The aquaculture and husbandry of exotic marine species is ever evolving, with breakthroughs occurring regularly. Currently, marine invertebrates that completely rely on heterotrophic feeding are not easily maintained in closed aquarium systems. Despite their husbandry difficulties, large quantities of these organisms are exported to Western countries annually due to high demand from the aquarium trade. As marine ecosystems are currently in decline, there is a growing incentive to aquaculture these commercially interesting animals. In part one of this article I will discuss the innovative filtration technology called DyMiCo (Dynamic Mineral Control) that allows aquarists to culture a rich diversity of marine organisms, especially those that rely on live plankton for their survival.
Coral reefs are of high ecological and economic importance, and are currently in global decline (Hughes et al. 2003; Hoegh-Guldberg et al. 2007). This is caused by global climate change and local anthropogenic disturbances, including pollution, coastal development and overfishing (Hughes et al. 2003). Overfishing is a threat to local reefs in Asia, for example in Indonesia and the Philippines, where reef organisms are collected for the international aquarium trade (Wabnitz et al. 2003). Fueled by a high demand for tropical species as home ornamentals, this trade is not sustainable as only a subset of exported animals is produced by aquaculture or mariculture. As the international aquarium trade may aggravate the detrimental effects of climate change and other human disturbances, there is a growing incentive to sustainably aquaculture reef organisms. Important candidates for culture include sponges and corals, as they are used as ornamentals and are becoming increasingly interesting for the pharmaceutical industry. A major caveat however is knowledge of their husbandry, which is still limited for many species. This impedes successful aquaculture of these invertebrates.
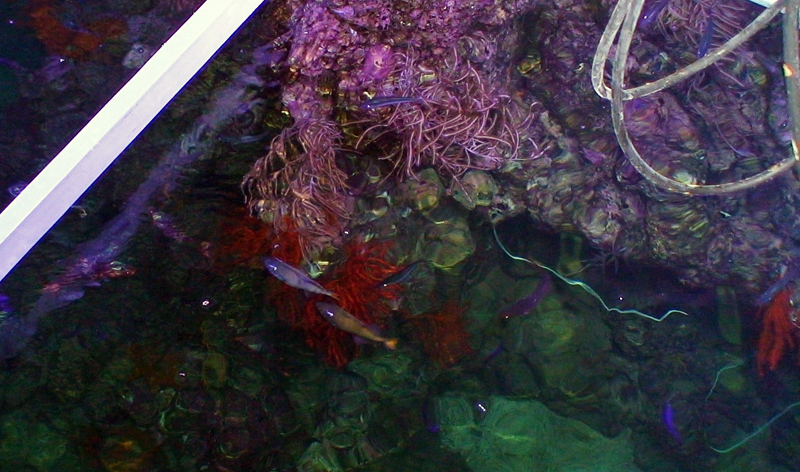
A DyMiCo system at Rotterdam Zoo (The Netherlands) housing Caribbean corals in the main display aquarium, and indo-pacific corals in the filter compartment. Note the flourishing red gorgonians (Swiftia exserta), which completely rely on plankton and dissolved nutrients for their survival.
To optimize the aquaculture of any species, detailed knowledge of factors affecting growth is of high importance. Today, several major factors for aquaculture of marine invertebrates have been identified, including light, water flow, water quality and nutrition (reviewed for corals by Osinga et al. 2011). The irradiance and water flow the average reef organism is exposed to can be mimicked quite effectively with modern technology. The same goes for water quality, although inorganic nitrogen and phosphorous concentrations usually are an order of magnitude higher than those found on a pristine reef. Nutrition, more specifically in the form of plankton, remains a major issue in most closed systems. Either the proper feeds are unavailable to the aquarist, or the amounts of feed required make it difficult to maintain water quality. In part one of this article I would like to present a filtration system that allows the aquarist to maintain a live plankton population in water of high quality, which will significantly improve the aquaculture of many invertebrate species.
Heterotrophic animals and plankton
Before I discuss the DyMiCo technology, I would like to establish that maintaining a vibrant plankton population in the aquarium is essential for the aquaculture of many organisms, especially those that are completely heterotrophic. What exactly are heterotrophic animals, and why are they sometimes difficult to care for?
