Holosystemics Part III: The Prokaryotes of the Coral Holobiont
by Chris Aslett
Cnidarian-nurturing wild ecosystems have declined 50 percent with further forecast where all classes of reef inhabitant have experienced 63 percent decimation (McCauley et al. 2022). This series of editorials introduces unfamiliar terms and builds, deepens, and consolidates our understanding whilst it contextualizes the micro- and macro-biota of our captive reef holosystem which expedites its life sustaining dynamic equilibrium.
Herein we discover the hidden richness and diversity of coral symbiotic prokaryotes of the kingdoms Bacteria and Archaea which confer immunity and trade beneficial and otherwise unavailable co-factors, trace elements, vitamins, nutrients, and carbon with their host (Kramarsky-Winter et al. 2006; Ainsworth et al. 2015; Munn 2019). Radiation is the newest candidate for an additional prokaryotic phylum (Pollock et al. 2018) while there lies a zone just beneath the surface of the skeleton that is home to endolithic phototrophs (Williams et al. 2015).
Contrasting micro-niches with distinct microclimates suit dissimilar lineages of autotrophic, phototrophic, mixotrophic, and heterotrophic facultative or obligate aerobes and anaerobes which exploit host and symbiont secretions and their metabolic by-products (Pollock et al. 2018).
The findings of several important studies spanning two decades are presented during which analytical techniques advanced significantly, where distinct prokaryotic genera are expressed as phylotypes, ribotypes, operational taxonomic units (OTUs), or amplicon sequence variants (ASVs; McCauley et al. 2022). Several rare unclassified, phylogenetically novel, or uncertain genera were discovered, where many such irregularities are emphasized.
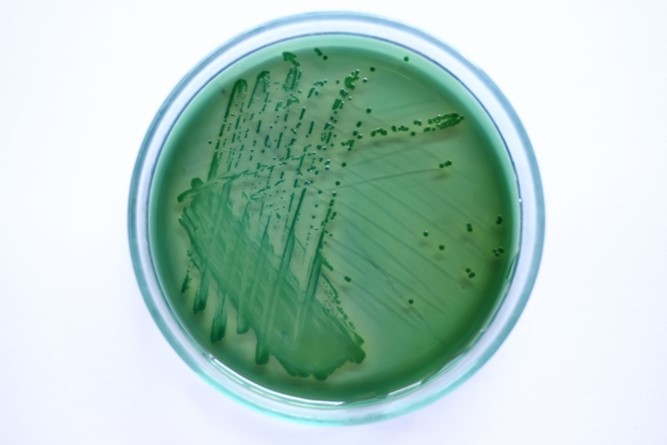
Fig 1. A streak of Vibrio parahaemolyticus cultured on sodium chloride (NaCl) enriched thiosulfate citrate bile salts sucrose agar (TCBS).
Otherwise environmentally rare endosymbiotic OTUs were attuned to colony morphology in one study which is redolent of phenotypic participation (Sunagawa et al. 2010) while the same species of coral can maintain remarkably analogous microbiomes despite significant geographic separation (Williams et al. 2015).
Initial investigations of the bacterial communities of corals utilized agar, broth, microscopy, and substrate exploitation/fermentation to isolate and identify species, however this technology cannot culture most bacteria (Fig 1.; Fuhrman & Campbell 1998) which concealed significant cross-sections of each population. Nevertheless many of the early challenges were surmounted by ribosomal small subunit (16S) nucleic acid (rDNA; rRNA) amplification (PCR) and sequencing, yet there remains a large disparity between the microbial profiles from culture and molecular techniques (Rohwer et al. 2002).
Research has identified several thousand prokaryotic lineages within corals which appear decisive in adaption to an everchanging environment, where the most valuable donations frequently originate from a minority. A coral’s mucosal microbiome is a several millimeters thick external coating of photoautotrophically fixed carbon embedded with microorganisms above an epidermis interspersed with similar communities analogous to a biofilm (Ainsworth et al. 2015).
