Toxins are the natural products of microbes, plants, and animals typically used as defensive mechanisms, to prevent competition, as antibiotics, or in order to subdue prey. Some toxins are happenstance, meaning they exist for another purpose and serve as a toxin against some species, such as steroid based saponins. Toxins, like toxicants, have an array of effects, mechanisms and different outcomes in different species. A cucumber’s toxin, for example, will affect fish but have no consequence as concerns corals or clams.
With these variables in mind, this discussion will highlight the importance of toxicology as it relates to aquarium husbandry. For a review of many terms relevant to this discussion, see the June 2002 issue of Advanced Aquarist magazine. Many factors play into unexplained deaths of animals in our tanks, so assigning blame to one factor is rarely easy to do. Infection by bacteria, fungi, or virus is always a possibility as is the presence of toxins and toxicants or even something as supposedly obvious as ectoparasites. This information is presented to give you, as a concerned aquarist, an idea of the complexity and sheer volume of possibilities inherent in the aquatic environment.
After reviewing a substantial volume of literature for this article series it is apparent that money is primarily spent to investigate human intoxication, effects, and pharmacological attributes. Comparatively little effort has been expended to determine natural system interactions. However, molecular mechanisms of action can still provide us with a glimpse of how these compounds work in relation to their immediate environment.
As an example of the interaction of the environment and toxicity, there is a relevant example referenced in Halstead. In summary, there are studies that show that aquatic environments with large populations of the arrow worm Sagitta setosa are poor in other organisms, including fish. In contrast, water rich in S. elegans is rich in everything from phytoplankton to fish. These studies indicate that organisms grown in the water with S. setosa have a higher degree of abnormal development and lowered viability. The same has been reported with the worm Bonellia and the sea star P. helianthoides. While no direct toxins have been found in the cultures, adding the water from these cultures to urchin cultures has shown the same results, indicating that indeed there is a toxin involved. If we assume our tanks are much like the natural environment we strive for, this indicates that if we select for or shift the balance of organisms (or even introduce unwanted species) we could be detrimentally affecting our tank inhabitants.
To give a general picture of recent trends in the science of marine natural toxins, consider the following reports by Munro et al. In 1986, 186 biologically active compounds had been reported in the scientific literature (specifically the MarinLit database, which is dedicated to marine natural products). In 1997, 713 compounds had been listed. At the time of the author’s most recent report in mid 1998, there were already an additional 484 new papers detailing bioactive marine compounds. The field has seen a recent explosion in data with the following order of prevalence: Porifera à Cnidaria à Chromophycota à Rhodophycota à Mollusca à Chordata à Echinodermata.
The organization of this article follows a taxanomic table. Those entries especially relevant to our tanks have been given special attention. Many examples of invertebrates are included that are not specifically relevant to human intoxication, although may play a role our closed systems.
Protists/Single Celled Organisms
This group includes amoeba, foraminifera, sporozoans, and the single celled alga, most of which have unknown toxic products or none discovered as of yet. The one exception is the alga. Algae as a whole produces what are termed phycotoxins. Dinoflagellates are single celled, flagellated algae that have a wide range of toxic outcomes and a wide range of life stages, some of which are toxic, some of which are not. Gymnodenium breve (now called Karenia brevis ) produces the so called ‘red tide’ which causes the death of many fish and shellfish. The responsible toxin, brevetoxin, also causes respiratory irritation to humans when aerosolized. Most dinoflagellates, in small numbers, will not be an issue as the toxin has a moderate half life. Other toxic dinoflagellates have been described including Pfiesteria sp., Gambierdiscus sp. (which is implicated in ciguatera poisoning) and Gonyaulax sp. The abundance of literature surrounding the toxic alga’s precludes too much detail in this review, but the following web references may be of value:
http://www.redtide.whoi.edu/hab/default.html http://www.agr.gc.ca/archives/phycotoxins.html.
Dinoflagellates are not the only toxic algal species. Many blue-green alga (also termed cyanobacteria) such as Microcystins produce known toxins. There are also reports of diatoms or like organisms killing snails when consumed in reef tanks, although this is as yet unverified. Cyanobacteria are also known to produce toxins. In a recent presentation at a Harmful Algae Bloom meeting in St. Pete Beach, FL., there were presentations showing avoidance behaviors by rabbitfish, tangs, and urchins to Lyngbya sp. These cyanobacters produce ypaoamide (which is the likely deterrent in the experiments). Alongside the noted avoidance behaviors of the various fish, there are specialized feeders that help deal with the seasonal blooms, such as B. leachii and S. striatus nudibranchs.
This discussion becomes relevant in a number of ways when extracted back to the home aquarium. Algal blooms, while rare, are an occurrence in tanks and can be comprised of variable species. Further, alga serve as a source of nutrition for clams, corals, and other invertebrates. A bloom of toxic algae could suddenly cause the death of one genera or even one species that selectively feeds on or has a specific susceptibility to a given algae.
In relation to human relevance, if a bloom occurs and an aquarist is exposed to aerosol or in contact with the water, it can cause adverse reactions. An example occurred in a lab at NC State University in which lab technicians were severely affected neurologically when dealing with a Pfiesteria culture. Most environmental, algal based intoxications occur as a result of consumption of fish or shellfish or contact with aerosol near the ocean. Shellfish poisonings, thought to have an algal etiology, include paralytic shellfish poisoning (saxitoxin), amnesic shellfish poisoning (domoic acid), diarrheic shellfish poisoning (okadaic acid), and erythematous (internal bleeding) shellfish poisoning.