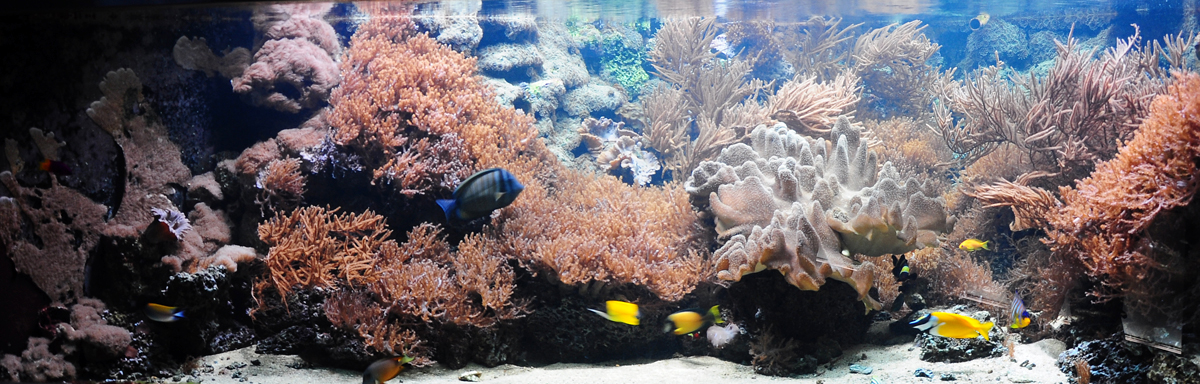
Another system at Antwerp Zoo (Belgium) dominated by octocorals, which also holds surgeon- and rabbitfishes, wrasses and Tridacna clams.
Based on metabolism, life on earth can be roughly divided into two groups; autotrophic and heterotrophic organisms. Autotrophic organisms are those that use inorganic molecules such as carbon dioxide (CO2) to build organic molecules, including carbohydrates, alcohols, fatty acids and amino acids. I use the term organic here for molecules that have a carbon chain, or “backbone””. Glucose, a simple carbohydrate, contains a chain of six carbon atoms and is highly important to life on earth because of the chemical energy it contains. Common examples of autotrophic organisms are plants, including unicellular algae, and cyanobacteria. These organisms are considered photoautotrophic as they use the sun’s energy to convert CO2 and bicarbonate (HCO3–) to organic molecules, which they use to fuel their metabolism and grow. Autotrophic organisms are also called primary producers, as they represent the first step in the food chain that leads to biomass production from inorganic molecules. Heterotrophic organisms are those that cannot produce their own organic molecules from inorganic ones (although they can interconvert organic molecules), and therefore have to acquire these from their environment. Organic nutrient acquisition may vary from direct uptake from a solution such as seawater, to ingestion and digestion of other organisms. Common examples of heterotrophic organisms are herbivores or plant-eating animals, such as cows or sea urchins. These organisms are called primary consumers. Examples of heterotrophs that of relevance to this article are certain sponges, corals and echinoderms. Reef-building corals however, like many other reef organisms, host symbiotic dinoflagellate algae (zooxanthellae) in their tissues, arguably making them polytrophic as they have both autotrophic (photosynthesis) and heterotrophic (plankton and organic matter uptake) feeding modes.
As heterotrophs such as marine invertebrates require some form of nutrition to meet their metabolic energy demand, grow and reproduce, the aquarist will have to supply this in one form or another. In many cases this is no issue, as many high quality commercial feeds are available nowadays. Fishes for example often thrive in aquaculture or in the aquarium as a wide range of appropriate feeds can be provided. Most reef corals have formed a mutualistic symbiosis with zooxanthellae, which supply their host with significant amounts of organic carbon. These corals usually grow well in the average aquarium. Animals that however rely on specific food sources that are insufficiently available in a closed system fail to grow, and eventually die. These animals are known as specialists (as opposed to generalists, which are able to use a wide range of nutrient sources), and they include a whole array of marine species. This brings us to plankton as a food source, which is essential for many organisms that are primary candidates for aquaculture. But what exactly is plankton?
Plankton, derived from the Greek word planktos or drifter, is a common name for an astoundingly large group of organisms that float around in a body of water. These include bacteria, algae and small animals such as crustaceans. Plankton is often divided into several classes based on particle size. The size classes relevant to this article are pico-, nano-, micro- and mesoplankton. Picoplankton consists of organisms having a size between 0.2 and 2 micrometers (m), such as cyanobacteria, archaea and protists; nanoplankton comprises organisms between 2 and 20 micrometers in size, including algae and larger protists; microplankton consists of small crustaceans such as rotifers, protozoa and larvae that range from 20 to 200 m in size; mesoplankton, finally, encompasses larger crustaceans and the larvae of many animals that are between 200 to 2000 m in size. Plankton as a whole is used as a major food source for many animals that live in water bodies, including the world’s oceans. Animals that feed on plankton are called filter and suspension feeders, as they filter or capture particles from the water in order to digest them. Filter feeders actively filter dissolved and suspended matter from the water by pumping water through filtration structures, and include zooplankton, tunicates, bivalves and sponges. Suspension feeders actively capture food particles from the water which travel in close proximity, by using tentacles. This strategy has been adopted by e.g. scleractinian corals, octocorals and crinoids.
