless. We are more interested in the total amount of rainfall reported in inches or centimeters. The same is true for light – we should be interested in the total number of photons in a given photoperiod.
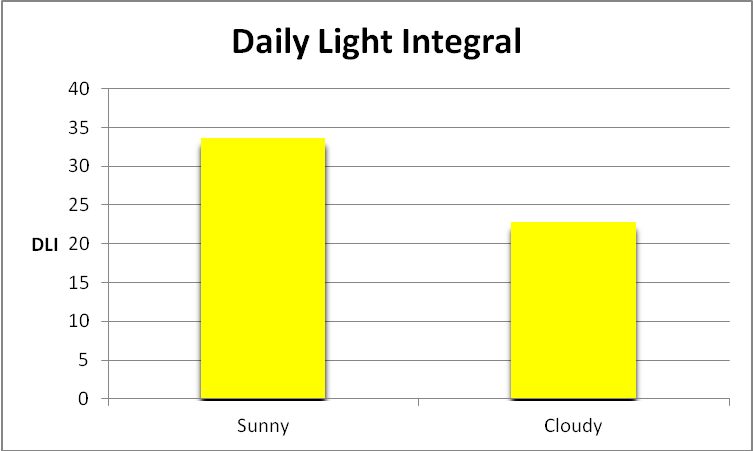
Figure 18. Total light dosage in a photoperiod, or Daily Light Integral (DLI), in mol photons on a clear sunny day in Hawaii at a depth of 10 feet.
DLI is calculated as follows. We take an instantaneous PAR value (in µmol photons·m²·sec) and multiply it by the number of seconds in the chosen photoperiod. This will give us a rather unwieldy number, so we divide by 1,000,000 to arrive at mol photons per square meter per photoperiod. Based on the info we have, we can calculate summer DLI values (summer on clear and cloudy days) for a Hawaiian reef:
- Summer DLI (sunny) – 10 feet – 33.7, or an average of 780 µmol photons·m²·sec
- Summer DLI (cloudy) – 10 feet – 22.9, or an average of 511 µmol photons·m²·sec
See Figure 18.
Since we know the attenuation coefficients for light wavelengths, we can also calculate DLI for wavelengths or color bandwidths.
Further experiments are needed if we want to compare attenuation factors of ocean water to a reef aquarium. Back to the lab we go.
Comparison of Hawaiian Seawater and Aquarium Water
Someone once said, and I believe it was Charles Delbeek, that ‘an aquarium is not the ocean.’ An aquarium is subject to dynamics unimaginable in the ocean. Therefore I felt it prudent to look at water quality from both an aquarium and a real reef. Water samples from an aquarium and a Hawaiian reef were gathered in analyzed for pH, true and apparent color, turbidity, and suspended solids (volatile – or organic – and inorganic).
Color of Water and Light Absorption by Yellow Substances (Gelbstoff, Gilvin)
Unless corrective measures are taken, aquarium water will accumulate dissolved substances that lend a yellow tint. These yellow substances are often due to decaying algae – brown algae in particular – and go by various names (gelbstoff and gilvin are two commonly used.)
These yellow substances are known to preferentially absorb ultraviolet, violet, and blue light.
Gelbstoff/gilvin can be controlled through judicious use of activated carbon and/or ozone. Routine water changes can also help.
Standard Methods for the Examination of Water and Wastewater (1998) describes the following methods for determining true and apparent color of water. Apparent Color describes color of an unfiltered sample, where suspended matter affects the measurement. True Color is determined after this matter is removed by filtration. Color can be judged visually using standards (a platinum/cobalt standard where 1 mg/l KPtCl6/CoCl2·6H2O = 1 PtCo Color Unit, with platinum as chloroplatinate ion), or by a spectrophotometer (in this case, using light at 455nm and measuring transmission through 2.5 cm of water).
True and Apparent Color of Water Samples

Figure 19. Aged water from a reef aquarium had much higher color (‘yellowness’) than water from a Hawaiian reef.
