This editorial begins to explore the fundamentals of marine system dynamics which will benefit all aquarists.
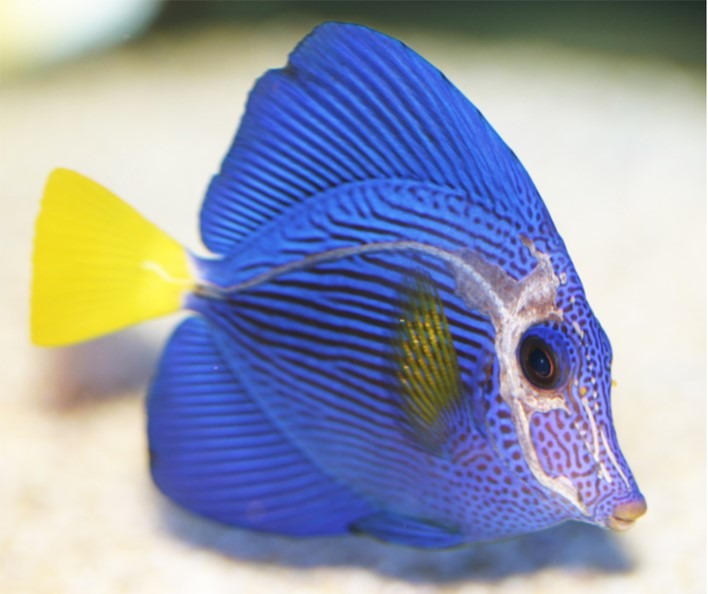
Fig 1. A purple tang (Zebrasoma xanthurum) exemplifying advanced head and lateral line erosion (HLLE).
The optimum solubility ratio of oxygen to carbon dioxide in seawater is 1 to 282 (Muangkaew et al. 2002; Dickson 2010) so merely 18 litres of dissolved oxygen (DO) can assimilate into the entire volume of an Olympic-sized swimming pool filled with saltwater, while the same pool can carry 5,000 litres of CO2. Furthermore, saltwater is predisposed to retaining less useful gases like CO2 and dinitrogen (N2) from respiration and denitrification which occupies space that might otherwise bear oxygen. Essentially, each marine system must optimise surplus gas discharge (degas) and useful gas replenishment (regas).
Several factors influence degas/regas. The degas competency of protein skimmers is likely negligeable insofar as they compress gas into solution and the solubility of DO in seawater is very low. Ornamental marine animals and bacteria that perform the nitrifying arm of the nitrogen cycle absorb DO and expire CO2 like algae in darkness, so nighttime is when the DO requirement of the system increases. Wavemaker nocturnal cycles downregulate flow because they were designed by engineers like so many peripherals and filtration components sold for marine systems, while here we discover safe compensatory stratagems. Disc-shaped fish like tangs, surgeonfish, butterflyfish, and angelfish require nightly torpors so we provide them with less turbulent grottos where they can rest, because lessening flow at night is potentially catastrophic.
Shallow trough aquaria present an expanse of surface area to the detriment of volume and visual impact, while a satisfactory volume to surface area ratio is retained by square-ended aquaria or cubes (Fig 3.). Tall thin aquaria pose insurmountable challenges to reef aquarists and must be avoided so order another and start again. As the volume of a display increases, its surface area to volume ratio decreases, so smaller aquaria have proportionally more meniscus for gas exchange, but they offer less stability to which these organisms are suited.