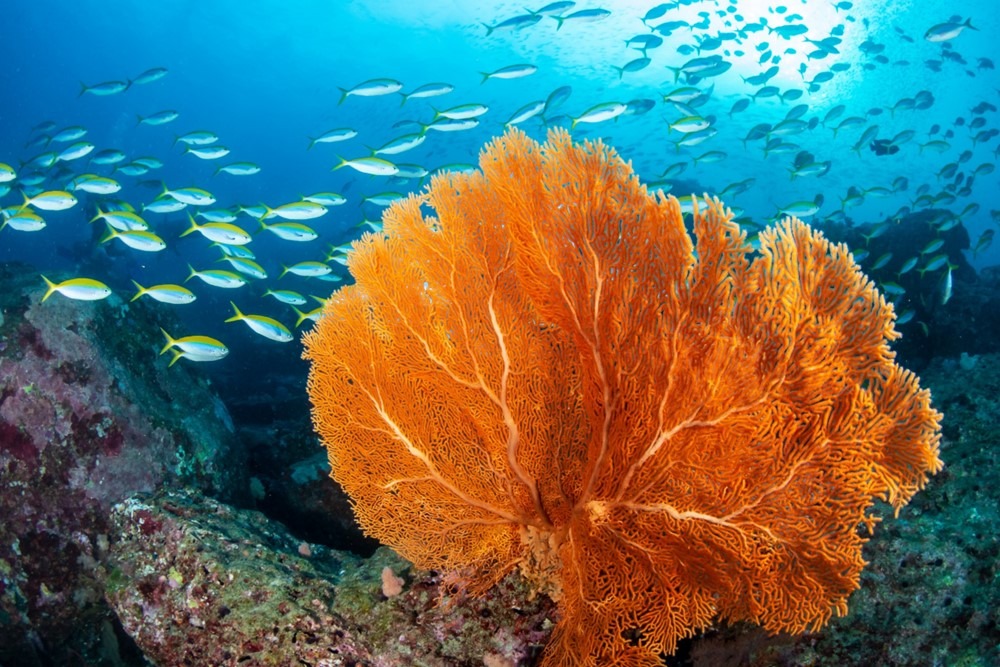
Fig 2. A sea fan of the subclass Octocorallia previously ranked in the orders Alcyonacea or Gorgonacea.
The composition of prokaryotic autotrophy versus heterotrophy versus mixotrophy within coral mucus remains consistent within species but varies interspecifically. Bacterial populations including pathogenic consortia may therefore be shaped by the presence of mucosal inorganic, organic, complex, simple, dissolved, and particulate carbon, amino acids, pyrimidines, glycerol, and phosphorylated compounds (Ritchie & Smith 1995). Photo- and non-photo-synthetic microorganisms dwell in ecto- and endo-dermal-derived tissues co-localized with zooxanthellae (Kramarsky-Winter et al. 2006; Ainsworth et al. 2015; Munn 2019).
Multiple fragments of GBR Acropora granulosa and Hawaiian Montipora capitata and Leptoseris species were collected from shallow to deep water. Prokaryotic (16S) rRNA was amplified from whole colony, symbiotic, and endosymbiotic tissues where the microbial communities were niche-dependent (Ainsworth et al. 2015).
156, 350, and 204 prokaryotic lineages were found in at least 30 percent of samples from A. granulosa, M. capitata, and Leptoseris species respectively, where near-90 percent made up less than 1 percent of each community. 15 and 41 taxa were present in all and merely the symbiotic tissue types of A. granulosa (Ainsworth et al. 2015); therefore symbiotic significance may be inversely related to abundance and proportional to diversity.
Phylogenetically related lineages were compartmentalized in each type of microbiome (Ainsworth et al. 2015) while host intracellular cell-associated microbial aggregates (CAMAs) likely comprise a single species and strain (Boilard et al. 2020).
The classes Burkholderiales and Alphaproteobacteria of the orders Rhizobiales and Caulobacterales were localized with endosymbionts, whereas the orders Endozoicimonaceae, Rickettisales, and Rhodobacterales dominated the mucosal and skeletal communities (Ainsworth et al. 2015).
Seven phylotypes were common core affiliates from geographically and photically diverse ecosystems suggesting they were obligate symbionts. Propionibacterium and Ralstonia species of the classes Actinomycetales and Burkholderiales were widely distributed among Scleractinia alongside zooxanthellae in symbiocytes (Ainsworth et al. 2015). Yet these genera featured as putative environmental contaminants in other studies (Williams et al. 2015).
Multiple kinds of transporter and permease enzymes were prevalent which indicate widespread molecular exchange, which mostly originated from Propionibacterium or Ralstoni species that monopolized amino acid metabolism. Proteins and nucleotides that are raised throughout DNA repair suggested harmful reactive oxygen species (ROS) were liberated from heat- and irradiance-stressed zooxanthellae, whereas biomarkers of nitrogen fixation and metallic ion utilization were symptomatic of diazotrophy and anaerobic endolithic symbiosis (Rohwer et al. 2002; Ainsworth et al. 2015). A recent study discovered that nominal ROS are generated in response to heat which do not leak (Nielsen et al. 2018) while “catalase positive” bacteria manufacture the ROS-disarming and antioxidant enzyme catalase (Colorni & Diamant 2014; Remuzgo-Martínez et al. 2015).