Zooplankton was not considered to be an important food source for marine organisms for many years; it was believed concentrations on the reef were too low to have any significant role. In the meantime, more accurate estimations have been made, based on improved measuring techniques. The abundance of plankton can be significant, especially when nutrients such as nitrogen, phosphorous and iron are elevated. This leads to so-called phytoplankton blooms, on which many planktonic animals thrive. Phytoplankton, including cyanobacteria such as Synechococcus, can reach very high concentrations of up to a billion cells per liter and are a major food source for many invertebrates. Although the concentration of zooplankton in reef waters lies in the range of 0.007 and 4.4 individuals L-1 (Heidelberg et al., 2004, 2010; Palardy et al. 2006; Yahel et al. 2005), the presence of these small animals and their larvae is vital to the health of many filter and suspension feeding organisms. This is because they carry important elements such as nitrogen and phosphorus, which are required to synthesize new tissue for growth and injury repair. It is exactly these filtering and suspension feeding organisms that are hard to culture or maintain in any closed system, as small plankton, despite the myriad of products on the market today, usually is not available in sufficient quantities. This has led to dismal survival rates of such animals in the average aquarium. Well-known popular examples are sponges (e.g. Haliclona spp., Trikentrion flabelliforme), bivalves (Lima scabra), hydrozoans (e.g. Distichopora and Stylaster spp.), echinoderms such as crinoids (e.g. Comatula, Comanthus and Himerometra spp.) and octocorals (e.g. Dendronephthya spp. and gorgonians such as Menella, Diodogorgia and Swiftia spp.). Although relatively little is known about the feeding habits of these animals, large numbers are imported to Western countries where they live short lives as aquarium ornamentals.
Filtration – plankton’s Nemesis?
Although it is clear that many reef organisms require plankton to survive, the best way how to provide this is not. Most marine systems are closed off from the ocean and depend on a foam fractionator (protein skimmer) for high water quality. By mixing water and air using aspirator or eductor-jet pumps, fine air bubbles remove organic (waste) molecules from the system water that condense in collection beakers. This process works especially well in marine water due to its low surface tension, resulting in very fine air bubbles and thus efficient organic waste removal. Although foam fractionation has resulted in successful efforts to aquaculture fishes and invertebrates such as scleractinian corals, species that heavily rely on plankton have not fared well in systems using this technology. This may, to a great extent, be the result of the disruptive nature of foam fractionating. The problem seems obvious: the mechanical destruction and removal of small organisms that make up the plankton. Other filtration devices such as sand filters and biological filters, with their high water throughput, may have this problem, too. Indeed, Feldman et al. (2011) demonstrated that aquaria equipped with a foam fractionator contain significantly lower bacterial concentrations than reef waters, by approximately one order of magnitude. The question that follows from the above is this:
How can we maintain high water quality and a vibrant plankton population simultaneously, thereby mimicking nature?
Fortunately, a new emerging technology may be the answer to this question. This technology is called DyMiCo, short for Dynamic Mineral Control.
Maintaining living water – Dynamic Mineral Control
As foam fractionators, sand filters and densely packed biofilters used in most systems are not up to the task of sustaining the “plankton soup” many (in)vertebrate animals require, a different approach is required. Around the year 2000, a small company known as EcoDeco started working on a new concept that was based on a very old idea. By taking nature’s principles, more specifically the biogeochemical processes that occur in the oceans, the company wanted to do something new. Peter Henkemans, EcoDeco’s founder and CEO, set out to develop a novel filtration system that was to be simple, effective, and most of all, natural. The final goal was to use this system to cultivate endangered corals and other marine organisms. He coined and patented his technology as DyMiCo, or Dynamic Mineral Control. Now, twelve years later, several institutions are using EcoDeco’s technology with compelling results. With virtually no water changes and no mechanical filtration, these systems flourish. But how exactly does it work?