Porifera/Sponges
The rapid development of the pharmaceutical market has brought about a bloom of information regarding various toxins native to the sponges. Evidence that sponges contain many toxins is easily noted by empirical observation in that algal overgrowth is rarely seen and predation rates are low. Recall that the dose is frequently the only factor segregating pharmaceutical use from intoxication.
Sponge toxins tend to fall into a few different broad categories: cytotoxic, neuroactive, or receptor interaction based compounds. These compounds can be steroid based, peptide/protein based or novel organic chemicals. Cytotoxic compounds are those that kill, rupture or cause the derangement of normal cell function leading to cell termination. Many of the cytotoxic compounds have been successfully used to treat diseases such as cancer, malaria or bacterial infection. Neuroactive compounds are those that interact with nervous system cells directly, such as interruption of synapse signaling (such as cholinesterase enzyme inhibition or potassium pump dysregulation). Receptor based interactions (many of the peptides found in sponge extracts are examples) occur when compounds directly bind to various cell receptors causing a downstream chain of events that are normal in a cell’s routine but at non- normal times or in excess. These are by no means the only interactions and are only generalizations as to the actual activity of the various toxins present, but show the diversity of the toxins formed naturally by sponges. Alongside the variable activity of the toxins, each sponge may produce a host of different compounds that are in some way toxic. The variability, as with other organisms, allows for stronger resistance of predation or competition. The following table illustrates the magnitude of the activities and presence of some of the toxins and pharmaceutically active compounds found in the previous few years:
Compound Class | Compound Name | Origin | Compound Effect |
---|---|---|---|
diterpene | axinyssene | Axinyssa sp. | mild cytotoxic |
brominated alkaloid | nakirodin A | Veronid sp. | |
sesterterpene | luffariolide (H&J) | Luffariella sp. | |
calyculinamide | geometricin A | Luffariella sp. | moderate cytotoxicity,
anti-algal |
hepta(peptide) | leucamide | Leucetta sp. | |
alkaloid | Leucetta sp. | cytotoxic | |
sterol (sulfur containing) | lembehsterols (A&B) | Petrosia sp. | inhibit cell growth |
enzyme | cyclotheonamide (E4&E5) | Ircinia sp. | |
alkaloid | axinellamine (A&D) | Axinella sp. | bacteriocidal, cytotoxic |
polyacetylene | lembehyne A | neuro-active | |
hydroquinone | adociasulfate | Adocia sp. | cytotoxic |
alkaloid | Hyrtioserecta sp. | enzyme inhibition | |
sterol acetate | agosterol A | cytotoxic | |
brominated alkaloid | suberedamine (A&B) | Suberea sp. | |
sesquiterpene | ulosin (A&B), others | Ulosa sp. | |
isonitrile terpene | antimalarial | ||
polyketide | callystatin A | high cytotoxicity | |
enzyme inhibitor | halenaquinone | cytotoxic, enzyme inhibition | |
enzyme inhibitor | hennoxazole A | antimalarial | |
cyclosterol | Strongylophora sp. | ||
steroid/cholesterol | 24-isopropylcholesterol
24-isopropenylcholesterol |
Epipolasis sp. | |
sesquiterpene | pelorol
ilimaquinone |
Dactylospongia sp. | low antimicrobial and low cytotoxicity |
alkaloid | pyrinodemin (A-D) | Amphimedon sp. | cytotoxic |
sesterterpene
alkaloid |
isodehydroluffariellolide
homofascaplysin A fascaplysin |
Hyrtios sp. | |
B2-receptor antagonist | S1319 | neuro-active | |
brominated alkaloid | biprasin | Dysidea spp. | caffeine-like, calcium release |
brominated alkaloid | mukanadins (A-C) | Agelasnakamurai sp. | |
steroid | xestoquinone | Xestospongia sp. | cytotoxic |
brominated fatty acid | Xesospongia sp. | ||
sesterpene | Strepsichordaia sp. | ||
brominated alkaloid | Homaxinella sp. | ||
brominated alkaloid | Hymeniacidon sp. | ||
cyclic polyketide | Plakortis sp. | ||
enzyme inhibitor | dragmacidin | Spongosorites sp. | |
aeroplyseinin 1 | Aplysina sp. |

Figure A- Principal morphological features of the a) undischarged and b) discharged nematocysts. This represents only a single type of nematocyst, but portrays the basics of function. Taken from Halstead 1998.
Coelenterates/Cnidarians
This group of animals is of greatest interest to most reef aquarists. Included are the hydroids, jellyfish, anemones and corals. Most members of this group, however, are innocuous to humans, although most contain nematocysts (figure A) which can cause dermatitis in sensitive individuals. Many have felt the not-so-gentle caress of a fire coral! There are approximately 40 species known to be dangerous to people. A great many more are likely dangerous to tank inhabitants. This is especially important when mixing animals from different regions in a single aquarium. An Atlantic Condylactis anemone, for example, has a very powerful sting and readily consumes many Indo-Pacific fish on first contact.
The nematocysts of this group are still under investigation as to their mechanism of action. Generally, the nematocyst is a cell containing a sharp, spearheaded portion. When contacted with certain chemicals or by touch, the spear is fired outward. Toxin is packed either around the spine or within a gland near the nematocyst. This is both a defensive mechanism and a method for capturing prey.