Multiple cores of Panamanian Montastraea franksi were harvested from five reefs between 5 and 10 m depth, where PCR and 16S rDNA sequence analysis detected a community comprising 36 percent of the phylum Cyanobacteria and 27 and 10 percent of the classes Alphaproteobacteria and Gammaproteobacteria. Eukaryotes, anaerobic Clostridium species, and two species of Betaproteobacteria were also present which are extraordinary in seawater. However laboratory culture of similar samples yielded a prokaryotic profile of 83 percent Gammaproteobacteria with a 50/50 mix of Pseudoalteromonas and Vibrio species, yet the remainder comprised Alphaproteobacteria and the genera Clostridium and Cytophaga. 20 and 50 percent and 4 and 25 percent of the phylotypes from culture and molecular analysis were new species and genera respectively (Rohwer et al. 2002). These investigations were thus incongruent and may be unrepresentative, while the differences between planktonic and coral-associated bacteria suggested certain microbes were coral adapted. The alphaproteobacterium Silicibacter lacuscaerulensis was identified in 25 samples from M. franksi taken from five reefs, which are phototrophic diazotrophs. These methodologies remained inadequate for quantification (Rohwer et al. 2002) while the sulphur/nitrogen cycling and fixing repertoires of microorganisms augment photosynthesis and expedite subsistence in the nutrient-poor ecosystems common to pristine wild reefs (Boilard et al. 2020).
Cnidarian lineage, health, life phase, geographic location, and depth influence symbiotic communities. Bacteria of the families Endozoicomonadaceae and Vibrionaceae of the class Gammaproteobacteria are conspicuous core affiliates of cnidarian holobionts. The bacterial phyla Proteobacteria (synonym: Pseudomonadota), Firmicutes (synonym: Bacillota), Bacteroidetes (synonym: Bacteroidota) as well as the Archaea Nanoarchaeota and Crenarchaeota are coral-pervasive which are listed in order of abundance. Around 43 percent of scleractinian-associated bacteria mirror the profile of the surrounding water. The subclass Hexacorallia nurture the greatest diversity and richness compared with Octocorallia where stony corals of the order Scleractinia average 894 ASVs reaching a maximum of 3,794 per sample, whereas Alcyonacea (sea fans) and Antipatharia (black corals) average 427 per sample with a maximum of 2,000 (Fig 2.; Fig 3.; McCauley et al. 2022).
The diversity within alcyonaceans, zoanthids, and scleractinians was greatest in shallow water ecosystems which was reversed in antipatharians. The endolithic microbiome was more diverse in corals with porous skeletons (McCauley et al. 2022) like Fimbriaphyllia or Caulastraea-like species compared to Porites (Fig 4.). Scleractinia exhibit the greatest diversity in their mucosal and skeletal microbiomes which was not observed in other Cnidaria, which suggests these niches are unique to stony corals (McCauley et al. 2022).
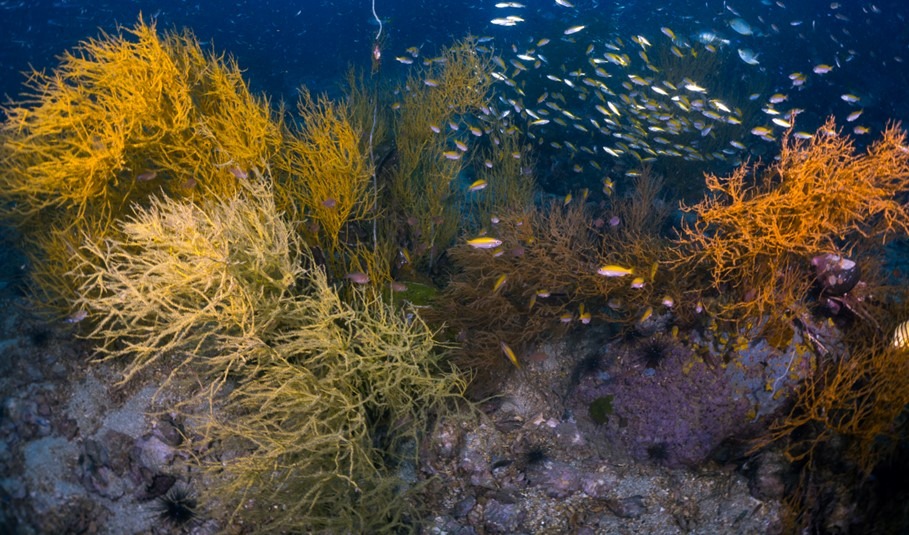
Fig 3. Antipatharian black coral.
