Although the subject of water changes in aquaria has been one of considerable debate over the years, they are generally considered to be beneficial. Refractory compounds’ (those substances resistant to traditional treatment methods) concentrations can be reduced, while desirable elements are at least supplemented. In short, we want to remove the ‘bad stuff’ and replace it with the ‘good’.
I suspect most successful reef hobbyists adhere to a general maintenance schedule and exercise reasonable care in their husbandry procedures. But what does that mean? For example, when making water changes, how many hobbyists adjust their ‘new’ water to match the existing conditions within the aquarium (such as pH and other parameters)? I suspect those doing so are in the minority, and think most will judge the suitability of exchange water based on two traits – salinity and temperature. In the cases presented below, I measured and adjusted only the salinity of the ‘new’ water before making a water exchange. How did this affect the parameters in the aquarium? And, more importantly, did these changes become detrimental to the aquarium’s inhabitants (particularly corals?).
Bingman (1995) reported coral bleaching as a result of increased water clarity (that is, increased transmission of visible and UV radiation) from either introduction of granular activated carbon or large water changes. In addition, ozone would surely aid in the removal of at least some compounds known to cause yellow water thus increasing transmission of visible/UV light. This article will revisit those thoughts, and, in addition, will quantity increased dosages of radiation bandwidths – Total UV-A + B, and PAR.
It is not the intent of this article to examine the full effects of water exchanges on water chemistry; instead our attention will be devoted to observations of the physical side effects. These will include the water column’s transmission of visible light (PAR, or Photosynthetically Active Radiation) and ultraviolet radiation (UVR), water color, pH, oxidation-reduction potential (ORP), salinity, and dissolved oxygen. Here, we report the effects observed before and after two monthly water exchanges and discussion the results’ implications. Our examination begins with:
Water Color
Water color can be a result of many factors, though in aquaria it is generally considered to be result of decomposing organic matter or exudates from algae. These extracellular products can include amino acids, carbohydrates, alcohols, vitamins, enzymes, volatile organic compounds, and ultraviolet radiation-absorbing substances (Fogg, 1966). The term ‘yellowing’ is often used to describe the apparent effect of increased water color. Besides its impact on aesthetic appeal, we know that yellow water can have an impact on light transmission through a water column and can affect PAR (particularly violet and blue wavelengths) and UVR.
The first step in a series of experiments examined water color of an aquarium containing a few fish and ‘filtered’ by protein skimming, mechanical filtration and light use of activated carbon. A water exchange of 40-50% is conducted on a schedule of more or less every 4 weeks. A Hach DR 890 colorimeter determined water color in platinum-cobalt units. De-ionized water was used to zero the instrument, and, since the water did not appear to contain suspended matter, no attempt was made to remove suspended solids. Figure One shows the reduction in water color after a monthly 50% water change.
Technically, the pH of the water should be reported along with the color determinations since water color increases as the pH rises (Standard Methods, 20th Edition, 1998). pH is reported below and discussed in the closing statements.
Even with diligent maintenance and husbandry, we see that the yellowing of water occurs in a relatively short period of time, and suggests that another battery of tests can proceed: That of the water column’s ability to transmit visible light and ultraviolet radiation.
Photosynthetically Active Radiation (PAR) Transmission
PAR is generally considered to be composed of light wavelengths between 400 nm and 700 nm. This bandwidth includes most wavelengths known to promote photosynthesis.
Sudden changes in PAR (especially when combined with increases in water temperature and ultraviolet radiation) can potentially induce the coral animal host to eject some or all of its symbiotic dinoflagellate population in a process known as bleaching. Corals suffering from the effects of bleaching are at a competitive disadvantage and, in extreme cases, might die.
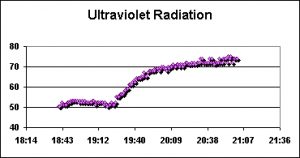
Figure 3. Note the increased transmission of UVR at the onset of the water change beginning at ~19:30 hours.
In order to test for the impacts of water exchanges on PAR transmission, a WatchDog data logger equipped with a PAR sensor was programmed to measure light intensity every 60 seconds, and the sensor was placed approximately 19 inches (16 inches of this the water column) below a reflector housing a 250-watt metal halide lamp. Baseline data was obtained before the water change was performed. Figure Two presents results typical of those obtained before and after both water changes.