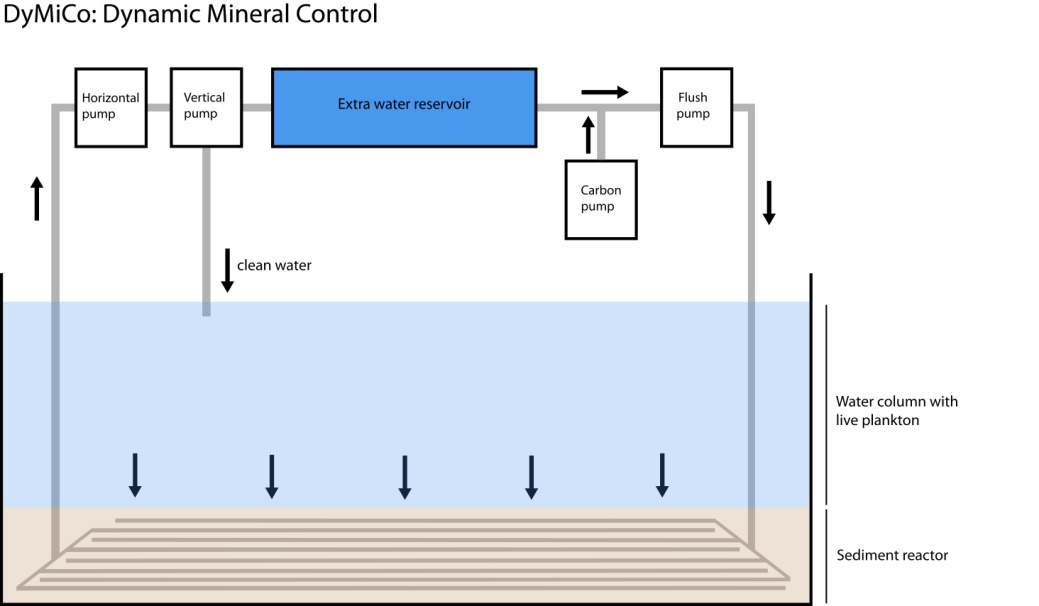
A schematic overview of the DyMiCo principle. A pull-through pump draws water through the reactor, and returns it to the water column after denitrification. A process pump ensures that carbon and CO2 are mixed homogeneously throughout the reactor, which are delivered by a carbon pump and CO2 bottle. The ORP and pH value of the reactor are controlled automatically by a computer, which dynamically adjusts the carbon, CO2 and water input, ensuring optimal denitrification rates. A flush pump can be used to back flush the system for cleaning purposes. Photograph by Tim Wijgerde
DyMiCo is based on the following principle: by using a denitrification reactor that dynamically (Dy) responds to the system, minerals (Mi) such as nitrate and phosphate can be controlled (Co). In more basic terms, this technology is computer-controlled denitrification reactor. DyMiCo monitors and controls the biogeochemical processes occurring in the reactor with two parameters: oxidation/reduction potential (ORP) and pH. These parameters are measured inside the reactor, by using the so-called interstitial water that flows through it. As the computer receives feedback from the ORP and pH probes in the reactor, it constantly adjusts the amount of water that is drawn through the reactor for denitrification. In addition, it controls the amount of organic carbon that is injected into the reactor, thereby manipulating the ORP through bacterial metabolism. This also prevents a carbon limitation for the heterotrophic denitrifying bacteria, as they require a carbon source to break down nitrate. In the same way as we humans breathe oxygen, the bacteria in a DyMiCo reactor respire nitrate to obtain energy from an organic carbon source. Through a series of biochemical reactions, nitrate is eventually converted into nitrogen gas (N2) that dissipates through the water column.
For those who have a more intimate knowledge of filtration and the role of bacteria in this process, DyMiCo may sound a lot like the Deep Sand Bed (DSB) and Jaubert method. In essence, this is true as all three systems employ denitrification to maintain high water quality. DyMiCo, however, goes a few steps further by measuring the biochemical processes that occur in the reactor, and using that information to maintain optimal ORP values for denitrification by modulating water throughput and carbon injection of the reactor. In addition, CO2 injection ensures that the reactor maintains a slightly acidic pH, allowing it to function as a calcium reactor as well as a denitrification unit. A system that uses DyMiCo does not require a foam fractionator or calcium reactor.
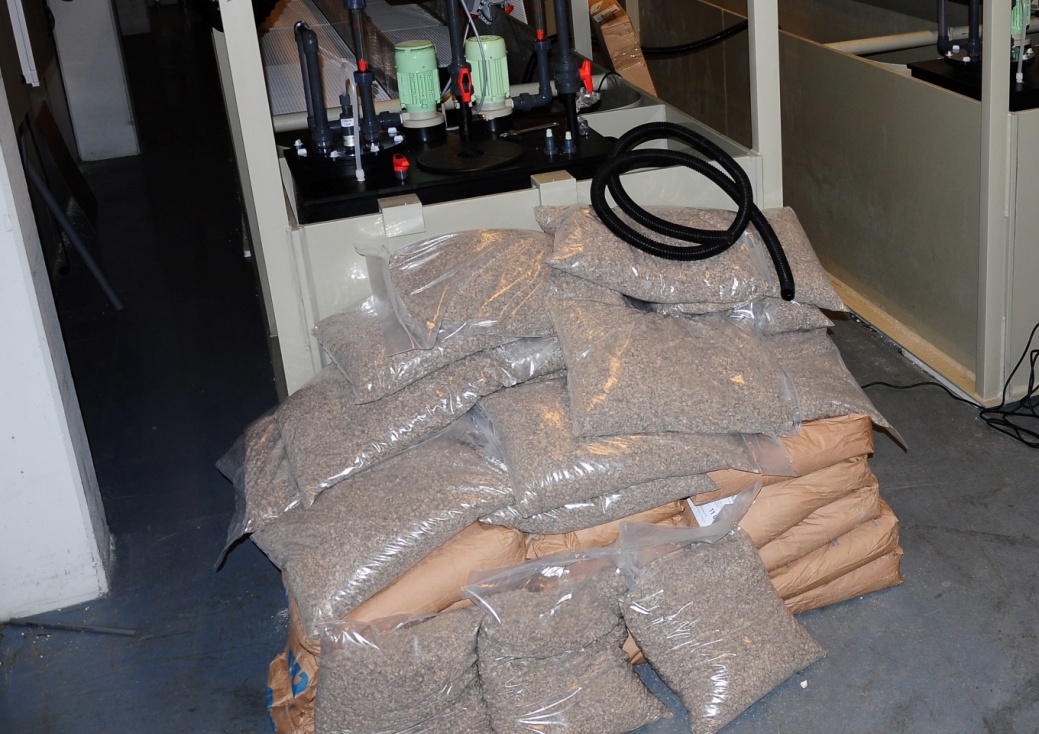
The main component of DyMiCo is the reactor or sand bed, here located underneath the black pump tank. The reactor is filled with a form of limestone and is adjusted to system volume and demand. In this case, the reactor size is 1,000 L (263 USG) or approximately 8% of total system volume. Photograph by Tim Wijgerde
A basic cycle of the DyMiCo control unit consists of several steps; first, nitrate-containing water is pulled into the reactor by a pull-through pump. Next, a pulse of organic carbon and CO2 is injected into the reactor, which is mixed throughout the reactor by a process pump. The last step is a lag phase that allows the bacteria in the reactor to break down the nitrate that came in with the fresh batch of water. This cycle is repeated continuously, and is dynamically adjusted based on the ORP and pH readings obtained from the interstitial water from the reactor. The schematic below provides a brief overview of the principles behind DyMiCo.