Microbial communities are attuned to geographic proximity and host phylogeny where some scleractinian diversity was akin to low microbial abundance sponges, yet the majority were analogues of medium to high. Merely 10 percent of 697,284 ASVs were shared by Cnidarian orders where 71 percent were common to Scleractinia (49,507) and 7.6 percent were found in Alcyonacea (5,299), while the prokaryotic profiles of Cnidaria and seawater are inextricably linked where most ASVs were ocean-specific and stratified with depth. Of the prokaryotic ASVs isolated from cnidarian tissue only 2.6 percent were common to euphotic zone skeletons and mucus (1,812). Scleractinia and Alcyonacea retain two thirds of the ASVs shared between cnidarian families (46,927), where 36.5 percent were hosted by Scleractinia (17,128) which constituted merely ~2.6 percent of their microbial community, whereas Alcyonacea nurtured 31.7 percent (14,876) that formed 17.5 percent of their microbes. ASV inter-order commonality was highest in alcyonaceans, whereas intra-order sharing was greatest in Scleractinia. 86.1, 6.7, and 5.4 percent of scleractinian-constrained cnidarian ASVs were only found in tissue (14,747), mucus (1,147), and skeleton (924), while anatomical proximity influenced population commonality (Pollock et al. 2018; McCauley et al. 2022). Only 2.4 and 1.2 percent of scleractinian ASVs were shared between tissue and mucus (411) or tissue and skeleton (205), while merely 0.2 percent were found in skeleton and mucus (34) with a colony-pervasive 0.1 percent (17). Hexacorallian and octocorallian endolithic ASVs were 10 to 20 times less likely to occur elsewhere compared to bacteria from tissue and mucus (McCauley et al. 2022).
Prokaryotic richness was order and depth dependent where deeper Cnidaria nurtured more unique communities compared with those from shallow water where microbiomes were conventionally replete. Although the symbiotic fitness of the cnidarians in the ecosystem influenced the proportions of tissue-bound symbionts, the impact was unapparent in discrete holobionts. Euphotic Scleractinia host more ASVs than other localized cnidarians, where less than 10 ASVs were collective core affiliates of corals albeit ~60 were mutual to anemones including “glass” (Exaiptasia pallida; McCauley et al. 2022).
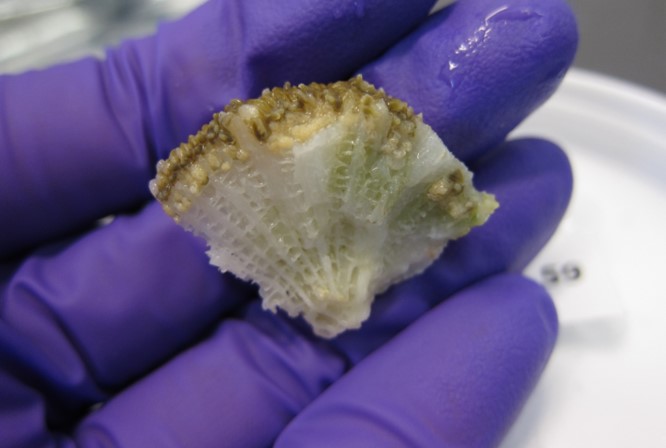
Fig 4. A small section of pillar coral (Dendrogyra cylindrus) featuring its fragile honeycomb-like skeleton and immature oocytes (eggs). Image courtesy of The Florida Fish and Wildlife Commission©.
Alteromonas, Propionibacterium, Pseudoalteromonas, Pseudomonas, and Vibrio species were shared among cnidarian orders irrespective of depth, whereas Candidatus Pelagibacter, Pelomonas, and Sphingomonas were common in the euphotic zone. Data analysis affirmed species of Alteromonas, Propionibacterium, and Pseudoalteromonas as cnidarian core affiliates, where Endozoicomonas species of the family Hahellaceae occur cross-anatomy and Candidatus, GpIIa, Pelagibacter, Pseudoalteromonas, and Vibrio species are frequent recruits of mucus and tissue (McCauley et al. 2022).
15 prominent clades of Endozoicomonas species may be evident which are not restricted to Cnidaria, yet they are present in the anthozoan orders Actinaria, Alcyonacea, Antipatharia, Corallimorpharia, Helioporacea, Rhizostomeae, Zoantharia, and Scleractinia including azooxanthellates. 74.3 and 15.8 percent of the 4,138 Endozoicomonas library ASVs occur in Scleractinia (3,074) and Alcyonacea (654), where 88.5, 5.5, 2.1, and 3.9 percent of scleractinian ASVs were found exclusively in tissue (2,720), mucus (169), skeleton (65), and whole anatomy (120). Clade 2 Endozoicomonas are unique to Acroporidae from the Indian Ocean; clade 13 inhabit Pocilloporidae in the Pacific, and clade 14 dwell in Rhizostomeae in the Atlantic, whereas clade 11 is a globally distributed generalist that favours Octocorallia (McCauley et al. 2022). Pollock and collaborators (2018) identified two clades of Endozoicomonas-like bacteria. The species and strains of the first (HS) exhibited extraordinary host fidelity which were close relatives of those known to infect gorgonians, molluscs, sponges, and other invertebrates which substantiates co-diversification and hence co-phylogeny, whereas the second clade was cosmopolitan and pervasively hosted which was designated clade HG (Pollock et al. 2018).