Hydroids – This group includes not only the free swimming, but colonial polyps as well as sedentary species, such as Millipore (the well named fire corals). The toxic factors in these species have hemolytic activity and cause pain.

Table 1 – Species list of ceolenterats that are known to produce toxins. The presence of vastly different toxins through many family and genus of organisms is astounding. This is a partial list. Taken from Halstead 1998.
Jellyfish – For an animal that is less than 5% solid material, jellyfish have one of the most potent venoms in the reef world. The effects of stings by these species in people ranges from mild dermatitis to death. Death is usually due to shock and drowning or anaphylactic reactions. Recovery can take from days to months depending on severity.
Anthozoans – This group has two classes of interest to aquarists, the Alcyonaria (soft corals, sea fans, etc) and the Zoantharia (anemones and true corals).
Anemones have been found to be toxic upon ingestion unless thoroughly cooked. Anemone consumption has even lead to death in children in tropical countries (specifically Rhodactis sp. using the old nomenclature). Anemones, it has been discovered, are much like sponges in the array of toxins present in their tissues. Determination of the localization of toxins, however, whether nematocyst or digestive tissues, is still a hotly contested area of debate. Cytolysins seem to be the largest group of toxins found in anemones.
The true corals are only recently coming under more scrutiny as far as their toxic components, although as with all cnidarians, they appear to have both peptide and chemical toxins. The toxins, however, are very more difficult to extract in quantities relevant for research due to low amounts of soft tissue on most scleractinian corals. Incidences of coral envenomation can result in ulcer development and become exceedingly painful, even so severe that it becomes disabling to the individual, although recovery usually occurs. This is something to consider regarding tank maintenance.
Instead of addressing each species independently, see the table from Halstead (table 1). More information exists, but the variety of toxins and pharmaceutically active components mirrors that from this older table.
Known pharmacological effects of coelenterate envenomations are wide ranging, from simple pain to interruption of nerve conduction, muscle contraction, and rupture of cells. (table 2) Most of the Cnidarian toxins fit into a category describable as active. The toxins range in effectiveness, but most are aimed at subduing prey or active defense. The toxins typically function by rupturing cells (cytolysis) as in the pore forming compounds such as equinatoxin from Actinia anemones and by hemolysis like those from fire coral Millipore’s nematocysts or they function to alter neurological signals as in the Bundosoma granulifera anemone’s granulitoxin.
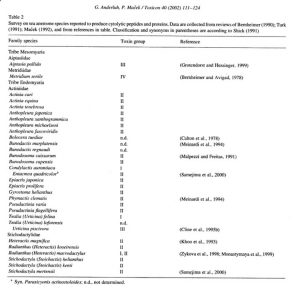
Table 2 – Recent publication showing anemone species producing known cytolytic toxins. Table taken from Anderlugh, G., Macek, P. 2002. Toxicon 40. pg 113.
Treatment for stings (in humans) is based mainly on effects. However, the common misconception of urinating on stings is false and increases nematocyst discharge making symptoms worse. Vinegar and meat tenderizer work well by breaking down the proteins involved. Hydrogen peroxide is highly suggested to debride coral wounds and prevent secondary infection after exposure, both at home and at the beach.
Treatment of tank occupants is not really an option as the amount of venom injected on a comparative scale is high and handling of fish is usually more detrimental than helpful. Mild stings to fish are usually of little concern, but some anemones have a powerful enough sting to paralyze fish. This is especially a concern as regards species that rest on the substrate such as gobies, blennies, and hawkfish.
Echinoderms
This group includes the sea stars, brittle stars, urchins, and cucumbers and contains some 5,300 species. The brittle stars and sea stars are generally not known to be toxic, with the one exception, the Crown of Thorns ( Acanthaster planci ) star. This sea star has glands located in its epidermis and a venom apparatus laden with a toxin termed asterotoxin. This toxin, in people, is reported to cause extreme pain, redness, swelling and can even lead to vomiting, numbness, and paralysis. Asterotoxin is a saponin that is extremely toxic to fish. Saponins of many kinds are found in both cucumbers and sea stars, but in variable forms and quantities.

Table 3- Compounds of coelentera origin. Note the variety and variability between the various species. These compounds serve many roles, including defensive, antibiotic, and offensive (prey capture). Taken from Halstead 1998.
The urchins are more commonly venomous and have one of two types of venom apparatus: spines (many tipped by venom glands, such as with Diadema ) or pedicellariae, which are located internal to the spines and have a form of jaw- or scissor-like pincers. Intoxication by urchins is most commonly from stings, but can also be from eating gonads or roe from certain species at certain times of year (ootoxic). The needle-like spines of Diadema or Echinothurid urchins can readily penetrate gloves and typically leave a purple-black stain just beneath the skin that can last for weeks. A common response to stings is immediate burning and the host of typical inflammatory responses. In severe cases, partial paralysis and irregular heartbeat can occur. Secondary infection from the puncture wound is also a real concern. In fish, the response would be much the same. Recovery usually occurs in 1-6 hours. Pedicellariae based stings can be very potent and the effects can range from
respiratory distress to nervous system interruption (including paralysis).