The end result of using DyMiCo in a closed system is clean water with a vibrant plankton population, including amphipods, isopods, copepods, small shrimp, and their nauplii. As a consequence, many invertebrates such as sponges, corals and echinoderms thrive. With this system, water changes are no longer required in most cases. To make things even better, the total power usage of the average DyMiCo system compares to the desktop computer you are reading this on. The standard model can be used to filter a system of up to 30,000 L or about 7,900 USG, at a total power consumption of only 300 Watts. Filter media for phosphate (Granular Ferric Oxide or GFO) or organic carbon (activated carbon) removal are not essential as the reactor is able to sequester various forms of inorganic phosphorus and organic carbon. Air stones may be used to prevent a decrease in pH and oxygen concentration of the water column, especially when stocking densities are high.
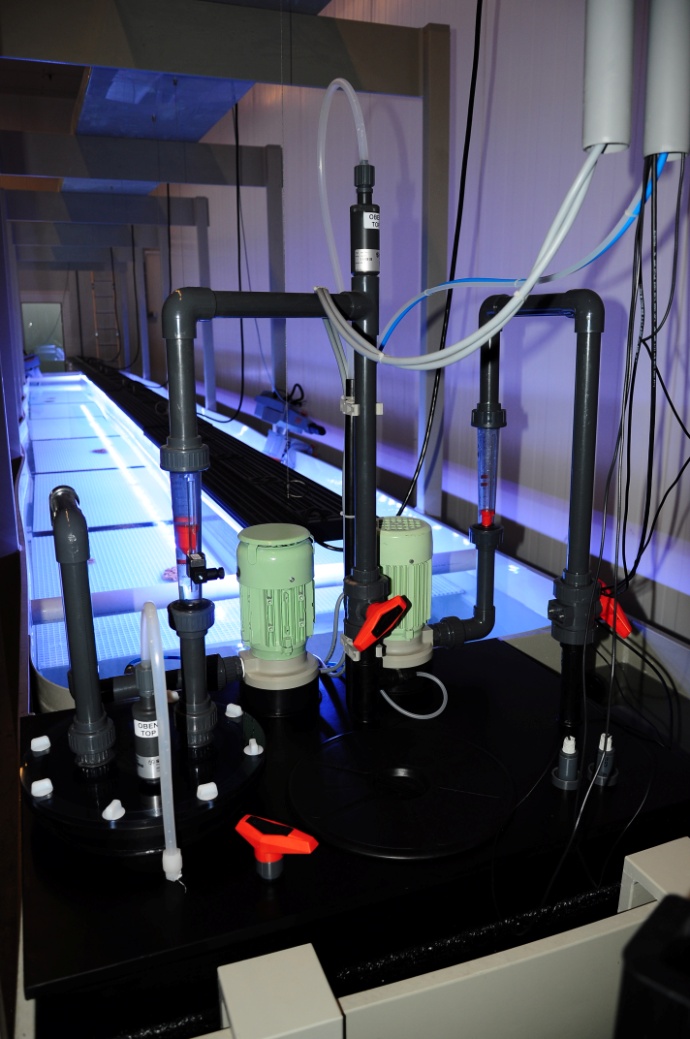
The second DyMiCo component: the pump tank which is suspended over the reactor. The left process pump powers an internal water circuit through the reactor, ensuring that a carbon source and carbon dioxide are delivered and mixed throughout the sand bed. The pull-through pump on the right returns clean water to the system, generating an under pressure which draws nitrate-rich water into the reactor. Two probes measure the ORP and pH values of the interstitial reactor water, and relay this information to the computer. Photograph by Tim Wijgerde