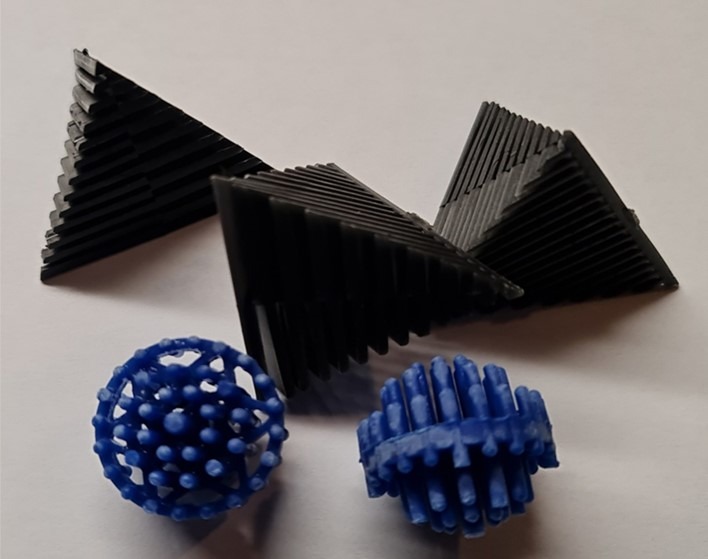
Fig 2. Bioball and biopyramid media provide maximum surface area for degas/regas and microbial inhabitation.
Degas occurs when water is in contact with air which is directly proportional to flow because discrete water molecules reach the surface more frequently. “In-tank” systems devoid of sumps irrespective of water movement accumulate gas exchange-inhibiting proteinaceous surface scum. Such slicks are resuspended and made protein skimmer exportable by streams of centimetre-sized air bubbles emanating from open-ended 5-millimetre outer diameter (OD) acrylic pipe supplied by a 300 lph air pump. Smaller and larger air bubbles are actively discouraged so adjust pump output accordingly. Such aeration augments degas slick-or-no-slick.
Reef aquaria must exchange large volumes of gas with air on an hourly basis and covering a reef causes degas-diminishing heavier than oxygen CO2 to form an asphyxiating blanket, where nocturnal hypoxia can instigate nightly fish demise until dwindling biomass expedites a precarious equilibrium.
Trickle towers are used in commercial systems to degas and regas from which domestic reefs benefit greatly. Square, tall glass sump system weirs were previously filled with bioballs and up drafted with air (Fig 3.; Fig 4.). Trickle weirs likely became obsolete due to their competency at generating waterborne nitrate distal to substrate and rock confined coupled nitrogen cycling. Such “nitrate machines” may cause nitrate to rise; however, pouring fresh reverse osmosis (R/O) water onto bioballs weekly and waiting for 10 minutes before resuming flow, bursts marine bacteria via osmotic shock. External or internal cannister filters are unnecessary because corals respond well to suspended solids and they generate nitrate, yet replacing their medium every fortnight forestalls their maturation.
The latest less intrusive weir syphons move water quickly and quietly, albeit water does not move through them uniformly and as such, some may commence to diminish in DO. Waterborne bacteria in mature systems cause stagnation if the DO of saltwater falls below a certain threshold which evolves deadly toxins. All water in a marine system must be homogenous and move uniformly to ensure systemwide oxygenation. That includes water under pressure in valved sidearms, at the extremities of spray bars, trapped beneath sump peripherals including the baseplates of return pumps and reactors, within hollow resin décor, or behind the beautification panels of pumps and powerheads. At least crack all valves, exploit a mini powerhead in existing syphon weirs to make sure that their waters thoroughly mix, and gently prise the rears off return pumps and other equipment.
Corals are stressed by fluctuating and stable temperatures above 27oC, while cooler water carries more DO which explains the productivity at the Poles. Acropora cervicornis grow more rapidly in winter because they expend fewer resources on homeostasis (Ruiz-Diaz et al. 2022, cited in Toledo-Hernandez et al. 2023) while the author has kept his reefs at 23.5oC year-round for over 20 years. Deltec® manufactured evaporative chillers early in the millennium yet such items are now obsolete. However, they can be DIY fabricated with nominal skills while they optimise degas and use 1 percent of the energy of refrigerant chillers (Fig 7.). Minimise or eradicate standing water in pipework including dosing and auto top-up pumps because “potions” and R/O water are not sterile (Feldman 2011), and stagnation is extremely harmful (Elliott et al. 1987; Roberts 1989; Jetten et al. 1998; Poulton et al. 2002; Jones 2006; Signe et al. 2009; Nielsen et al. 2010; Dornhoffer 2014; Siang et al. 2017; Aslett 2024).
