“All substances are poisons; there is none which is not a poison. The right [concentration] differentiates a poison from a remedy.”
-Paracelsus
Toxicology, or the study of poisons and their modes of action, can be a very relevant addendum to any aquarist’s background. Toxicity can be elicited from sources within a tank or from sources outside of the tank. This discussion leads us through many of the more salient points regarding reef aquaria and the relationships of toxicology.
We are all familiar with the presence of toxic organisms in our tanks or at least the possibility of them. Cucumbers can produce the ever fearful ‘cuke nuke’ or we might reach in and find ourselves nursing a sting from a foxface rabbitfish. But, toxicity in our tanks can have a diverse array of sources. These can range from the use of aerosols or solvents (such as ammonia, hairspray, or spray lubricants) in close proximity to our tanks to the presence of certain corals, fish, or alga within the system to that frying pan in the kitchen that occasionally smokes up the house.
In discussing toxicity in our tanks there are a few terms with which we should all be familiar:
- Toxins are compounds that are natural in origin, such as those from invertebrates, fish, or alga. These can be proteins, small peptides, or other organic compounds.
- Toxicants are those compounds that are anthropogenic in nature (human made) such as pesticides, solvents, or residues from burning fossil fuels or waste.
- Bioaccumulation is the process of a chemical or toxin passing from the environment into living tissue, such as a solvent used in paint gradually making its way into the fish in your tank. Bioaccumulation consists of uptake from both the environment and food minus that portion of a compound lost to metabolism or excretion (termed depuration). (figure 1)
- Biomagnification is what most people think of when they hear the term bioaccumulation. This is the passing of compounds from prey items into predator (or plant material to herbivore) with a resulting concentration higher in the consumer than in the individual food sources.
- Bioconcentration is the increase in a concentration of toxicant in the body of an organism directly from the environment. This is generally a passive process although certain compounds have been shown to be taken up actively. A good example is mercury compounds through the gills of fish during respiration.
- Partitioning is a term used to describe the movement of a compound from one media (i.e., air) to another (i.e., an organism or water in an aquarium).
- Detoxication describes the process of removing an agent from a site by an exogenous means. Activated carbon export is a good example relative to our tanks.
- Detoxification is a natural process that occurs when animals or bacteria/fungi convert compounds into less toxic forms. These processes can also cause activation in rare instances.
- LC50 or lethal concentration is a measure of the concentration it takes to kill 50% of the population of interest. (LD is lethal dose, EC is effective concentration; subscripted by the % mortality or effected).
Now that you have a basic set of definitions, I would like to remind you that very few compounds of real interest in our aquaria have known toxic values (LC50 or otherwise). The only data that exist tend to be derived from real world ecosystems or closed laboratory systems. Each type of animal we keep has different susceptibilities, different mechanisms for dealing with the insult, and wholly different responses to the presence of toxic chemicals. Murky estuarine systems will have a very different outcome regarding many chemicals than will a pristine reef. Organisms from different areas also have a wide range of susceptibilities. Most compounds discussed in this current context will only be represented in basic terms and generally oversimplified. Much of what I will present to you is based on organic compounds (an organic molecule is a molecule containing carbon in its structure) that have been thoroughly studied and modeled in different environments, such as pesticides and various hydrocarbons, so whether these assumptions can be extrapolated to a home aquarium or not is still unknown. Proceeding conservatively though, we should consider the worst possible scenario to preserve the animals we house.
There are a number of physical-chemical properties of individual compounds that allow for more movement into our aquaria from the surrounding environment. The water solubility of compounds is of primary concern. Some compounds have a very strong aversion to water, which precludes them from readily entering our systems (hydrophobic or lipophilic). If a compound has extremely high water solubility, this will allow it to enter the water in a tank, but frequently prevents entry of the toxin into organisms due to the presence of lipid based cell membranes. (figure 2) However, as with most things, there are complicating factors. The presence of various organic compounds and surfactants in the waters of our tanks actually assist the transfer of some organic compounds into our tanks. This has been well studied in organic contaminants such as PCBs, PAHs, and pesticides. Many inorganic contaminants and metals are taken up readily (due to small size or the presence of specific carrier molecules in the cell surface). In general, those toxicants or toxins with moderate to moderately high lipophilicity values and little to no ionic charge are the most likely to cause issues within a tank and to our animals.