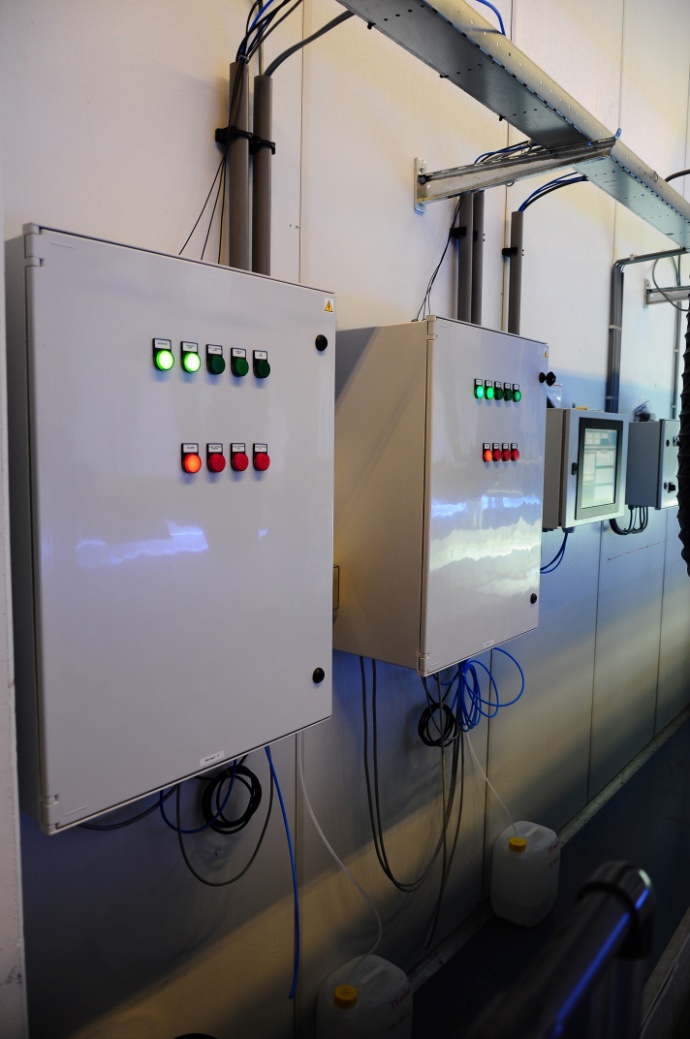
The final component that allows the system to operate is the control unit, a computer that receives information from the ORP and pH probes installed in the pump tank. The injection of a carbon source, carbon dioxide and water throughput are adjusted based on the ORP and pH values measured in the reactor water. Photograph by Tim Wijgerde
Typical water parameters
DyMiCo has proven to yield water parameters that are well suited for the aquaculture of marine organisms, including invertebrates. Inorganic macronutrients such as calcium (Ca2+) and magnesium (Mg2+) are invariably high as the reactor substrate, a form of limestone, slowly dissolves and releases these elements to the system water. Alkalinity, here defined as the ability of marine water to resist a change in pH, usually is very high due to the same reason; bicarbonate (HCO3–) and carbonate (CO32-) anions are released together with the above mentioned kations. Potassium (K+) and trace elements such as iodine (I–, IO3–), which are important for a variety of biological processes, can be maintained using high quality artificial salts, water changes and supplementation.
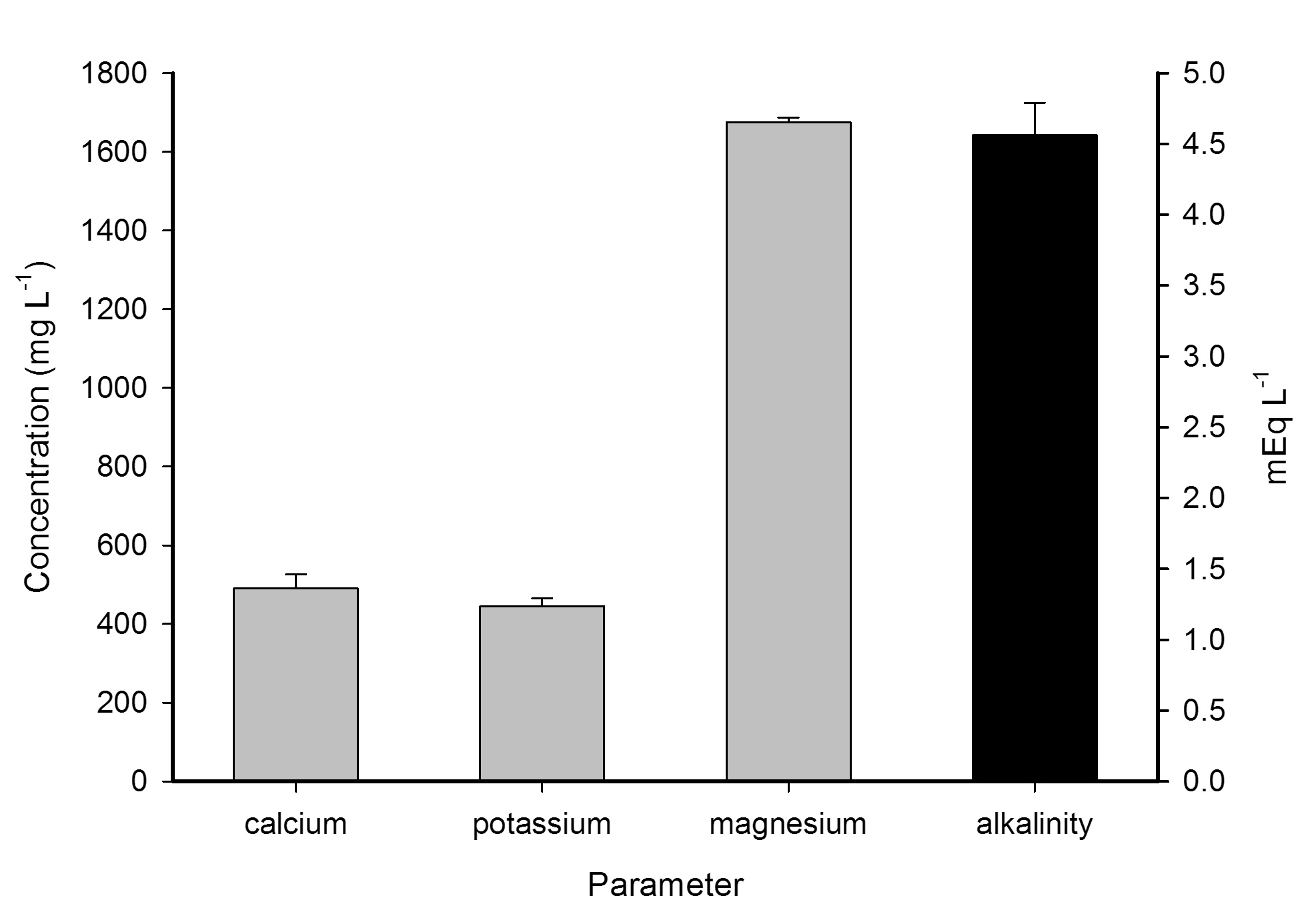
Calcium (Ca2+), potassium (K+), magnesium (Mg2+) in mg L-1 and alkalinity in mEq L-1 in a typical DyMiCo system. Error bars show standard deviations (N=2). Photograph by Tim Wijgerde