Aerate all R/O water from the moment of production with large air bubbles, operate R/O filters 24/7 to waste if necessary, and aerate “salt mix” for a minimum of 48 hours before use to ensure adequate CO2-derived buffering.
Substrate, Rock, and Bricks: Safe Denitrification
Once again, we must safeguard against the conditions which cause stagnation because water remains stationary within rockwork and sand/gravel and as such becomes microaerobic (suboxic) and anaerobic (anoxic). As reef aquarists we value such zones which are indispensable for SPS reefs because these colonies turn brown and their growth diminishes from 1 milligrams per litre (mg l-1; ppm) of nitrate which is barely detectable (Balling et al. 2008). Nevertheless, pastel hues emerge on SPS colonies exposed to nutrient-limited nitrate and phosphate because these corals require these ionic compounds but only within a critical range. Enriched they become pollutants, yet when scarce the coral and its algal partners die.
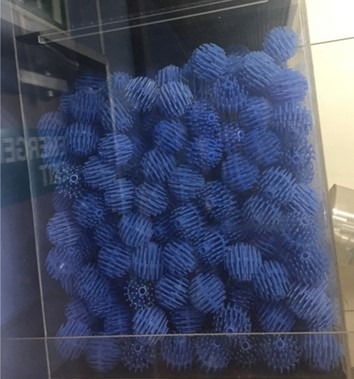
Fig 4. A 1990s domestic trickle tower which is part of a purpose-built reef sump. Photograph from Pet and Aquatic World, Armley, Leeds. Aslett ©. Out of fashion does not mean ineffective.
Microaerobic/anaerobic zones only become hazardous when water’s DO is diminished because they could surface and release toxic by-products into water (Neilsen et al. 2010). Taking heed of the above information how could that possibly occur.
From the smallest bacterium to the largest fish, marine systems take on a life of their own and each inhabitant produces waste. Those essential and welcome nitrogen-cycling microorganisms produce cyclic aromatic hydrocarbons called phenolics. Phenols are also liberated by corals as aggression compounds including terpenoids and carotenoids (Knee 2001; Wilson, personal communication; Riddle 2014; Lee et al. 2017). These compounds are only mildly reductive in negligeable concentrations, but if not regularly purged, they accumulate and attack nitrifiers and the central nervous systems (CNSs) of fish. Reductants literally lessen DO and when diminished, there is less to confine anaerobia to the inside of rock and beneath substrate (Nielsen et al. 2010).
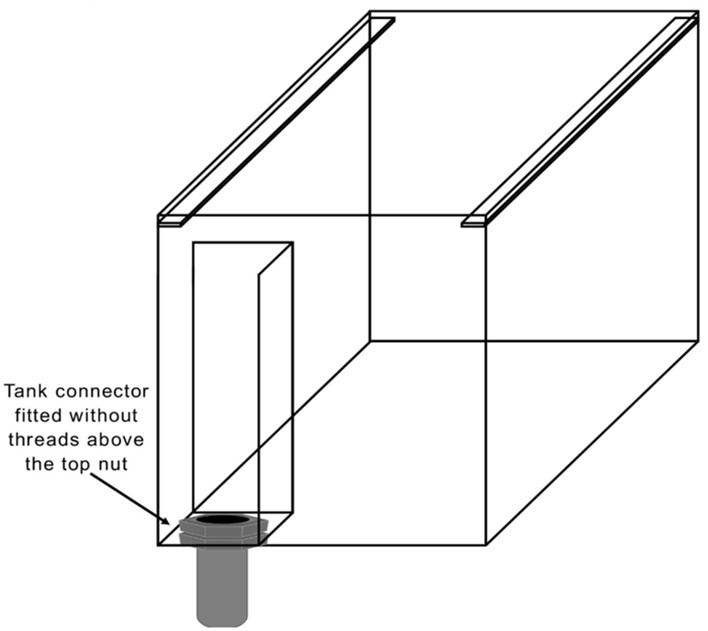
Fig 6. An illustration of an aquarium fitted with a two-sided glass weir.