The phylogenetic relationship between the host and its symbionts proposes the paradigm of phylosymbiosis where phylogeny, geography, and the environment structure microbial assemblages, while prokaryotic endosymbionts common to most Cnidaria and organisms that share related lifestyles posit co-phylogeny where the host evolves with rare subpopulations like clades of Endozoicomonas (Pollock et al. 2018).
236 fragments from 32 Scleractinia and four octocorallian sea fans were sampled from GBR Australian reefs 17o south of the equator, whereupon a further 162 fragments were collected from Lizard Island in summer and winter. 10 percent of ASVs remain undetected by PCR and 16S rRNA sequence analysis, while mitochondrial nucleic acid was used to classify coral genera. 69 prokaryotic phyla were discerned from Scleractinia, of which 56.5 percent were Proteobacteria (39) and 2 percent were Archaea (1). Candidatus Amoebophilus is an intracellular symbiont of eukaryotes that was found in over 50 percent of tissue microbiomes, while mucosal communities exhibited strong intrahost commonality, stability, and richness with a plastic minority. Host species was the single most determining factor where nominal cross-anatomy affiliates were shared, while populations were routinely environmentally and interspecifically diverged (Pollock et al. 2018).
Multivariate machine learning forecasts microbial communities with over 74 percent accuracy, where host traits and ancestral lineage determine endosymbionts and skeletal populations whilst the mucosal microbiome remains environmentally attuned. Furthermore mucus and tissues become less crowded at greater latitudes which causes minor expansions of diversity (Pollock et al. 2018).
Host maturity impacts population dynamics where larger colonies hosted less Aurantimonas and Balneola species associated with white plague type II (Fig 5.; Denner et al. 2003) and Red Sea sewage discharge, which was accompanied by a loss of diversity (Ziegler et al. 2016). Juvenile colonies and “frags” may be thus more disease-susceptible but this trend likely represents age-dependent stability (Pollock et al. 2018).
Hosts and prokaryotes are phylosymbiotically aligned inasmuch as related corals nurture comparable populations, which is more noticeable in endolithic communities. Endosymbiotic and skeletal microbiomes became proportionally dissimilar as coral lineages diverged, while mucosal microbiomes were restructured to a threshold after which they stabilized (Pollock et al. 2018). Cross-anatomy prokaryotic richness was comparable in host sister taxa which became disproportionately dissimilar as phylogenetic distance increased, however balance was restored beyond familiar separation. These findings suggest a significant alteration in microbiome density coincided with the “modern” radiation of scleractinian families some twenty-five to sixty-five million years ago (LaJeunesse et al. 2018; Pollock et al. 2018). Phylosymbiosis is thus upheld insofar as the evolution of cnidarian hosts has shaped and constrained the composition and richness of their endosymbiotic and endolithic communities. Very few prokaryotes and corals have co-diversified which validates co-phylogeny, however their recently established host-specific mucosal microbiomes were not structured on an equivalent evolutionary timescale. Nevertheless their skeletal populations are extraordinarily enriched with the clearest phylosymbiotic signature (Pollock et al. 2018).
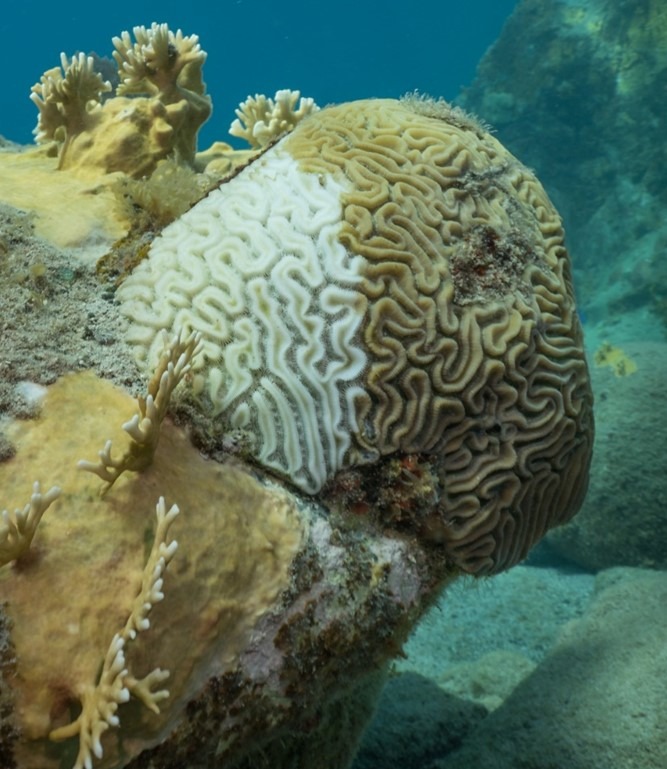
Fig 5. cf. white plague of Pseudodiploria strigosa, St. Thomas, the US Virgin Islands. Photograph by Dan Mele©.