Water samples were gathered from an aquarium and a Hawaiian reef. The aquarium had gone a few months without a water change and used a refugium filled with Chaetomorpha alga and a downdraft protein skimmer for filtration. Small amounts of an unidentified brown alga grew in the aquarium and provided a natural food for a single Yellow Tang (Zebrasoma flavescens). The sample from the reef was gathered at dusk of a day where the ocean was relatively calm. Hence, the aquarium sample could be considered as being towards ‘worst case’ and the reef sample ‘best case.’ These samples were taken to the lab and analyzed for ‘apparent color’ (samples were not filtered). ‘True color’ (after the sample was filtered through a 0.45 micron filter) was determined on the aquarium sample only.
Visually, the aquarium sample had a yellow tint to it while the reef sample appeared clear. A spectrophotometer (with wavelength set to 455nm) analyzed the sample. Figures 19 and 20 show the results.
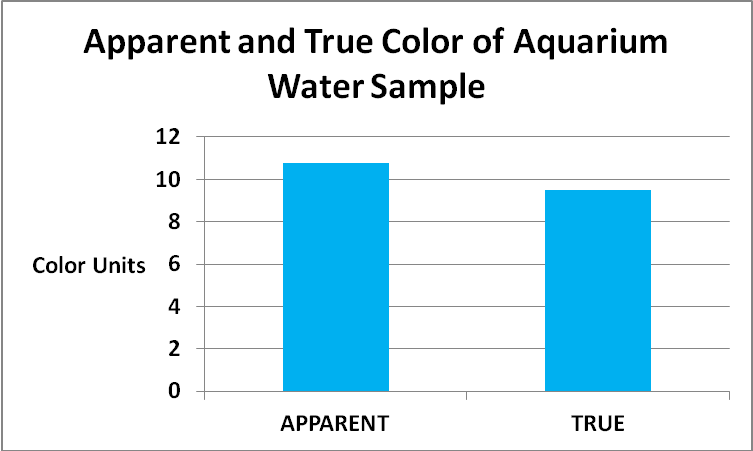
Figure 20. Refinement of data presented in Figure 19. Most of the color of the aquarium water sample is due to dissolved substances. See text for details.
The color of water invariably increases with higher pH values and Standard Methods states pH should be reported along with the color value. So, the aquarium sample pH was 8.2, and the reef sample was 8.12.
Methods for Determining Color of Water
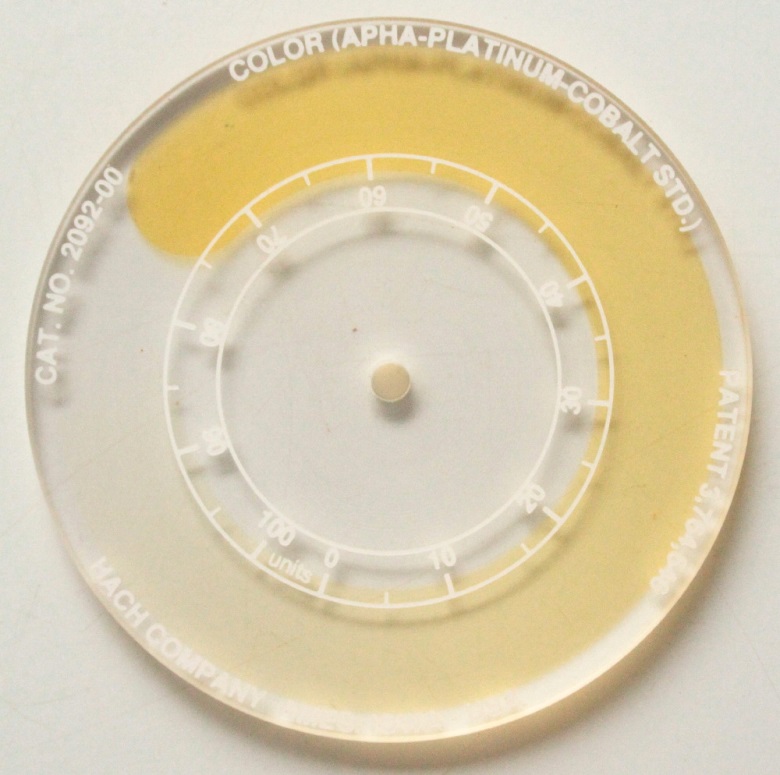
Figure 21. This disk, when inserted into a comparator, allows visual estimation of the color of water.