Phenols also turn water yellow which scatters and absorbs useful blue light so they must be routinely eliminated because leave phenols to amass for a month and a half, and corals are photoshocked at the sudden presence of intense blue light. Blue LEDs generate potentially harmful bleaching intensities (Riddle, personal communication, cited in Neptune Systems 2019) yet metal halide lamps and fixtures are endorsed for SPS colonies. Ozone is used to oxidise phenolics in comparatively smelly and polluted fish-only systems but applying ozone to bight, clear, reef water generates injurious secondary oxidants (Westerhoff et al. 1998; Jones et al. 2006; Perrins et al. 2006). The only tool at our disposal is thus granular activated carbon (GAC) which causes head and lateral line erosion (HLLE) in susceptible fish which just happen to be the prized reef janitors: tangs and surgeonfish (Fig 1.). The lateral line canal runs from the bass of the tail (the caudal peduncle), along the vertebral flank, onto the opercular, around the eyes, and onto the snout. It is integral to the CNS and is highly sensitive which provides pressure and water velocity feedback that assists shoaling and predation avoidance (Dow et al. 2018). It is assumed that spicules of GAC enter the canals and instigate erosion which must be painful (Graber 2010; Ho 2011; Stamper et al. 2011). Nevertheless, GAC’s impacts are minimised when used correctly.
Activated carbon must be the right grade because some described as suitable for aquaria are washed in phosphoric acid which releases reef-devastating quantities of phosphate (Delbeek & Sprung 1994). The author tests all his consumables with a Salifert® phosphate kit and GACs are no exception. No matter how they are described, some of this industry’s manufacturers do unfathomable things like supply phosphate-free GACs and phosphate absorbing granular ferric oxide (GFO) but with filter bags that contain and release significant phosphate. Furthermore, such pollution is often released slowly which mystifies some of the most experienced “reefers”. Test everything.
Include a small quantity of any consumable in an R/O water phosphate test and shake. Blue indicates the item is unsuitable or in the case of feeds which always include phosphate like live phytoplankton, it advises caution (Fig 8.). Prerinse GAC in copious amounts of R/O water before use and force water through it or fluidise for merely 48 hours, because it begins to release what it has absorbed after two days which renders its continuous use, as ever moot.
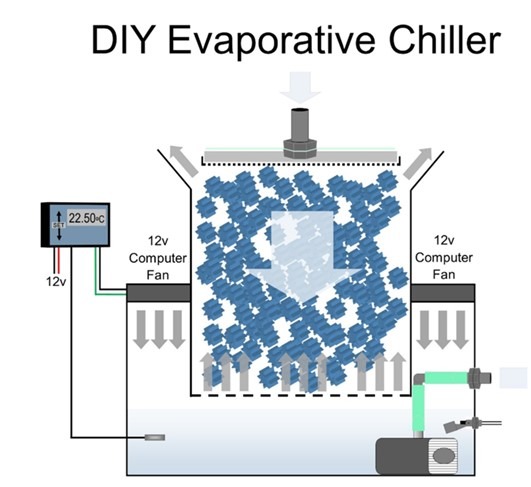
Fig. 7
So microporous substrates like corals sands, live rock, and sump bricks provide acres of surface area for inhabitation with microbes that couple both arms of the nitrogen cycle to nitrify ammonia to nitrate and denitrify nitrate to dissolved dinitrogen. N2 surface degas thus nullifies ammonia excretion.