Total Ultraviolet Radiation Transmission
As with PAR, two sets of tests were conducted before, during, and after monthly water changes. A WatchDog data logger equipped with a UV sensor recorded intensity every 60 seconds. The results are shown in Figures 3 and 4.
UV-A Spectral Characteristics and Intensity During a Water Change
Ultraviolet-A radiation is that bandwidth just below the visible wavelengths and is generally described as containing wavelengths between 320nm and 400 nm. Though it is considered less damaging than UV-B and UV-C bandwidths, its presence should not be taken lightly (no pun intended). Note that there is no known UV-absorbing compound (such as mycosporine-like amino acids) that absorbs UV at the maximum wavelength generated by this and many other lamps (365 nm). In addition, many if not most plants and algae can utilize at least some portions of UV-A for promoting photosynthesis. Characterization of UV-A transmitted through the water column was made in order to determine if colored water preferentially absorbed certain UV wavelengths. This test utilized an Ocean Optics USB2000 configured for collection of UV and visible light, and equipped with a 600-micron patch cord. See Figure 5.
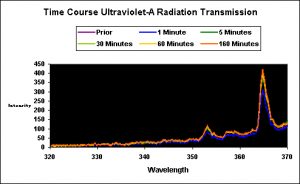
Figure 5. Spectral characteristics and intensity of UV transmitted through a water column before during and after a water change.
Temperature
Temperature needs little introduction. Its ease of measurement makes it perhaps the most often measured parameter within an aquarium.
Due to various constraints, water used for changes is mixed outdoors in a 55-gallon plastic drum. This drum is exposed to not only ambient out door temperatures at sea level in Hawaii, but also gains some heat from the submersible pump used for mixing. Thus, this water can be quite a bit warmer than that of the aquarium water.
Figures 6 and 7 demonstrate temperature modulations during two water changes.
Water temperature was gathered using the built-in temperature sensors of pH, salinity, dissolved oxygen and redox probes connected to two Hach HQ40d multi-meter data loggers.
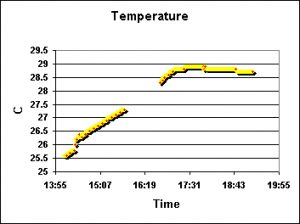
Figure 7. Heating of the water during the initial portion of this test is attributed to heat transfer from a metal halide lamp, which did nothing to cool the influx of warm exchange water. The interruption of data was due to intentional shutting down of the recording instrument in order to conserve limited memory capacity.
Oxidation Reduction Potential
Oxidation Reduction Potential, or ORP (also known as reduction-oxidation potential or redox) is the general tendency of substances within aquarium water to acquire electrons thus becoming reduced. ORP is often reported on a scale of -1999 to +1999 millivolts (mV). In aquarium water the reduction potential is the tendency of the solution to either gain or lose electrons when it is subject to change by introduction of a chemical (such as ozone). It does not characterize the capacity of the system for oxidation or reduction, in much the same way that pH does not characterize alkalinity or acidity. With that said, the measurements I’ve made of ocean water here in Hawaii have generally been within the range of 200 – 250 mV.
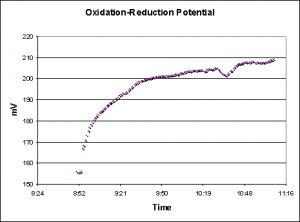
Figure 9. ORP measurements were made and recorded with a Hach redox sensor connected to an HQ40d multi-meter.
pH
pH is a logarithmic scale of… blah, blah, blah. We all know (or should) what pH is and how to interpret the measurements.
pH measurements were made and recorded with a Hach pH sensor connected to an HQ40d multi-meter.
Dissolved Oxygen
The amount of dissolved oxygen (DO) in water is dependent upon several factors, including water temperature, altitude and barometric pressure. The amount of DO will decrease with increasing temperature and altitude. However, the most oxygen seawater can hold at sea level is generally considered to be about 7 mg/l (milligrams per liter, or parts per million), and any measurement higher than this is said to be super-saturated. In many biological processes, a rule of thumb suggests maintaining a DO of at least 2 mg/l.