Cucumbers, or holothurians, produce a generic toxin named holothurin or holotoxin. Most cucumbers store this toxin in the Organs of Cuvier, which means that envenomation is not a concern. Intoxication by cucumbers occurs via contact with ejected liquid (known to cause blindness or dermatitis) or from ingestion. The discovery of holotoxin was made in 1929 by a Japanese scientist named Yamanouchi. He noted that fish placed into tanks with cucumber tissue extracts died within minutes. The toxin is mainly in the body wall and causes loss of motor control (coordination and reflexes) which can end in paralysis. As with most animal toxins, the variable components of the cucumber poison have different effects. Bleeding or hemorrhage of the lungs has been noted if the toxin is introduced directly to the bloodstream of mammals. Twenty four of 27 Indo-Pacific species tested were toxic. That said many of these same species are considered edible as stomach acid neutralizes the toxin. The four
“worst” species are: H. axiologa, H. atra, S. variegates, and T. ananas. The toxin affects fish by entering via the gills. Cucumbers also release toxins under stressful conditions, such as when physical trauma occurs. As a side note, the toxin has been noted to kill planaria at 0.00001% concentration! The various other toxins contained in cucumber flesh have been described as both anti-metabolic and anti- cholinergic. As with sea stars, the main components appear to be saponins. In this case, however, they are glycosides, which are uncommon in animals (sea stars carry steroidal saponins).
Mollusks
Snails, bivalves (clams) and octopi make up this family of organisms. Bivalves are typically not directly toxic but serve as major vectors for algal based toxins, such as ciguatera, and the various effectors of shellfish poisoning. Callistin and venerupin poisonings from their consumption are common. The two species reported to be toxic in and of themselves are Tridacna gigas and maxima, but these also may be due to accumulation of toxins from an algal diet as well, as no toxin has been isolated and identified.
The snails and slugs have a few members who are both venomous and poisonous. Whelks, for example, have poison salivary glands but no venom apparatus making them poisonous but not venomous. This is used in penetrating oyster and other bivalve shells for consumption. These are not a threat to humans, but could be a threat to bivalves within the tank. The poison contained in their glands is a tetramine with curare-like properties (which cause loss of motor control). One commonly found toxin is called surugatoxin and another is IS- toxin. Cone snails are the most deadly of the mollusks, having well developed venom apparatus adapted from radula teeth. This adapted tooth is a long harpoon, complete with barbs on the tip. The harpoon is stored in a chamber in the top of the foot and loaded forward at need. A few of the species in this group don’t have harpoons at all, but release the toxin into the water column near possible prey causing paralysis. The main ingredient in the Conidae toxin is a peptide of small size (13-15 amino acids in length). Most human intoxications are a result of handling these pretty shells while the animal within is still alive and getting stung as a result. The effect in vertebrate animals is severe paralysis usually leading to asphyxiation. Accidental introduction of this genus to a home aquarium would be very dangerous as concerns all smaller fish. There are reports in the literature of turbo snails also containing ciguatera-like toxins, although these are of little relevance to the aquarist. Murex or Rock Shells, once used as a source for Tyrian purple dye also have a venom gland, although little information exists concerning this snail’s toxicity.

Figure B2: glycoside based saponins from Echinoderms. The chemical origins for these various toxins is very different, yet the effects from intoxication are much the same. Taken from Halstead 1998.
Nudibranchs, or sea slugs, have a few defensive toxins. Some isolated toxins from nudibranchs mirror those common to the corals. Terpenes and nerve interruptive compounds have been found in a few sea slug species in the eggs and skin. The most common form of defense is not native to the nudibranchs, but rather involves capturing nematocysts from other stinging organisms that they consume as prey and using these nematocysts by storing them in cnidosacs for future use. Some, however, do secrete toxins called crinotoxins, although they are no real threat to a tank or to humans in small quantity. The purified toxin is very mild.
The octopuses have a toxin as well. Most bites from octopuses are very mild and only cause a burning/itching sensation in the limb, although severe cases have been reported that include ‘severe feeling of detachment and paralysis’. The most venomous of the group are H. lunulata, O. macropus, and O. vulgaris. The Blue Ring Octopus ( Hapalochlaena lunulata ) appears in the trade on occasion and should be respected and only attempted by advanced aquarists with the proper tank setup and understanding.
Worms
Platyhelminthes is the only class of flatworms worth noting as being poisonous. The Turbellaria family are free living types that have a chemical defense system to ward off predation. The nature and structure of the toxin(s) are unknown.
Ribbon worms (nemerteans) are worms with well developed extensible proboscis with a stylet on the tip. This structure is typically used for feeding and no reports are available on toxic effect on people. As concerns the aquarist, it is a powerful feeder and would not make for a peaceful tank.
Annelids or segmented worms are those we all know well. The ‘bristle worms’ as the most common marine annelids in reef tanks are described, typically only cause irritation due to setae stings. The setae are hollow and possibly filled with mild venom. The toxin is generally just a dermal irritant to humans. Many fish feed on these worms, and so aside from specific species, which feed on corals, these are beneficial to the reef tank as both a detritivore and a foodsource. Bloodworms also fall into this category. Glycera dibranchiata, the common bloodworm, can inflict painful bite wounds. Venom glands have been noted in association with the jaws of these worms. With a range on the East coast of the United States from North Carolina to Northeastern Canada they are a concern for people collecting sand or rock locally. Annelids occasionally contain a toxin named nereistoxin that affects the nervous system.
Arthropods have only been found to be ootoxic (reproductive tissues) and are not relevant to the aquarist.