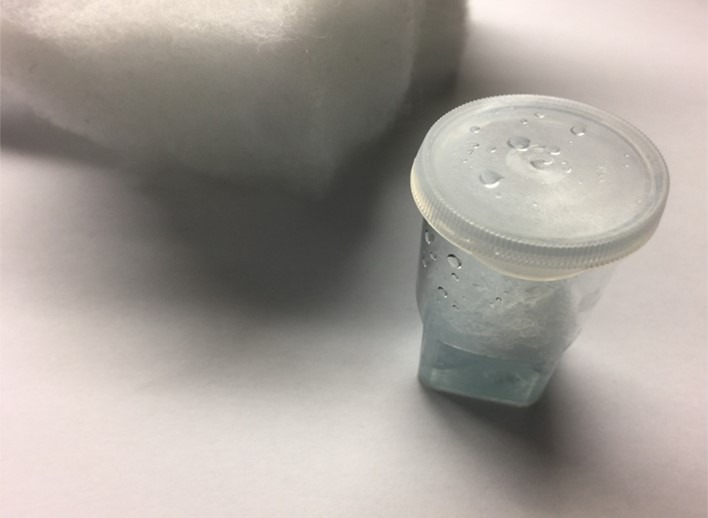
Fig 8. Top left – a section of pond filter wool/matting. Bottom right – a phosphate test containing the reagents, R/O water, and matting fibers. Blue indicates a positive result therefore test filter wool for the presence of phosphate. Test everything.
Despite providing masses of this type of substratum and filtration, nitrate can still rise. A number of practices have likely stifled denitrification in the modern reef aquarium which is beyond the scope of this article, yet is discussed in the next.
References
Aslett, C., G. (2024) The Complete Reef Aquarist, for the hobby, trade and academics – A Conservation Manual. Aslett, C., G. (ed.). Reef Ranch Publishing Ltd, Leeds, West Yorkshire, UK. In press. https://www.reefranch.co.uk/
Balling, H., W., Janse, M. & Sondervan, P. (2008) Trace elements, functions, sinks and replenishment in reef aquaria. Advances in coral husbandry in public aquariums. Leewis, R., J. & Janse, M. (eds.). Burgers’ Zoo, Arnhem, The Netherlands. pp 143-156.
Delbeek, C. & Sprung, J. (1994) The Reef Aquarium: A Comprehensive Guide to the Identification and Care of Tropical Marine Invertebrates (Volume 1). Two Little Fishies. p 115.
Dickson, A., G. (2010) The carbon dioxide system in seawater: equilibrium chemistry and measurements. Scripps Institution of Oceanography, University of California, USA. https://www.researchgate.net/publication/284774361_The_carbon_dioxide_system_in_seawater_Equilibrium_chemistry_and_measurements
Dornhoffer, T. (2014) Nitrogen Cycling Revisited: Sand, critters, carbon, and why you may be under-feeding your tank. AdvancedAquarist.com. https://www.advancedaquarist.com/2014/5/chemistry
Dow, E., Jacobo, A., Hossain, S., Siletti, K. & Hudspeth, A., J. (2018) Connectomics of the zebrafish’s lateral-line neuromast reveals wiring and miswiring in a simple microcircuit. eLife. 7, e33988.
Elliott, S., Lu, E. & Rowland, F. (1987) Carbonyl sulfide hydrolysis as a source of hydrogen sulfide in open ocean seawater. Geophysical Research Letters. 14(2), 131-134.
Graber, S. (2011) Activated carbon indicted in inducing head and lateral line erosion. https://www.advancedaquarist.com/blog/activated-carbon-indicted-in-inducing-head-and-lateral-line-erosion
Ho, L. (2011) Activated carbon affirmed as causative agent for HLLE disease. AdvancedAquarist.com. https://www.advancedaquarist.com/blog/activated-carbon-affirmed-as-causative-agent-for-hlle-disease
Jetten, M., Strous, M., van de Pas-Schoonen, K., Schalk, J., van Dongen, U., van de Graaf, A., Logemann, S., Muyzer, G., van Loosdrecht, M. & Kuenen, J. (1998) The anaerobic oxidation of ammonium. FEMS Microbiology Reviews. 22(5), 421-437.
Jones, A., Gensemer, R., Stubblefield, W., van Genderen, E., Dethloff, G. & Cooper, W. (2006) Toxicity of ozonated seawater to marine organisms. Environmental Toxicology & Chemistry. 25(10), 2683-2691.
Jones, A., Gensemer, R., Stubblefield, W., van Genderen, E., Dethloff, G. & Cooper, W. (2006) Toxicity of ozonated seawater to marine organisms. Environmental Toxicology & Chemistry. 25(10), 2683-2691.