Several methods are available for the determination of water color. Two are visual. The least expensive method involves a laminated card with sections colored pure white and yellow (such as those marketed some years ago by the German firm Dupla GmbH). It is qualitative, not quantitative. This card is dipped into the aquarium and a water change is called for if the white section appears yellow (Note: The card marketed by Dupla was apparently made for use in freshwater aquaria only, as the yellow reference indicated a water color much too high for a reef aquarium). The second visual method involved a comparator, where the color of a sample in a tube is matched to another sample behind a rotating disk with reference colors printed on it. See Figure 21. The best method for determination involves either a colorimeter (see Figure 22) or a spectrophotometer. These instruments are able to compare the transmittance of light at a given wavelength through a sample and a blank. Results are reported digitally.
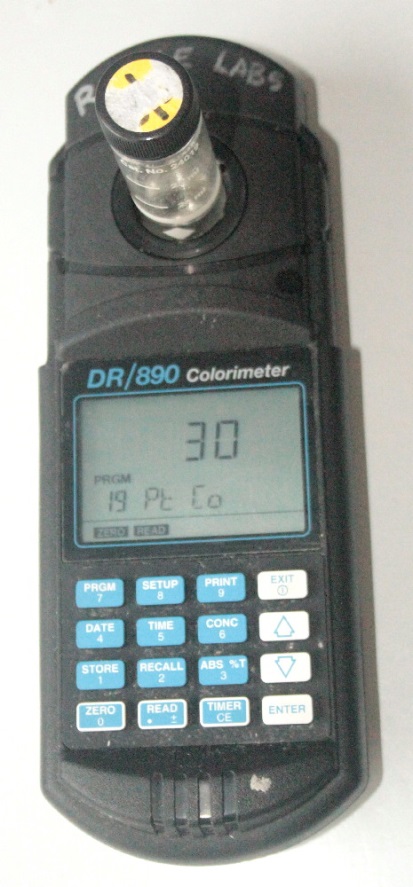
Figure 22. Many colorimeters (such as the one above) and spectrophotometers can estimate the color of water.
Light Absorption by Tripton

Figure 23. Total suspended solids minus volatile solids equals an estimate of the inorganic content.
Tripton is defined as ‘suspended non-living matter in a water column.’ This definition leaves a good deal of latitude in interpreting its true meaning. An examination of both water samples revealed unidentified detrital particles, none of which appear alive. For the purposes of this article, non-living material is defined as non-volatile suspended solids. This requires determination of total suspended solids and volatile total suspended solids. See Figures 23-25.

Figure 24. Suspended solids (those retained on a filter with a pore size of 1.5 microns) in an aquarium and a natural reef.
Determination of Suspended Solids
The protocol for determining total suspended solids and volatile suspended solids was as follows. Known volumes of the samples were filter through pre-combusted and pre-weighed glass fiber filters with a pore size of 1.5 microns. These were dried at 103C for one hour. Filters were weighed once again and values were plugged into this formula:
a-b / sample volume (ml)*1,000 where: a = filter + retained solids weight (mg), and b = filter weight (mg)
Volatile solids were determined in this manner: The filters were placed in a muffle furnace with at a temperature of 550C for 20 minutes. After cooling for a few minutes in a desiccator, the filter was weighed, and this formula used.