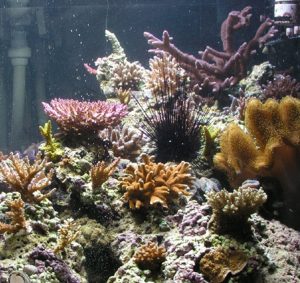
Figure D- An aquarist’s reef tank. Note the presence of the diadema urchin. Careless work in the tank could result in a painful encounter. (Image from Brian Ferguson)
Bryozoans
Upon contact with skin, Bryozoans or Moss Animals, can cause a fairly severe dermatitis and burning sensation, although the toxin and the mechanism of action are both unknown for these colonial animals. These are found in temperate and tropical environments worldwide.
Removal Processes
The presence of toxins in our tanks, those produced by corals or invertebrates from within the system, might be of serious concern or of little concern when considering processes that work toward the removal of these toxins. For example, the warfare of corals competing for space might have a detrimental effect on anything ‘downstream’ of the coral releasing its toxin but has little effect beyond that point. On the other hand, an algal bloom or a cucumber suddenly coming into contact with a powerhead will release toxins that are extremely detrimental in even minute quantities. Luckily, there are processes, both chemical and biological, that help us in our quest to provide a stress free environment to our inhabitants. For a more thorough explanation, see the earlier link to the first article in this series. In short, chemical interactions occur with UV light, oxygen, and other chemical agents that break, bind up, or remove the toxin. In a biological context, organisms can prevent intoxication by several means. Stopping the uptake of a toxin, breaking it down upon entry into the body, or even release of chemical agents such as thiols (sulfur containing) into the water column can all serve a function in preventing intoxication at the individual level.
The aquarist can also minimize or eliminate intoxication threats by regular water changes, use of activated carbon in the system, and strong protein skimming. Just as important, understanding the organisms you add to a tank will go a long way to preventing unwanted problems with water quality. Simple measures, such as keeping powerheads away from sand beds where holothurians dwell or screening overflows to prevent physical trauma to various inhabitants should also be a primary concern.
*Please note that I am not an invertebrate biologist or taxonomist. Taxonomy changes too quickly and literature frequently becomes outdated. All references made herein were correct to my knowledge, although due to the advancement of science may not be the most recent discoveries. All information concerning toxins and their mechanisms of action should be up to date.
References
General Toxicology
- Currie BJ. Clinical toxicology: A tropical Australian perspective. THERAPEUTIC DRUG MONITORING 2000, Vol 22, Iss 1, pp 73-78
- Faulkner DJ. Marine pharmacology. ANTONIE VAN LEEUWENHOEK INTERNATIONAL JOURNAL OF GENERAL AND MOLECULAR MICROBIOLOGY 2000, Vol 77, Iss 2, pp 135-145
- Fenner PJ. Dangers in the ocean: The traveler and marine envenomation. I. Jellyfish. JOURNAL OF TRAVEL MEDICINE 1998, Vol 5, Iss 3, pp 135-141
- Gleibs S; Mebs D. Distribution and sequestration of palytoxin in coral reef animals. TOXICON 1999, Vol 37, Iss 11, pp 1521-1527
- Halstead, BW. Poisonous and Venemous Marine Animals of the World, 2nd Ed. The Darwin Press, Inc. Princeton, NJ. 1988
- Hodgson, E. Modern Toxicology. Appleton & Lange. Norwalk, Connecticut. 1994
- Meier, J. White, J. Eds. 2000. Handbook of Clinical Toxicology of Animal Venoms and Poisons. CRC Press, NY. NY.
- Munro MHG, Blunt JW, Dumdei EJ, Hickford SJH, Lill RE, et al. The discovery and development of marine compounds with pharmaceutical potential. JOURNAL OF BIOTECHNOLOGY 1999, Vol 70, pp 15-25
- Whittle K; Gallacher S. Marine toxins. BRITISH MEDICAL BULLETIN 2000, Vol 56, Iss 1, pp 236-253
Single Celled Organisms
- Lehane L; Lewis RJ. Ciguatera: recent advances but the risk remains. INTERNATIONAL JOURNAL OF FOOD MICROBIOLOGY 2000, Vol 61, Iss 2-3, pp 91-125
- Matsubara K; Hori K; Matsuura Y; Miyazawa K. A fibrinolytic enzyme from a marine green alga, Codium latum. PHYTOCHEMISTRY 1999, Vol 52, Iss 6, pp 993-999
- Sato Y; Oda T; Muramatsu T; Matsuyama Y; Honjo T. Photosensitizing hemolytic toxin in Heterocapsa circularisquama, a newly identified harmful red tide dinoflagellate. AQUATIC TOXICOLOGY 2002, Vol 56, Iss 3, pp 191-196