Ammonium (NH4+), nitrate (NO3-) and phosphate (PO43-) in mg L-1 in a typical DyMiCo system. Error bars show standard deviations (N=2). Photograph by Tim Wijgerde
Plankton-friendly pumps
A related issue which I should address is the usage of aquarium pumps, more specifically return pumps. High pressure and cavitation produced by these devices could damage a significant proportion of the resident plankton, thereby negating the plankton-saving properties of DyMiCo. Although plankton-friendly pumps are on the market today, these are not yet mainstream for (home) aquaria. These pumps are currently in use in various industries and are able to pump fluids without affecting resident particles.
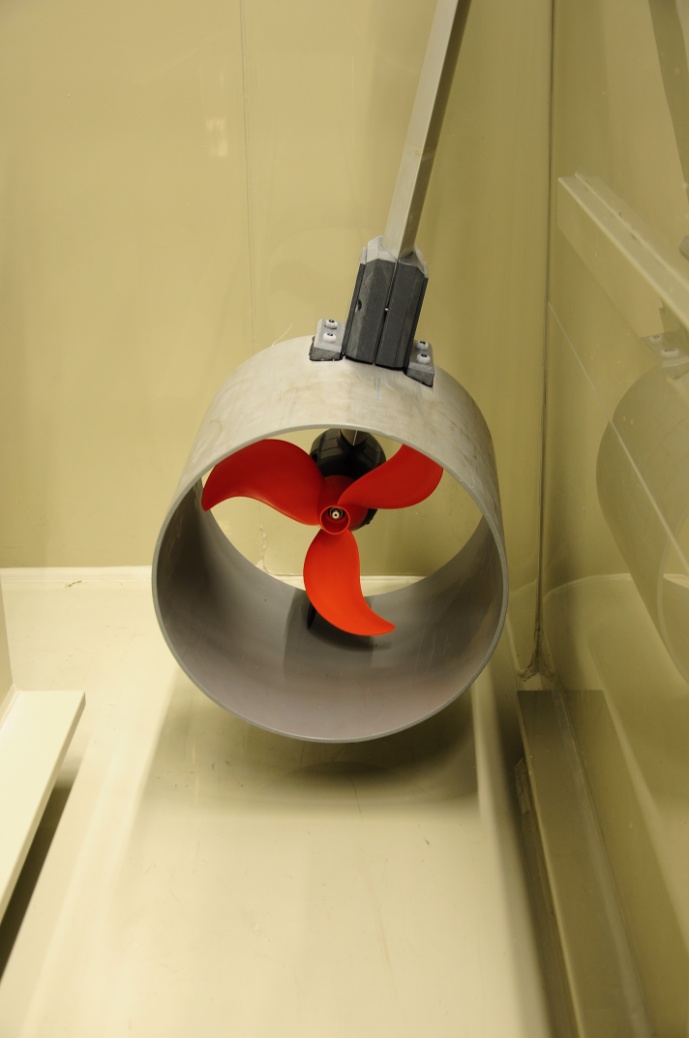
A modified outboard motor generates strong water motion at low energy cost. In addition, damage to plankton may be reduced. Photograph by Tim Wijgerde
A solution to this problem is making use of large propellers which can displace large water volumes at low rotational speeds. Low RPM pumps prevent damage to sensitive particles such as plankton, and are highly cost efficient. For example, a propeller with a diameter of 30 cm (one feet) can displace roughly 50,000 L or 13,000 USG per hour, at a power usage of only 30 Watts! Of course, this solution is not applicable to home aquaria, for which small high RPM pumps have been designed. In these small systems, pumps that do not produce pressure or cavitation may have significant value in terms of saving plankton.