a-c / sample volume (ml)*1,000 where: a = filter + retained solids weight (mg), and c = filter + filter retained solids weight after combustion (mg)
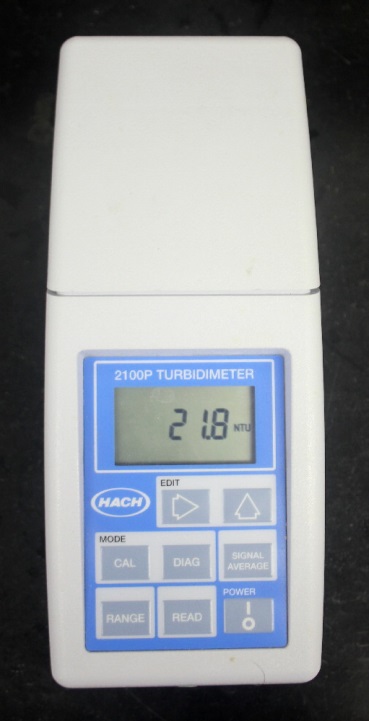
Figure 26. An instrument called a turbidimeter measures how much light is scattered by suspended particles.
Light Absorption by Phytoplankton
Many natural waters contain at least some phytoplankton (algae, diatoms, etc.) The photopigments within these organisms absorb various light wavelengths to power photosynthesis, thus altering the optical quality of the water. In extreme cases, the water may appear green (algae) or brown (diatoms) due to absorption and reflectance of light.
Light Scattering by Suspended Particles (Turbidity)
Turbidity is defined as ‘the optical property that causes light to be scattered or absorbed by a particle rather than transmitted’. In other words, turbidity is a measurement of how much light is scattered by suspended particles. Many years ago, turbidity was determined visually using a cylinder of water and a candle. This method was reported in Jackson Turbidity Units, or JTU. Advances in miniaturized electronics now allow turbidity to be determined with a photometer called a turbidimeter and reported in units of NTU (or nephelometric turbidity units). See Figure 26.
Determination of Turbidity and NTU
An electronic turbidimeter was used to estimate water turbidity. The instrument was calibrated to standards of known turbidity.
The results of these tests are shown in Figure 27. Since the suspended solids in the seawater sample were higher than that in the aquarium water sample, it is not surprising that the turbidity measurement was higher as well.

Figure 27. Turbidity, or scattering of light caused by suspended particles, in an aquarium and water collected from a Hawaiian reef.
Effects of Salts Found in Seawater on Absorption of Light
Kirk (2000) states that salts found in seawater do not appear to have any significant effect on absorption of light.
Comparison of Sunlight (10′ Depth) and Light from a LED Fixture
Comparisons of spectral data of sunlight at depth on a reef and artificial light in an aquarium allows us to begin to understand why some corals thrive and generate colors (both fluorescent and non-fluorescent) thought to protect zooxanthellae from excessive light at very low light levels in an aquarium.
Figures 28 and 29 & Table 2 show a breakout of seawater spectral qualities.
Bandwidth | % | Bandwidth | % | Bandwidth | % |
---|---|---|---|---|---|
400-410 | 2.10% | 501-510 | 6.52% | 601-610 | 1.95% |
411-420 | 2.34% | 511-520 | 6.16% | 611-620 | 1.67% |
421-430 | 2.33% | 521-530 | 6.27% | 621-630 | 1.45% |
431-440 | 2.83% | 531-540 | 6.04% | 631-640 | 1.22% |
441-450 | 3.34% | 541-550 | 5.84% | 641-650 | 1.00% |
451-460 | 3.52% | 551-560 | 5.34% | 651-660 | 0.83% |
461-470 | 4.10% | 561-570 | 4.75% | 661-670 | 0.71% |
471-480 | 5.28% | 571-580 | 4.34% | 671-680 | 0.55% |
481-490 | 5.88% | 581-590 | 3.78% | 681-690 | 0.30% |
491-500 | 6.49% | 591-600 | 2.91% | 691-700 | 0.17% |
The LED luminaire is from Build My LED (www.buildmyled.com), a Texas-based company that builds LED fixtures to the specifications of the customer. The LED used for the experiments in this article is a 48″ strip housing sixty LEDs generating light peaking at 450nm and 470nm as well as broad spectrum white light rated at 4500 Kelvin. The sequence of LEDs in this luminaire is 450nm – 470nm – 4500K – 4500K. This sequence repeats 15 times for a total of 60 LEDs in a 48 inch strip.