Sponges
- Aoki S, Matsui K, Takata T, Hong W, Kobayashi M. Lembehyne A, a spongean polyacetylene, induces neuronal differentiation in neuroblastoma cell. BIOCHEM BIOPHYS RES COMMUN 2001 Nov 30;289(2):558-63
- Aoki S, Naka Y, Itoh T, Furukawa T, Rachmat R, Akiyama S, Kobayashi M. Lembehsterols A and B, novel sulfated sterols inhibiting thymidine phosphorylase, from the marine sponge Petrosia strongylata. CHEM PHARM BULLETIN 2002 Jun;50(6):827-30
- Aoki S, Ye Y, Higuchi K, Takashima A, Tanaka Y, Kitagawa I, Kobayashi M. Novel neuronal nitric oxide synthase (nNOS) selective inhibitor, aplysinopsin-type indole alkaloid, from marine sponge Hyrtios erecta. CHEM PHARM BULL 2001 Oct;49(10):1372-4
- Aoki S, Chen ZS, Higasiyama K, Setiawan A, Akiyama S, Kobayashi M. Reversing effect of agosterol A, a spongean sterol acetate, on multidrug resistance in human carcinoma cells. JAPANESE JOURNAL OF CULTURE RESEARCH 2001 Aug;92(8):886-95
- Amagata T, Usami Y, Minoura K, Ito T, Numata A. Cytotoxic substances produced by a fungal strain from a sponge: physico-chemical properties and structures. JOURNAL OF ANTIBIOTICS 1998 Jan;51(1):33-40
- Bunc M; Strupi-Suput J; Vodovnik A; Suput D. Toxic effects of head-to-tail 3-alkylpyridinium polymers isolated from the marine sponge Reniera sarai in rat. TOXICON 2002, Vol 40, Iss 7, pp 843-849
- Capon RJ, Rooney F, Murray LM, Collins E, Sim ATR, Rostas JAP, Butler MS, Carroll AR. Dragmacidins: new protein phosphatase inhibitors from a southern Australian deep-water marine sponge, spongosorites sp. JOURNAL OF NATURAL PRODUCTS 1998 May;61(5):660-2
- Fontana A, Ishibashi M, Shigemori H, Kobayashi J. New cyclic polyketide peroxides from okinawan marine sponge plakortis sp. JOURNAL OF NATURAL PRODUCTS 1998 Nov;61(11):1427-9
- Fujiwara H, Matsunaga K, Saito M, Hagiya S, Furukawa K, Nakamura H, Ohizumi Y. Halenaquinone, a novel phosphatidylinositol 3-kinase inhibitor from a marine sponge, induces apoptosis in PC12 cells. EUR JOURNAL PHARMACOLOGY 2001 Feb 9;413(1):37-45
- Goclik E, Konig GM, Wright AD, Kaminsky R. Pelorol from the tropical marine sponge Dactylospongia elegans. JOURNAL OF NATURAL PRODUCTS 2000 Aug;63(8):1150-2
- Gross H, Kehraus S, Konig GM, Woerheide G, Wright AD. New and biologically active imidazole alkaloids from two sponges of the genus Leucetta. JOURNAL OF NATURAL PRODUCTS 2002 Aug;65(8):1190-3
- Hirano K, Kubota T, Tsuda M, Mikami Y, Kobayashi J. Pyrinodemins B-D, potent cytotoxic bis-pyridine alkaloids from marine sponge amphimedon sp. CHEM PHARM BULL 2000 Jul;48(7):974-7
- Hori A, Imaeda Y, Kubo K, Kusaka M. Novel benzimidazole derivatives selectively inhibit endothelial cell growth and suppress angiogenesis in vitro and in vivo. CANCER LETTERS 2002 Sep 8;183(1):53-60
- Inaba K, Sato H, Tsuda M, Kobayashi J. Spongiacidins A-D, new bromopyrrole alkaloids from hymeniacidon sponge. JOURNAL OF NATURAL PRODUCTS 1998 May;61(5):693-5
- Ito M, Hirata Y, Nakamura H, Ohizumi Y. Xestoquinone, isolated from sea sponge, causes Ca(2+) release through sulfhydryl modification from skeletal muscle sarcoplasmic reticulum. J PHARMACOL THER 1999 Dec;291(3):976-81
- Jahn T, Konig GM, Wright AD. Three new scalarane-based sesterterpenes from the tropical marine sponge strepsichordaia lendenfeldi1 JOURNAL OF NATURAL PRODUCTS 1999 Feb;62(2):375-7
- Kalaitzis JA, de Almeida Leone P, Harris L, Butler MS, Ngo A, Hooper JN, Quinn RJ. Adociasulfates 1, 7, and 8: New Bioactive Hexaprenoid Hydroquinones from the
- Marine Sponge Adocia sp. JOURNAL OF ORGANIC CHEMISTRY 1999 Jul 23;64(15):5571-5574
- Kehraus S, Konig GM, Wright AD. A new cytotoxic calyculinamide derivative, geometricin A, from the Australian sponge Luffariella geometrica. JOURNAL OF NATURAL PRODUCTS 2002 Jul;65(7):1056-8
- Kehraus S, Konig GM, Wright AD, Woerheide G. Leucamide A: a new cytotoxic heptapeptide from the Australian sponge Leucetta microraphis. JOURNAL OF ORGANIC CHEMISTRY 2002 Jul 12;67(14):4989-92
- Kehraus S, Konig GM, Wright AD. New carbonimidic dichlorides from the Australian sponge Ulosa spongia and their possible taxonomic significance. JOURNAL OF NATURAL PRODUCTS 2001 Jul;64(7):939-41