Knee, M. (2001) Terpenoids and Phenolics. Oregon State University. PlantFacts.osu. https://plantfacts.osu.edu/resources/hcs300/biochem3.htm
Lee, Y., S., Duh, T., H., Siao, S., S., Chang, R., C., Wang, S., K. & Duh, C., Y. (2017) New Cytotoxic Terpenoids from Soft Corals Nephthea chabroli and Paralemnalia thyrsoides. Marine drugs. 15(12), 392
Muangkaew, S., McKelvie, I., Grace, M., Rayanakorn, M., Grudpan, K., Jakmunee, J. & Nacapricha, D. (2002) A reverse-flow injection analysis method for the determination of dissolved oxygen in fresh and marine waters. Talanta. 58(6), 1285-1291.
Neptune Systems (2019) #LetsTalkReef – Today its all about measuring light – with Guest Dana Riddle. https://www.youtube.com/watch?v=LKlFA59-Acc
Nielsen, L., Risgaard-Petersen, N., Fossing, H., Christensen, P. & Sayama, M. (2010) Electric currents couple spatially separated biogeochemical processes in marine sediment. Nature. 463(7284), 1071-1074.
Perrins, J., Cooper, W., van Leeuwen, J. & Herwig, R. (2006) Ozonation of seawater from different locations: Formation and decay of total residual oxidant—implications for ballast water treatment. Marine Pollution Bulletin. 52(9), 1023-1033.
Poulton, S., Krom, M., Rijn, J. & Raiswell, R. (2002) The use of hydrous iron (III) oxides for the removal of hydrogen sulphide in aqueous systems. Water Research. 36(4), 825-834.
Riddle, D. (2014) Effects of Yellow Water on Light Transmission in an Aquarium – Why Should You Care. AdvancedAquarist.com. https://www.advancedaquarist.com/2014/1/aafeature
Roberts, R., J. (1989) Fish Pathology 2nd Edition. Bailliere Tidal, 24-28 Oval Road, London. p 70.
Ruiz-Diaz, C., P., Toledo-Hernández, C. & Sánchez, J., L. (2022) The effects of depth-related environmental factors and source of collection on life history traits in Acropora cervicornis raised in nurseries. Water J. 14, 212. https://doi.org/10.3390/w14020212
Siang, H., Y., Tahir, N., M., Malek, A. & Isa, M., A., M. (2017) Breakdown of hydrogen sulfide in seawater under different ratio of dissolved oxygen/hydrogen sulfide. Malaysian Journal of Analytical Sciences. 21, 1016-1027.
Signe, H., Revsbech, N., P., Kuenen, J., G., Jorgensen, B., B., Gallardo, V., A., van de Vossenberg, J., Neilsen, J., L., Holmkvist, L., Arning, E., T. & Neilsen, L., P. (2009) Physiology and behaviour of marine Thioploca. Microbial population and community ecology. 3, 647-657.
Stamper, M., A., Michele, M., Kittell, M., M., Patel, E., E. & Corwin, A., L. (2011) Effects of Full-Stream Carbon Filtration on the Development of Head and Lateral Line Erosion Syndrome (HLLES) in Ocean Surgeon. Journal of Aquatic Animal Health. 23(3), 111-116.
Toledo-Hernandez, C., Ruiz-Diaz, C., P., Ramirez-Lugo, J., S., Torres-Diaz, M., Santiago-Pagan, L., Bruno-Chardon, A. & Diaz-Vazquez, L., M. (2023) Uncovering the link between environmental factors and coral immunity: A study of fluorescent protein expression and phenoloxidase activity in Acropora cervicornis. Forntiers in Marine Scinence. https://dio.org/10.3389/fmars.2023.1133486
Westerhoff, P., Song, R., Amy, G. & Minear, R. (1998) Numerical kinetic models for bromide oxidation to bromine and bromate. Water Research. 32(5), 1687-1699.
Wilson, M. (2008) World Feeds Limited, 3b Coulman Street Industrial Estate, Thorne, Doncaster, DN8 5JS, United Kingdom.
0 Comments