The spectral data presented here was gathered at a depth of 8 inches in an aquarium. The color of the water is considerably higher than ocean water (see Figure 19) but turbidity is less (see Figure 27).
Figures 31 – 33 & Table 3 show a breakout of the LEDs’ spectral qualities at a depth of 8″ (about half the depth of the average aquarium).
Bandwidth | % | Bandwidth | % | Bandwidth | % |
---|---|---|---|---|---|
400-410 | 0.197% | 501-510 | 2.310% | 601-610 | 2.097% |
411-420 | 0.387% | 511-520 | 2.721% | 611-620 | 1.807% |
421-430 | 1.234% | 521-530 | 3.110% | 621-630 | 1.534% |
431-440 | 4.373% | 531-540 | 3.317% | 631-640 | 1.178% |
441-450 | 12.255% | 541-550 | 3.397% | 641-650 | 0.922% |
451-460 | 16.151% | 551-560 | 3.308% | 651-660 | 0.714% |
461-470 | 12.520% | 561-570 | 3.126% | 661-670 | 0.527% |
471-480 | 7.486% | 571-580 | 2.917% | 671-680 | 0.387% |
481-490 | 3.943% | 581-590 | 2.696% | 681-690 | 0.298% |
491-500 | 2.470% | 591-600 | 2.412% | 691-700 | 0.208% |
Comparison of Daily Light Integrals
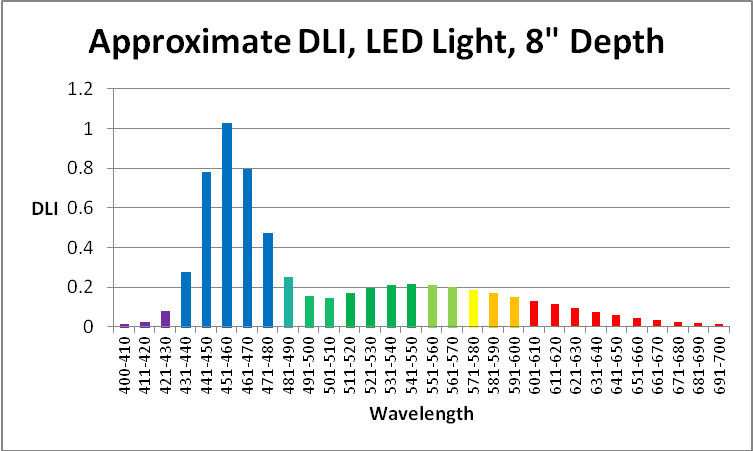
Figure 33. Aquarium light DLI (mol photons per photoperiod) generated by LEDs at 8″ depth in an aquarium. PAR is 150µmol·m²·sec and the photoperiod is 12 hours.
Based on the information present above, and using a photoperiod of 12 hours, we can calculate the Daily Light Integral (DLI – the total dose of light in a given photoperiod).
It is important to remember that the light intensity of the LED strip is relatively low. If the PAR value were to increase to 300 µmol·m²·sec, many corals’ zooxanthellae would be at their photosynthetic saturation point, and increasing the amount of light might be detrimental. Note that blue wavelengths (431 – 471nm) at a PAR value of 300 would exceed those seen by a coral at 10 feet in clear water during the summer.