Acropora-like mitochondrial lineages (mitotypes) host a comparatively dense microbial community because the genera of tissue-bound endosymbionts are 59 percent more aligned with host mitotype compared with phylotype. The prokaryotic families Clostridiaceae, Myxococcales, and Kiloniellales were restricted to certain mitotypic hosts, although Candidatus Amoebophilus is a common endolithic core affiliate of the genera Seriatopora and Stylophora which share a clade and is thus phylotypically constrained (Fig 6.). 76 percent of coral mitotypes sustain enduring associations with clades of bacteria, while merely a subpopulation of prokaryotic genera exhibit meaningful host specificity. Bacteria of the families Clostridiaceae, Endozoicomonaceae, Kiloniellales, and Myxococcales routinely infect certain phylotypes of coral (Pollock et al. 2018).
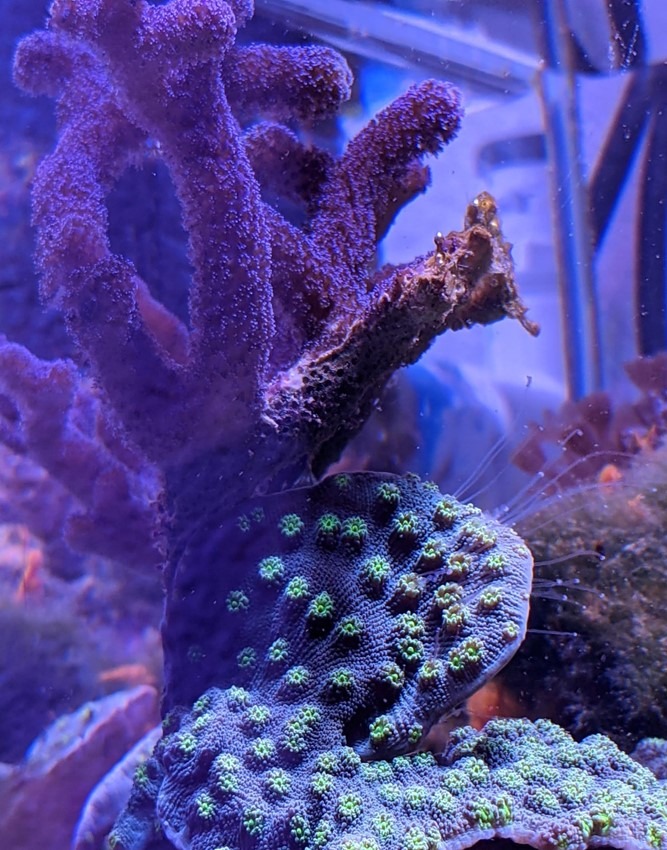
Fig 6. The sweeper tentacles of chalice coral Echinopora lamellosa (Faviina: Merulinidae) have destroyed the tissue of adjacent Stylophora pistillata (Astrocoeniina: Pocilloporidae). Image courtesy of Jason Hughes©, Austin, Texas, USA.
An age-related study analyzed the prokaryotic microbiomes of juvenile (2.8 cm; 1 year), small (6 cm; 2 to 3 years), medium (9.9 cm; 4 years), large microatolls (28 cm; 9 to 10 years) and large entire colonies (32 cm; 10 to 12 years) of Coelastrea aspera of the family Merulinidae from Phuket Island, Thailand. Mucosal and endosymbiotic microbiomes and cores of skeleton with and without discernible endolithic algae were taken for microbial analysis from juvenile and large entire colonies from a tidal flow channel, while others were from a surge-insulated but heat-exposed reef flat table (Williams et al. 2015).
Lower than anticipated cross-anatomy and cross-size richness was pronounced in juvenile and small colonies which may be peculiar to corals in exposed areas of a reef. Significant microbial diversity was influenced by colony age, insofar as it was slightly curtailed in older while the communities of younger and their replicates varied considerably. Corynebacterium, Lentisphaerae, Meiothermus, Propionibacterium, Pseudovibrio, Rhodobacteraceae (unclassified Paracoccaceae), and Tenericutes species (unclassified Mycoplasmatota) were amongst those of small and juvenile C. aspera but Nitrospirae (unclassified Nitrospirota) and Ralstonia species were predominant in small colony populations yet were absent in other sizes. Vibrio, Streptococcus, Pseudomonas, Kiloniellales (unclassified), Halomicronema, Terriglobia (synonym: Acidobacteria), and invalid Verrucomicrobia species were conspicuous in larger colonies while only the former three were found in other sizes (Williams et al. 2015).