- Kirsch G, Kong GM, Wright AD, Kaminsky R. A new bioactive sesterterpene and antiplasmodial alkaloids from the marine sponge hyrtios cf. erecta. JOURNAL OF NATURAL PRODUCTS 2000 Jun;63(6):825-9
- Kobayashi M, Kitagawa I. Marine spongean cytotoxins. J NATURAL TOXINS 1999 Jun;8(2):249-58
- Kobayashi M, Mahmud T, Tajima H, Wang W, Aoki S, Nakagawa S, Mayumi T, Kitagawa_ Marine natural products. XXXVI. Biologically active polyacetylenes, _ adociacetylenes A, B, C, and D, from an Okinawan Marine sponge of Adocia sp. CHEM PHARM BULL 1996 Apr;44(4):720-4
- Kobayashi M, Wang W, Ohyabu N, Kurosu M, Kitagawa I. Improved total synthesis and structure-activity relationship of arenastatin A, a potent cytotoxic spongean depsipeptide. CHEM PHARM BULL 1995 Sep;43(9):1598-600
- Kodama K, Higuchi R, Miyamoto T, Van Soest RW. (-)-Axinyssene: A Novel Cytotoxic Diterpene from a Japanese Marine Sponge Axinyssa sp. ORG LETT 2003 Jan 23;5(2):169-71
- Konig GM, Wright AD. Marine natural products research: current directions and future potential. PLANTA MED 1996 Jun;62(3):193-211
- Koulman A, Proksch P, Ebel R, Beekman AC, van Uden W, Konings AW, Pedersen JA, Pras N, Woerdenbag HJ. Cytotoxicity and mode of action of aeroplysinin-1 and a related dienonefrom the sponge Aplysina aerophoba. JOURNAL OF NATURAL PRODUCTS 1996 Jun;59(6):591-4
- Murakami N, Sugimoto M, Morita M, Kobayashi M. Total synthesis of agosterol A: an MDR-modulator from a marine sponge. CHEMISTRY 2001 Jun 18;7(12):2663-70
- Murakami Y, Takei M, Shindo K, Kitazume C, Tanaka J, Higa T, Fukamachi H. Cyclotheonamide E4 and E5, new potent tryptase inhibitors from an Ircinia species of sponge. JOURNAL OF NATURAL PRODUCTS 2002 Mar;65(3):259-61
- Murakami N, Sugimoto M, Kobayashi M. Participation of the beta-hydroxyketone part for potent cytotoxicity of callystatin A, a spongean polyketide. BIOORG MED CHEM 2001 Jan;9(1):57-67
- Murakami N, Sugimoto M, Nakajima T, Kawanishi M, Tsutsui Y, Kobayashi M. Participation of the conjugated diene part for potent cytotoxicity of callystatin A, a spongean polyketide. BIOORG MED CHEM 2000 Nov;8(11):2651-61
- Pham NB, Butler MS, Hooper JN, Moni RW, Quinn RJ. Isolation of xestosterol esters of brominated acetylenic fatty acids from the marine sponge xestospongia testudinaria. JOURNAL OF NATURAL PRODUCTS 1999 Oct;62(10):1439-42
- Suzuki H, Ueno A, Takei M, Shindo K, Higa T, Fukamachi H. The effects of S1319, a novel marine sponge-derived beta2-adrenoceptor agonist, on IgE- mediated activation of human cultured mast cells. INFLAMMATION RESEARCH 2000 Feb;49(2):86-94
- Suzuki A, Matsunaga K, Shin H, Tabudrav J, Shizuri Y, Ohizumi Y. isprasin, a novel Ca(2+) releaser with caffeine-like properties from a marine sponge, Dysidea spp., acts on Ca(2+)-induced Ca(2+) release channels of skeletal muscle sarcoplasmic reticulum. J PHARMACOL EXP THER 2000 Feb;292(2):725-30
- Tsuda M, Endo T, Watanabe K, Fromont J, Kobayashi J. Nakirodin a, a bromotyrosine alkaloid from a verongid sponge. JOURNAL OF NATURAL PRODUCTS 2002 Nov;65(11):1670-1
- Tsuda M, Endo T, Mikami Y, Fromont J, Kobayashi J. Luffariolides H and J, new sesterterpenes from a marine sponge Luffariella species. JOURNAL OF NATURAL PRODUCTS 2002 Oct;65(10):1507-8
- Tsuda M, Sakuma Y, Kobayashi J. Suberedamines A and B, new bromotyrosine alkaloids from a sponge Suberea species. JOURNAL OF NATURAL PRODUCTS 2001 Jul;64(7):980-2
- Tsukamoto S, Tane K, Ohta T, Matsunaga S, Fusetani N, van Soest RW. Four new bioactive pyrrole-derived alkaloids from the marine sponge Axinella brevistyla. JOURNAL OF NATURAL PRODUCTS 2001 Dec;64(12):1576-8
- Uemoto H, Tsuda M, Kobayashi J. Mukanadins A-C, new bromopyrrole alkaloids from marine sponge agelasnakamurai. JOURNAL OF NATURAL PRODUCTS 1999 Nov;62(11):1581-3
- Umeyama A, Ito S, Yoshigaki A, Arihara S._ Two new 26,27-cyclosterols from the marine sponge Strongylophora corticata._ JOURNAL OF NATURAL PRODUCTS 2000 Nov;63(11):1540-2
- Umeyama A, Adachi K, Ito S, Arihara S. New 24-isopropylcholesterol and 24-isopropenylcholesterol sulfate from the marine sponge Epipolasis species. JOURNAL OF NATURAL PRODUCTS 2000 Aug;63(8):1175-7
- Umeyama A, Ito S, Yuasa E, Arihara S, Yamada T. A new bromopyrrole alkaloid and the optical resolution of the racemate from the marine sponge homaxinella sp. JOURNAL OF NATURAL PRODUCTS 1998 Nov;61(11):1433-4