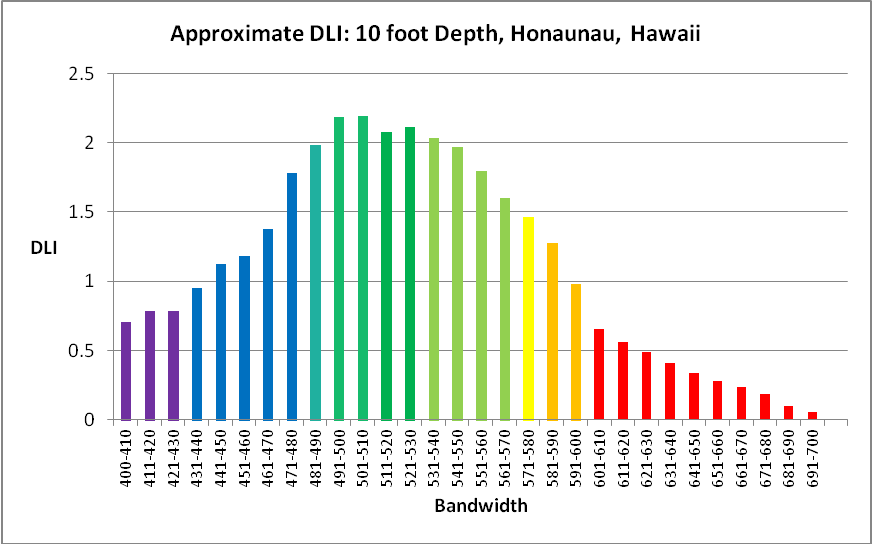
Figure 34. Approximate DLI per bandwidth found on the reef at Honaunau, Hawaii. Photoperiod is 12 hours and varying light intensity used is shown in Figure 14.
Discussion
Many factors must be considered when estimating light intensity and quality at depth. The quality of sunlight must be considered, as well as sky conditions, as well as amount of aerosols and particulates (as estimated by visibility or air transparency). The quantity of light depends upon these conditions in addition to seasonal changes. Turbidity and true color of water are factors that affect light transmission once light enters a water column. In short, estimating the quantity of light is fairly easy, but calculating light quality at depth, without sophisticated instrumentation and personnel with time and training to monitor these computer-driven devices, becomes an educated guestimate. And this is certainly the story here. Our estimates are based on data gathered from various reference sources meshed with field information garnered by a spectrometer and quantum meter in an underwater housing here in Hawaii. At best, it is fuzzy science – but is suitable for our purposes. We should not be overly concerned that this information is not universal and absolute. The challenges of comparing aged aquarium water to that sampled from a Hawaiian reef required further data collection involving analyses of total and volatile suspended solids, color of water, and turbidity. Based on the information presented in this article, aquarium water can be less turbid but contain dissolved and suspended particles that lend to a higher value of apparent and true water color than that of water from the real reef. Under different circumstances, where an aquarium’s water quality is maintained with activated carbon, judicious use of ozone, and regular water changes, the situation with color could be reversed. The same might apply to turbidity caused by suspended particulate matter. In the case presented here, aquarium water had fewer suspended solids. Realizing that conditions in an aquarium and a natural reef can be highly variable should not prevent us from learning from the lessons these data present.
Aged, yellow water is known to absorb some ultraviolet energy and violet & blue wavelengths. Further testing is needed to quantify the amount of light energy absorbed.
The total light dosage on a natural reef can be determined at depths up to 40 feet with the information presented here. The standard I will use in future articles will be that present on a natural reef at a depth of 10 feet during a clear, summer (July) day in Hawaii (City of Refuge, or Honaunau, Big Island of Hawaii). The water column would have low suspended solids (turbidity) and water color.