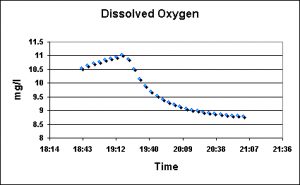
Figure 12. Dissolved Oxygen concentrations fell as freshly-mixed seawater entered the system. This is not surprising given the circumstances.
DO measurements were made and recorded with one of Hach’s new luminescent dissolved oxygen sensors connected to an HQ40d multi-meter and data logger.
Discussion
Table One summarizes the results observed during two water changes. All parameters, save pH, demonstrated similar (either up or down) swings.
Item | Increased | Decreased |
---|---|---|
Color | x | |
PAR Transmission | x | |
UV Transmission | x | |
Temperature | x | |
pH | x | x |
Dissolved Oxygen | x | |
Redox Potential | x |
Should we be concerned with these modulations?
pH of the water rose during the first water change, and fell the next. The exact reason(s) for dissimilar results is unknown, but the degree of shift in pH in both cases is likely of little concern. However, see comments below on the effects of pH on water color (and hence light transmission through the water column).
The same can be said for the rise in the oxidation-reduction potential. Careful observation of corals’ responses to sharp decrease or increase in ORP usually includes tentacle retraction – this is usually a short-lived reaction.
The dissolved oxygen content of the water fell during both exchanges, and for good reason. This particular setup involves a closed-system aquarium and a 100-gallon sump. The pump transferring water from the sump is shut down, and most of the water is drained from the sump. Once this is completed, the ‘new’ water is pumped into the sump and, once full, the recirculation pump is started. During the time the sump is empty and no recirculation occurs between the sump and the aquarium, the photosynthetic alga and corals produce oxygen which accumulates to supersaturated concentrations. I’m speculating here, but if the water contains this much oxygen, then the corals’ tissues probably contain much more oxygen and I have to wonder if dissolved oxygen concentrations could be used as a proxy for harmful superoxide radicals (these dangerous forms of oxygen are injurious to zooxanthellae and coral tissues). Although the water change is not directly responsible for the elevated DO concentrations, this observation suggests that oxygen concentrations in smaller aquaria, even when not strongly illuminated, could rise to high levels. This deserves further investigation.
The degree of water color, in and of itself, is not particularly important other than for aesthetic reasons. However, the results of these experiments demonstrate that increased transmission of visible and UV radiation can occur due to the decrease of compounds capable of absorbing light energy. Water color invariably increases with rising pH, and vice versa (Standard Methods, 1998). This is a potentially important clue.
Note that water color was determined via spectrometry and did not rely on any of the commercially available comparative strips specifically marketed for determination of ‘yellow water”. It has been my experience that water yellowing should never advance to the degree indicated by these strips!
This leaves us with the three parameters known, when drastic changes occur, to induce coral bleaching. These are: Light energy responsible for the promotion of photosynthesis (Photosynthetically Active Radiation, or PAR), ultraviolet radiation (or UVR) and water temperature. Note that all three increased in both phases of testing. While by themselves these may not be dangerous, it is possible that these 3 parameters acting in synergy could harm captive corals (in fact, a very mild bleaching episode was observed after the latter water change. The corals lost some coloration and appeared ‘washed out’).
Based on this experience, I will have to become more attentive to controlling the temperature of the ‘new’ water, and will increase its pH in an attempt to minimize the impacts of increased transmission of visible and UV radiation due to a lower pH. Admittedly, the impact of color and pH is most likely much less than increased light transmission due to simple dilution of yellowing compounds by the water exchange, but until I get a better handle of how much of an impact, I’ll play it safe.
I’m conducting some experiments on how we can eliminate the potentially negative impacts of water exchanges, and hope to have results soon. In the meantime, please direct any questions to the comments section below.
References
- American Public Health Association, 1998. Standard Methods for the Examination of Water and Wastewater, 20th Edition. Maryland Composition Company, Glen Burnie, MD.
- Bingman, C., 1995. The effect of activated carbon treatment on the transmission of visible and UV light through aquarium water. Part 1: Time-course of activated carbon treatment and biological effects. Aquarium Frontiers, Summer issue.
- Fogg, G.E., 1966. The extracellular products of algae. Oceanogr. Mar. Biol. Ann. Rev., 4: 195-212.
0 Comments