- Ulubayram K, Aksu E, Gurhan SI, Serbetci K, Hasirci N. Cytotoxicity evaluation of gelatin sponges prepared with different cross-linking agents. JOURNAL OF BIOMATERIAL SCI POLYM ED 2002;13(11):1203-19
- Urban S, de Almeida Leone P, Carroll AR, Fechner GA, Smith J, Hooper JN, Quinn RJ. Axinellamines A-D, Novel Imidazo-Azolo-Imidazole Alkaloids from the Australian Marine Sponge Axinella sp. CHEM 1999 Feb 5;64(3):731-735
- Watanabe D, Tsuda M, Kobayashi J. Three new manzamine congeners from amphimedon sponge. JOURNAL OF NATURAL PRODUCTS 1998 May;61(5):689-92
- Wright AD, Wang H, Gurrath M, Konig GM, Kocak G, Neumann G, Loria P, Foley M, Tilley L. Inhibition of heme detoxification processes underlies the antimalarial activity of terpene isonitrile compounds from marine sponges. J MED CHEM 2001 Mar 15;44(6):873-85
- Wright AD, Konig GM, Angerhofer CK, Greenidge P, Linden A, Desqueyroux-Faundez R. Antimalarial activity: the search for marine-derived natural products with selective antimalarial activity. JOURNAL OF NATURAL PRODUCTS 1996 Jul;59(7):710-6
- Yokokawa F, Asano T, Shioiri T. Total synthesis of the antiviral marine natural product (-)-hennoxazole A. ORG LETT 2000 Dec 28;2(26):4169-72
Ceolenterates
- Fenner PJ; Harrison SL. Irukandji and Chironex fleckeri jellyfish envenomation in tropical Australia. WILDERNESS & ENVIRONMENTAL MEDICINE 2000, Vol 11, Iss 4, pp 233-240
- Keamy J; Umlas J; Lee Y. Red coral keratitis. CORNEA 2000, Vol 19, Iss 6, pp 859-860
- Lindquist N. Tridentatols D-H, nematocyst metabolites and precursors of the activated chemical defense in the marine hydroid Tridentata marginata (Kirchenpauer 1864). JOURNAL OF NATURAL PRODUCTS 2002, Vol 65, Iss 5, pp 681-684
- Mizuno M; Nishikawa K; Yuzawa Y; Kanie T; Mori H; Araki Y; Hotta N; Matsuo S. Acute renal failure after a sea anemone sting. AMERICAN JOURNAL OF KIDNEY DISEASES 2000, Vol 36, Iss 2, art. no. E10
- Radwan FE; Aboul-Dahab HM; Bumett JW. Some toxicological characteristics of three venomous soft corals from the Red Sea. COMPARATIVE BIOCHEMISTRY AND PHYSIOLOGY C-TOXICOLOGY & PHARMACOLOGY 2002, Vol 132, Iss 1, pp 25-35
- Rojas A; Torres M; Rojas JI; Feregrino A; Heimer-da la Cotera EP. Calcium- dependent smooth muscle excitatory effect elicited by the venom of the hydrocoral Millepora complanata. TOXICON 2002, Vol 40, Iss 6, pp 777-785
Molluscs
- Ciminiello P; Dell’Aversano C; Fattorusso E; Forino M; Magno S; Poletti R. The detection and identification of 42,43,44,45,46,47,55-heptanor-41-oxoyessotoxin, a new marine toxin from Adriatic shellfish, by liquid chromatography-mass spectrometry. CHEMICAL RESEARCH IN TOXICOLOGY 2002, Vol 15, Iss 7, pp 979-984
- Gavagnin M, Vardaro RR, Avila C, Cimino G, Ortea J. Ichthyotoxic diterpenoids from the Cantabrian nudibranch Chromodoris luteorosea. JOURNAL OF NATURAL PRODUCTS 1992, Vol 55, Iss 3, pp 368-371
- Kubanek J, Anderson RJ. Evidence for de novo biosynthesis of the polyketide fragment of diaulusterol A by the Northeastern Pacific dorid nudibranch Diaulula sandiegensis. JOURNAL OF NATURAL PRODUCTS, 1999, Vol 62, pp 777-779
- McIntosh JM; Corpuz GO; Layer RT; Garrett JE; Wagstaff JD; Bulaj G; Vyazovkina A; Yoshikami D; Cruz LJ; Olivera BM. Isolation and characterization of a novel Conus peptide with apparent antinociceptive activity. JOURNAL OF BIOLOGICAL CHEMISTRY 2000, Vol 275, Iss 42, pp 32391-32397
- Olivera BM; Cruz LJ. Conotoxins, in retrospect. TOXICON 2001, Vol 39, Iss 1, pp 7-14
- Reel KR, Fuhrman FA. An acetylcholine antagonist from the mucous secretion of the dorid nudibranch, Doriopsilla albopunctata. COMP BIOCHEM PHYSIOL C 1981, Vol 68C, Iss 1, pp 49-53
Annelids/Worms
- Asakawa M; Toyoshima T; Shida Y; Noguchi T; Miyazawa K. Paralytic toxins in a ribbon worm Cephalothrix species (Nemertean) adherent to cultured oysters in Hiroshima Bay, Hiroshima Prefecture, Japan. TOXICON 2000, Vol 38, Iss 6, pp 763-773
- Hwang DF; Tsai YH. Toxins in toxic Taiwanese crabs. FOOD REVIEWS INTERNATIONAL 1999, Vol 15, Iss 2, pp 145-162
- Kem WR. Structure and membrane actions of a marine worm protein cytolysin, cerebratulus toxin-a-111.. TOXICOLOGY 1994, Vol 87, Iss 1-3, pp 189-203
0 Comments