The total light dosage, or DLI, under these conditions would be:
- Summer DLI (sunny) @10 feet = 33.7, or an average of 780 µmol photons·m²·sec in a 12-hour photoperiod
- Summer DLI (cloudy)@10 feet = 22.9, or an average of 511 µmol photons·m²·sec in a 12-hour photoperiod
Obtaining light intensity of 511 or 780 µmol photons·m²·sec (light levels needed to reach a DLI of 33.7 or 22.9, respectively, in an aquarium lighted for 12 hours) is certainly possible, but is it desirable? Most likely it is not, based on PAM fluorometer experiments (the PAM fluorometer is a ‘photosynthesis meter’ that estimates rates of electron transport between photosystems through measurements of chlorophyll fluorescence). The information I have gathered so far suggests most corals require only ~400 µmol photons·m²·sec or less in order to reach a maximum rate of photosynthesis (the saturation point). Some corals, such as Montipora capitata, reach a maximum rate of photosynthesis at only ~135 µmol photons·m²·sec, while a Tridacna derasa clam did not reach saturation at light levels of about 550 µmol photons·m²·sec. See here for details: http://www.advancedaquarist.com/2007/3/aafeature1
Clearly, more experiments are needed here as well. The determination of the maximum amount of light required for photosynthesis by deeper water corals (such as 10 feet) is not something I have explored.
Of more interest to me, however, is the information presented here concerning the spectral quality of sunlight at a depth of 10 feet. Unless, I have a compelling reason to do so, this will become the standard I will use when comparing light on a real reef to light in an aquarium.
There are intriguing reports of hobbyists and commercial coral farms growing brilliantly colored corals under extremely low light levels. At present, the hypothesis is that the relatively high amount of blue light at relatively low light intensity (PAR) is the environmental trigger for not only rapid growth but extreme coloration as well. This flies in the face of opinions of many hobbyists, where the thought is that corals, or more correctly, their zooxanthellae, are infinitely adaptable to higher amounts of PAR (the ‘more is better’ train of thought). Experiments are planned to examine photosynthetic efficiencies of LEDs producing differently colored light. In addition, I have new information about blue light and its fate when promoting photosynthesis (or not) in corals’ zooxanthellae. But that is a discussion for another time.
Researchers have, in the last ten years or so, determined the wavelengths needed to induce coloration in corals. We can quantify the amount of light per wavelength or bandwidths and determine the suitability of a particular light source in promoting these colors.
While I have put a great deal of time and effort in writing this article, and consolidated data I have collected on natural reefs in Hawaii over the last 14 years, the information presented here is but a foundation for further research.
References
- American Public Health Association, 1998. Standard Methods for the Examination of Water and Wastewater. United Book Press, Maryland.
- Bradner, H., undated. Attenuation of light in clear deep ocean water.
- Hunt, R., 1998. Measuring Colour. Fountain Press, England. 344pp.
- Jerlov, N., 1976. Marine Optics. Elsevier Press, Amsterdam.
- Joshi, S., 2013. Equipment Review: LED Lighting Tests: Ecotech radion Pro, Aqua Illumination Hydra, GHL Mitras 6100HV and 6200HV. http://www.advancedaquarist.com/2013/8/review
- Kirk, J., 2000. Light and Photosynthesis in Aquatic Ecosystems. Cambridge University Press, Cambridge, UK. 509 pp.
I have a guesting Dana Riddle, First of all…thank you for sharing about reef aquarium lighting. I have been studying and learning a lot over the past month because we, not long ago, started adding corals to our fish-only tank. When programing the lighting, such as LED lighting, what intensity of each spectrum should we use? Example, at my peak levels, I have B, RB, UV, and V at 100%, all whites at 40%, and red and green at 10%. Should the white, red, and green have a higher level?
I have a guesting Dana Riddle, First of all…thank you for sharing about reef aquarium lighting. I have been studying and learning a lot over the past month because we, not long ago, started adding corals to our fish-only tank. When programing the lighting, such as LED lighting, what intensity of each spectrum should we use? Example, at my peak levels, I have B, RB, UV, and V at 100%, all whites at 40%, and red and green at 10%. Should the white, red, and green have a higher level?
Epic article!