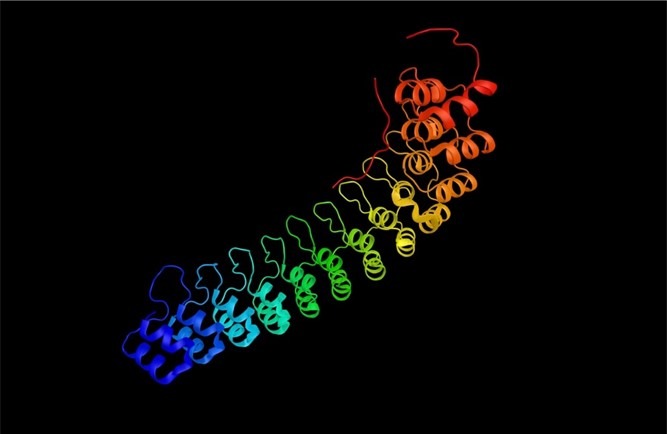
Fig 7. Ankyrin repeats (vANK) are polymers of two helices separated by a loop.
Tenericutes and Spirochaetes-like ribotypes were detected in mucus but not tissue, where the former were found exclusively in younger and the latter in older colonies. Almost twice as much prokaryotic richness was found in tissues compared to skeleton yet some ribotypes were shared such as Endozoicomonas, Corynebacterium, Bacteroidia (synonym: Bacteroidetes), Granulicatella, Pseudovibrio, Halomicronema, Rhodobacteraceae, and Oscillospira species. The bacterial communities of endolithic algal bands were dominated by Corynebacterium, Bacteroidetes, Cyanobacterium, Pseudovibrio, Halomicronema, and Oscillospira species where a minority were shared amongst skeleton and tissue (Williams et al. 2015).
Scanning electron micrographs (SEMs) of skeleton revealed cf. Bacteroidetes and Pseudovibrio species, whilst aggregates of cf. Halomicronema species of boring Cyanobacteria were observed in the algal band where some had invaded tissue. Histology revealed contagion between symbiotic compartments in medium colonies where Halomicronema and Oscillospira species were pervasive in tissue and endolithic algal bands, which may explicate skeletal recruitment and translocation of photosynthate from phototrophic endolithic populations during postbleaching recouperation (Williams et al. 2015).
Corynebacterium, Granulicatella, Pseudovibrio, and Propionibacterium species remain core affiliates throughout coral development, while Meiothermus, Tenericutes and Lentisphaerae species wane. The genera Tenericutes, Lentisphaerae, Ralstonia and Nitrospirae are merely present in juvenile and small colonies. Species of Rhodobacteraceae are conspicuously prominent in juvenile and small, nominally represented in medium, and absent from microatoll and large specimens. Other studies have noted bacteria such as these of the clade Roseobacter were acquired early in coral development, which include coral pathogens and antimicrobial-competent phototrophic symbionts (Biebl et al. 2005; Garren et al. 2009; Nissimov et al. 2009; Mouchka et al. 2010).
Roseobacter and Pseudoalteromonas species inoculated onto the mucus of diseased corals, attenuated the growth of two known pathogenic species of Vibrio (Nissimov et al. 2009) whereas diseased corals retain higher proportions of the genera Acidobacteria, Clostridia, Cyanobacteria, Rhodobacter, and Vibrio (Mouchka et al. 2010). The composition of microbial symbionts appears to vary throughout the ageing process (Williams et al. 2015) whereas defined classes of prokaryotes are liberated during coral spawns and within gamete mucus which may assist their recruitment (Thompson et al. 2015; Boilard et al. 2020).
The orders Alpha- and Gamma-proteobacteria of the phylum Proteobacteria and the phyla Bacteroidetes, Cyanobacteria, Firmicutes, and the genera Endozoicomonas, Vibrio, and Serratia are widespread common core affiliates of Scleractinia.
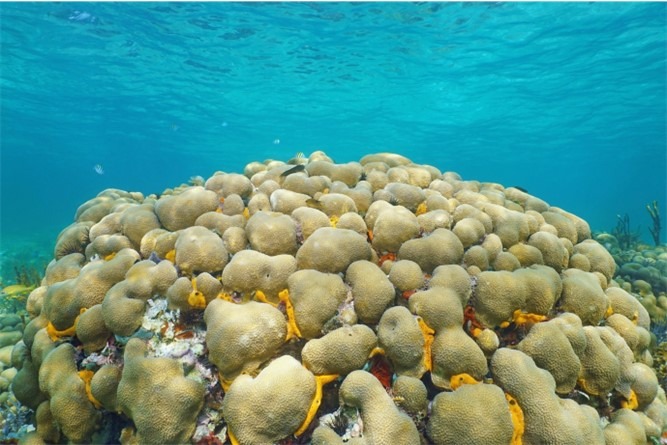
Fig 8. A giant (massive) colony of Porites porites providing an ecological niche where holobiont affiliates include sponges, tubeworms, anemones, urchins, polychaetes, and corallivorous snails.