Another chemical property is vapor pressure of the compound. This is directly related to water solubility via a factor called Henry’s Law (Eq. 1). Higher H values (Henry’s Law values) indicate less aqueous risk. Vapor pressure is a measure of the ability of compounds to escape from one media (i.e., water) into air. The specifics of the interactions of these physical-chemical properties will be left out of this discussion to keep it easier to follow. If you want more information, any good environmental chemistry text is a great source, please see the references.
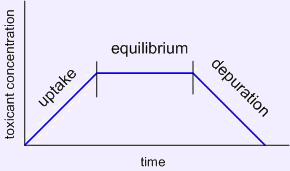
Figure 1 – Hypothetical uptake and depuration curve based on an animal placed into a tank that is polluted then moved to a clean environment or exposure such as a solvent used near a tank that peaks then declines after use.
The pH of our tanks is also a point that needs to be made in reference to toxicity. Many compounds have a state that shifts from ionic to nonionic around a given pH. This fact makes some compounds more toxic in a reef tank versus a low pH freshwater tank. Cyanide is a great example as it is nonionized (HCN) at low pH and ionized (CN–) at high pH (pKa of 9.1, which indicates 50% of each at a pH of 9.1). While cyanide as CN– is more deadly to fish, there will be a greater proportion of HCN in a tank that is at pH 8.0. Generally ionized compounds tend to be less of a threat to living systems than nonionized due to passive permeability through membranes, ammonia as NH3 or NH4+ is a prime example. Certain compounds that are charged can mimic endogenous or necessary substrates (lead mimicking calcium for example) and are readily taken up by cells.
Passage of toxins or toxicants into an organism is also highly dependant on the specific physical-chemical characteristics of a given toxicant. The ability of a compound to pass through a cellular membrane is dependant on a property much like water solubility, only in this instance it’s the reverse. Typically referred to as lipophilicity, it is a measure of how much a compound likes to dissolve in lipid membranes or hydrophobic solvents. Lipophilicity is usually measured as the octanol:water coefficient (Kow). (Eq. 2) This partitioning coefficient is a good indicator of the ability of compounds to pass into cellular membranes. As with water solubility, too much potential to dissolve into lipids causes the toxins to get stuck in cellular membranes and fat deposits and be of little importance to the inner workings of a cell. This partitioning effect can account for some forms of toxicity to cells, although the vast majority of compounds are bound away from their site of action. Those toxicants or toxins with the moderate to moderately high lipophilicity values are the most likely to cause issues within a tank. Lipophilicity is not a true concern for inorganic compounds as most are regulated by different mechanisms and have no associated Kow.
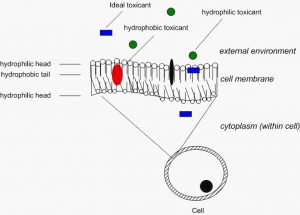
Figure 2 – Diagramatic representation of a cell membrane and typical organic toxicants ranging from fully hydrophilic to fully hydrophobic. The ideal toxicant has some degree of water and lipid solubility and no ionic charge. Note that many inorganic compounds do not follow this example.
The case of lipophilic compounds entering our tanks gets a bit more complex when you also consider what is in the water. A truly lipophilic or hydrophobic compound theoretically would not enter the system; however, our tanks have a significant amount of organics in the water column. This organic matter can serve as a transport vehicle for those compounds. Binding of organic molecules to one another is common, and the organics in our tanks can include surfactants as well as residual slime, fatty acids, and other organic byproducts from corals, fish, or plants that readily serve as transport media.
Equations:
Equation 1: H = VP/S
- VP = vapor pressure
- S = solubility constant
Equation 2: KOW = concentration in octanol/concentration in water
Sources of Toxicants
Anthropogenic sources come in all forms: vapors/aerosols, gases, liquids, and solids. Those of most concern to the average aquarium hobbyist (assuming no one tosses pennies in the tank for good luck!) are vapors/aerosols. These can be in the form of a pesticide sprayed in proximity to the tank (sprays applied outside the home are found inside in measurable quantity and within a short amount of time, Leidy et al.) or an abundance of solvent vapor from painting or cleaning. Most solvents used in household application have little overt toxicity and are unlikely to be soluble enough in water due to very high vapor pressure to partition into our tanks, but the effects on invertebrates and fishes are rarely, if ever, known. As a general rule, if a product has warnings for ventilation on it or toxicity on contact information, follow even more stringent rules than you would for human safety. There are sources more remote as well. Compounds emitted from the combustion of fossil fuels and wastes are known to be toxic in quantity and are found ubiquitously. Pesticides can also be widely distributed, although most of concern (on a large scale) is traditional and no longer in use. One such pesticide is DDT, of which we all carry detectable amounts. There is, of course, a concern with gases as well, but in general terms the conditions under which these can occur are rare as it would also be unhealthy for people. High concentrations of carbon monoxide (CO) and carbon dioxide (CO2) are possible in a well insulated house with natural gas appliances. These would have the same effect on your tank’s residents as it would have on you; namely suffocation. Cleaning solvents such as bleach (sodium hypochlorite) or ammonia are of concern in this regard. Both are volatile to a sufficient degree and water soluble. This gives them the correct combination of characteristics to be toxic to our animals. Use caution and ventilate the house when cleaning with these
products.