Downscaling DyMiCo
At this moment, a downscaled version of the DyMiCo system is being developed for use in home aquaria. If such a small reactor yields the same results as its larger brothers, it could potentially revolutionize the marine aquarium hobby. Not only is this system a lot more economical in terms of energy and water usage, the husbandry of marine organisms would be greatly improved. Fish, shrimp and jellyfish breeders may also benefit from this system, as Kreisels are no longer required to prevent larvae from being killed. The protein skimmer as a filtration device, which has been the backbone of the marine aquarium hobby for decades, may lose ground over the next few years. We may, in the end, return to nature’s principles and rely on bacteria and the geochemical processes they drive.
I did not expound on all details surrounding DyMiCo, as my goal with this article was to provide the reader with a brief overview of this technology and what it can do for aquaculture and the aquarium hobby. In the second installment of this article, I will present some of the results that have been obtained with this technology over the last year. More specifically, I will address the aquaculture of live plankton and several commercially important marine invertebrates.
Tim Wijgerde, M.Sc. is a Ph.D. candidate at Aquaculture and Fisheries, Department of Animal Sciences, Wageningen University. His research focuses on the heterotrophy of scleractinian corals. To find out more about the Dynamic Mineral Control technology, visit the official website at www.ecodeco.eu.
References
- Feldman KS, Place AA, Joshi S, White G (2011) Bacterial counts in reef aquarium water: baseline values and modulation by carbon dosing, protein skimming, and granular activated carbon filtration. Advanced Aquarist, March 2010. <http://www.advancedaquarist.com/2011/3/aafeature>
- Heidelberg KB, O’Neil KL, Bythell JC, Sebens KP (2010) Vertical distribution and diel patterns of zooplankton abundance and biomass at Conch Reef, Florida Keys (USA). J Plankt Res 32:75-91
- Heidelberg KB, Sebens KP, Purcell JE (2004) Composition and sources of near reef zooplankton on a Jamaican forereef along with implications for coral feeding. Coral Reefs 23:263-276
- Hoegh-Guldberg O, Mumby PJ, Hooten AJ, Steneck RS, Greenfield P, Gomez E, Harvell CD, Sale PF, Edwards AJ, Caldeira K, Knowlton N, Eakin CM, Iglesias-Prieto R, Muthiga N, Bradbury RH, Dubi A, Hatziolos ME (2007) Coral reefs under rapid climate change and ocean acidification. Science 318:1737-1742
- Hughes TP, Baird AH, Bellwood DR, Card M, Connolly SR, Folke C, Grosberg R, Hoegh-Guldberg O, Jackson JBC, Kleypas J, Lough JM, Marshall P, Nyström M, Palumbi SR, Pandolfi JM, Rosen B, Roughgarden J (2003) Climate Change, Human Impacts, and the Resilience of Coral Reefs. Science 301:929-933
- Osinga R, Schutter M, Griffioen B, Wijffels RH, Verreth JAJ, Shafir S, Henard S, Taruffi M, Gili C, Lavorano S (2011) The biology and economics of coral growth. Mar Biotechnol. 13:658-671
- Palardy JE, Grottoli AG, Matthews KA (2006) Effect of naturally changing zooplankton concentrations on feeding rates of two coral species in the Eastern Pacific. J Exp Mar Biol Ecol 331:99-107
- Wabnitz C, Taylor M, Green E, Razak T (2003) From Ocean to Aquarium. UNEP-WCMC, Cambridge, UK
- Yahel R, Yahel G, Berman T, Jaffe JS, Genin A (2005) Diel pattern with abrupt crepuscular changes of zooplankton over a coral reef. Limnol Oceanogr 50:930-944
0 Comments