Metals are also a concern in a reef tank. We all know we shouldn’t use metal accessories as they corrode or rust with saltwater contact. But what you may not realize is that many metal alloys contain various metals which can become ‘entrapped’ in our tanks due to a lack of export mechanisms. Galvanization commonly employs zinc for example. While some metals are not directly toxic, there can be other indirect effects such as algal blooms with increased iron content. Many cases of nutrient limitation are not actually N or P, but in reality Fe. We all know that copper can destroy invertebrate populations. Mercury is another of concern. Although rare to find, old thermometers used mercury and a single thermometer breaking in a tank could have dire consequences (note that red liquid in thermometers is an alcohol and not nearly as dangerous as the silver mercury type). The mercury danger would also extend to the reefkeeper upon contact with the water. I will cover mercury more thoroughly in a future article. Metals and other toxic inorganic molecules can accrue due to poor input water quality (arsenic is a good example in the west and southwest), dropping metal items into the tank and allowing them to corrode, or from the atmosphere.
Another source of toxicants, although some might possibly call them toxins, is the tank itself. The nitrogen cycle produces ammonia, nitrite, and nitrate all of which are fairly toxic although via different mechanisms, especially in the marine environment. Nitrite is the most toxic on a concentration basis. A completely cycled and balanced tank with sufficient media (i.e., that with bacterially colonized surface area such as live rock and sand) is prevention enough for these inherent threats.
Movement and Clearance of Toxins
Persistence is a measure of the time a toxin or toxicant stays in a system. Scientifically noted as half-life (t1/2), it is an actual measure of the time it takes for 50% of the compound to be removed or altered to another form. Some compounds are relatively unstable and are broken down quickly, whereas others are highly resistant to breakdown. A good example of this process is seen in DDT. DDT is the parent compound, an insecticide, used to treat fields. DDT is broken down to DDE (and DDD) by various chemical and biological processes. DDE is very much more stable than DDT (although both are relatively stable hence they are now illegal to use) so that measurements taken today can still detect quantities of DDE and only traces of DDT from historical use. Persistence is affected by the above listed mechanisms of removal and metabolism/breakdown.
Chemical processes
Toxins in natural systems, whether in estuaries, the open ocean, or our aquaria, undergo movement and transformation. There exist both chemically and biologically based mechanisms that destroy or remove these compounds. Chemically, the main removal processes are photolysis – the energy from light radiation causing the breakage of molecules; hydrolysis – the destruction of chemical bonds by insertion of a water molecule; and oxidation – the process of removing electrons usually by adding groups such as oxygen molecules into a chemical structure. These processes likely occur in our tanks (assuming the UV/light intensity is enough in the case of photolysis) helping to remove some less stable organic molecules. For those aquarists using ozone or UV sterilizers, oxidation may be a leading mechanism of elimination. However, breaking organic molecules is not always beneficial. The broken molecules can be detrimental in their own right due to increased reactivity, but that is beyond the scope of this article.
Complexation can be another mechanism that keeps toxicants from having any ill effects in our tanks. Copper is a great example. In regions where there is a high degree of near anoxic space, such as internal to live rock and deep in sand beds, copper is combined readily with sulfur causing a precipitation into a solid form. This mechanism is taken advantage of in wetlands during bioremediation. This is probably not a major source of removal for our tanks, however, as ideally we keep them highly oxygenated and the surface area to water volume ratio is too low.

Figure 3 – Input and elimination routes for aquaria and fish. Green arrows represent uptake. Red arrows represent depuration (elimination).
Biological processes
Inherent in the term biological is the fact that living things go through certain cycles of growth, birth, lactation, diet and death. Each of these can have a role in removal or dilution of toxicants. If a body burden of a specific hydrocarbon is unchanged and a fish grows, the concentration per unit of body weight is reduced. Birth or deposition of eggs is a process by which a great deal of lipophilic toxicant can be shed. Fish laden with heavy stores of glycogen and fat (fish use muscle as a storage mechanism where mammals use fat cells) that are forced to fast due to breeding or migratory behaviors can see a release of compounds from those stores as they are consumed. This can be very detrimental in cases of highly lipophilic compounds in that they are suddenly available again and can cause cellular injury. The activity associated with life can be both beneficial, as is the case with removal of toxins, or detrimental as stated above.
Biological processes leading to the breakdown of toxicants or toxins use many of the same chemical means as listed above. The major difference is that organisms have a suite of enzyme driven systems for altering and eliminating these compounds from their cells. Let’s consider a toxicant such as an organophosphate insecticide (parathion is a good example). The water solubility is sufficient to allow it to enter your tank water via the air passing over its surface and concomitant contact with organics in the water. The lipophilicity is sufficient to allow the compound to enter into the body, either via ingestion or through simple diffusion. Now that the compound is within the cell, how is it removed? It obviously could simply diffuse out, but if it were carried in actively, what then? It had all the characteristics that allowed it to accumulate within the cell and those same characteristics will allow it to stay in the cell. In defense, the cell calls into action a series of enzymes that allow for alteration or metabolism of the pesticide. Small side groups are added or removed, or the molecule is split into smaller pieces. These altered molecules now have altered properties. They are typically more water soluble and have sites that allow for recognition and removal from the cell by specific transporters. This is the process of metabolism and excretion. These processes are what allow organisms to survive in an ever-changing world filled with unknown and potentially toxic compounds. There are also situations where these compounds cannot be removed readily. In this case, the potential for biomagnification exists.
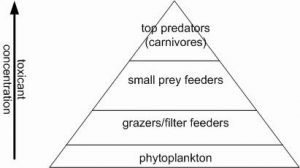
Figure 4 – Biomagnification. Increase in toxicant concentration in tissue at higher trophic levels due to dietary uptake versus biomass.
Just as in the case with excretion from individual cells, organisms are also capable of removing toxins from the body. In mammals, the bile system is used to clear compounds from the bloodstream via the liver. The bile then flows outward into the intestines where some compounds are reabsorbed and others are excreted. This process is much the same in all vertebrates.
Plants may serve as a source of export as well: Macroalgaes specifically as they are readily pruned back and physically removed from the system. Macrophytes, however, are not a good primary source of elimination. Organic uptake tends to be limited to smaller organic compounds such as sugars, amino acids, nucleic acids, peptides, and various others. In order for plants to be effective as an elimination route, bacteria and other micro-life must convert the larger molecules into smaller molecules. This service as a secondary export is useful, but not in the context of detoxication.
Another facet of biological metabolism and elimination is the presence of bacteria and fungi. In most natural systems, and likely, in most reef tanks, the biomass of the bacterial and fungal populations far exceeds that of any other biological agent. These bacteria and fungi are responsible for the low residual free nitrogen oxide levels as well as many other highly specific and important functions. Among those functions is the ability to convert compounds to their mineralized state. Mineralization is the process, whether driven by a single species or by many species, of taking a chemical and reducing it to its smallest components, such as H2O, CO2, O2, etc.
Remember as well that our reef tanks are not open systems with backing by heavily populated mangrove or estuarine systems. Our closed systems, while we attempt to mimic natural processes, prohibit complete mimicry. Microbial action and recycling of nutrients help us in our attempt to provide oligotrophic waters, although export remains critical. A striking example of the need for export mechanisms (not just transformation processes) is in the bacterial recycling of conjugated toxins. Vertebrates (and likely other higher organisms as well) have systems, as previously mentioned, for conjugating toxins to help eliminate and reduce toxicity of some compounds. The system works very efficiently to prevent toxicity in the organism. However, many conjugates are a substrate for bacterial growth and are cleaved from the parent molecule, thereby freeing the original toxin from its altered state and allowing it to once again be available for uptake.
Mechanical processes
While the chemical processes above will occur in our tanks, there are other means by which we can eliminate toxins and toxicants so as to make their presence less deleterious. Activated carbon functions well in this regard. Activated carbon is known to bind organic molecules (note that it will not help with nitrogen cycle components or many other inorganic compounds). It has commonly been employed in clarifying water such as removing the yellow coloration from older tanks. The molecules which cause yellowing in the water are organic wastes, typically large organic acids, which readily bind to activated carbon. Carbon is consumed once all of the available sites are covered with these organics or a biofilm (bacterial mat) develops on the granule surfaces preventing diffusion through the pores. Carbon is a highly useful, readily available, and easy to use method for removal of smaller organic contaminants. I highly recommend at least occasional use of carbon for the removal of wastes and other released compounds, especially those released by corals competing for space in our closed systems.
Another common method of organic removal is via a protein skimmer. This method has many of the same properties as carbon usage, only by a different chemical interaction. This takes advantage of the fact that organics will cling to one another, in function, much the same process as activated carbon. In the end, it is another great mechanism for removal of toxins.
Conclusions
In conclusion, I would like everyone to come away with a better understanding of what could be affecting our tanks. We have all either been involved in or known someone who had ‘something’ happen in the tank and never been able to identify a source of the problem. Be aware of the consequences of using aerosols and other household cleaners and solutions in proximity to your aquaria. Be smart. The processes, from input to export are all dynamic. This manuscript is to make you aware of possibilities, but don’t forget that the dynamics of the system may eliminate sources of stress before they ever have an obvious effect. Here are common mistakes that people make and their cures:
- Spraying glass cleaner directly on the aquarium.
- Instead, spray it onto a rag or paper towel well away from the tank. Glass cleaners frequently contain small amounts of ammonia, but more importantly have various alcohols of unknown toxicity.
- Use of pesticides in proximity to a tank. Many pesticides travel very well, especially when put into aerosol form. Our bodies deal with the toxicant without ill effect in most instances, but our fish cannot do so as readily.
- Instead, use less toxic forms of insect control or very careful application (the 3 foot spray instead of the 22 foot!).
- Cooking in hot oil with a tank nearby. This vaporizes the oils which then condense on cool surfaces. This type of toxicant can actually kill birds in acute exposures.
- Always ventilate well and place tanks away from the kitchen.
- Allowing pesticide application to the entire house. Even spot applications spread throughout the air in a house quickly. Many pesticides are extremely toxic to aquatic life, especially invertebrates.
- Stick to low toxicity pesticides or remove the tank prior to application (I suggest for months afterwards of heavy application that carbon be used and changed weekly).
In summary, a toxin must have three properties to be of concern. First, it must be toxic. If a compound is present, but has no toxic consequences, then it is of little concern. Secondly, it must be available. Availability includes lipophilicity and vapor pressure. If a compound immediately leaves the aqueous environment, it is of little concern. Thirdly, the toxin must be persistent. If a chemical has a half-life of only minutes, it will not likely be of concern.
Remediation of a tank that has been polluted by a toxin or toxicant is highly dependant on the specific compound involved. Basically, any organic compounds would best be dealt with using activated carbon and water changes. Water changes serve to dilute most toxic compounds, but remember that stress can occur from environmental changes which amplify toxic effects. Be certain to match all parameters to the tank’s current levels. The carbon acts as a sink for the compounds, removing them from being active against your animals. As an example, I have seen a number of cases where sand eating holothurians (cucumbers) have been assaulted by powerheads and have released a fairly potent toxin that hits vertebrates. Immediate use of carbon seems to be critical in saving fish affected by the toxin, although if ingestion of cucumber flesh occurs, there is almost no option to save the fish.
While this article deals with the various processes behind poisonous compounds entering our tanks and their movement and removal from tanks, it listed only mild examples of various toxins and toxicants. The next articles in the series will deal with specific examples that are more relevant to our home aquaria, including fish, invertebrates, alga, and anthropogenic compounds.
References
- Wright CG, Leidy RB, Dupree, Jr HE. “Chlorpyrifos in the Air and Soil of Houses Eight Years after Its Application for Termite Control.” Bull. Environ. Contam. Toxicol. 1994. 52:131-134
- McClintock JB, Baker BJ, Eds. Marine Chemical Ecology. CRC Press, Boca Raton, FL. 2001
- Halstead, BW. “Poisonous and Venemous Marine Animals of the World,” 2nd Ed. The Darwin Press, Inc. Princeton, NJ. 1988
- Hodgson, E. Modern Toxicology. Appleton & Lange. Norwalk, Connecticut. 1994
- Meier, J. White, J. Eds. 2000. Handbook of Clinical Toxicology of Animal Venoms and Poisons. CRC Press, NY. NY.
0 Comments