Symbiotic bacteria modulate the density, growth, and nutrition of Symbiodiniaceae, forestall pathogenic invasion, and mitigate stress. The prokaryotic phyla of Porites lutea carry genes encoding ankyrin repeats which are multiple arrays of two helices separated by a loop that are associated with symbiotic stability in corals and immune modulation in fish (Fig 7.; Fig 8.; Guo et al. 2011; Boilard et al. 2019).
Prokaryotic symbionts can complete the nitrogen cycle and thus donate ammonia (NH3), ammonium (NH4+), nitrite (NO2–), nitrate (NO3–), biocidal nitric oxide (NO), nitrous oxide (N2O), Cyanobacteria-nourishing dissolved dinitrogen (DN2), organic nitrogen (Boilard et al. 2020), and hydrazine (N2H4) from anaerobic ammonia oxidation (anammox) occurring in endolithic Archaea.
Loss of Symbiodiniaceae is accompanied by a holobiont dearth of dissolved inorganic nitrogen (DIN), whereas diazotrophy generates DIN from dissolved dinitrogen (DN2) which promotes photosynthetic efficacy, zooxanthellae abundance, and coral health. The Archaea Euryarchaeota and Thaumarcheota are abundant in corals where the latter are conspicuous nitrogen cyclers. Endozoicomonas species are versatile exploiters of organic substrates that can transform the osmoregulating substance dimethylsulphoniopropionate (DMSP) of Symbiodiniaceae to dimethylsulphide (DMS) which proves vital for the detoxification of accumulated and localized metabolic by-products. Competent barrier-sustaining mucosal microbiomes comprise bacterial exopolymers and compounds of carbohydrates and fats (glycolipids). Many commensal bacteria gain nutrition by digesting mucus using glycosidase enzymes yet they swarm to outcompete invading pathogens. Bacteria in symbiotic tissues produce a repertoire of biocides that remarkably comprise fungicides (Boilard et al. 2020) and communicate using quorum sensing autoinducers that expedite a concerted response to their environment, albeit they quench these signals from invading pathogens (Tait et al. 2010). Endozoicomonas species decline, virulence determinants from pathogenic vibrios increase, and diversity is amplified before bleaching (Boilard et al. 2020; Xu et al. 2023). Notwithstanding contrasting studies acknowledge a loss of diversity precedes dysbiosis and disease (Pollock et al. 2019).
Hermatypic prokaryotes are integral to the holobiont metabolic network which supports photosynthesis and the stability of eubiosis in the face of anomalous temperatures. However severe perturbation of population dynamics interrupts the exchange between the coral and its Symbiodiniaceae which inevitably leads to photosynthetic shutdown and dysbiosis, which frequently develops into disease and mortality. These variations occur a significant time before bleaching and thus their elucidation and profiling is key to monitoring wild populations and the development of manipulative inoculums that restore function and health. Elevated temperatures cause cellular trauma which initiates necrosis in all holobiont affiliates, while the coral’s capacity to recover depends on cellular repair, and the multiplication of residual as well as the recruitment of new zooxanthellae and microbes (Preston 2015; Boilard et al. 2020).
Microbial community shifts may be adaptive whereby unfavourable conditions are sensed and the population responds to conserve homeostasis, and/or adapts to favor recruitment of resilient microbes and zooxanthellae (Boilard et al. 2020).
Next time we discover how the prokaryotic communities of reef-building corals confer resilience to environmental change in part IV.
References
Adams, L., M., Cumbo, V., R. & Takabayashi, M. (2009) Exposure to sediment enhances primary acquisition of Symbiodinium by asymbiotic coral larvae. Marine Ecology Progress Series. 377, 149-156.
Ainsworth, D., T., Krause, L., Bridge, T., Torda, G., Raina, J., Zakrzewski, M., Gates, R., Padilla-Gamiño, J., Spalding, H., Smith, C., Woolsey, E., Bourne, D., Bongaerts, P., Hoegh-Guldberg, O. & Leggat, W. (2015) The coral core microbiome identifies rare bacterial taxa as ubiquitous endosymbionts. The ISME Journal. 9(10), 2261-2274.
Andreoni, F. & Magnani, M. (2014) Photobacteriosis: Prevention and Diagnosis. Journal of immunology research. 2014,.
Balling, H., W., Janse, M. & Sondervan, P. (2008) Trace elements, functions, sinks and replenishment in reef aquaria. Advances in coral husbandry in public aquariums. Leewis, R., J. & Janse, M. (eds.). Burgers’ Zoo, Arnhem, The Netherlands. pp 143-156.
Berger, M. The University of Leeds, UK.
Biebl, H., Allgaier, M., Tindall, B., J., Koblizek, M., Lunsdorf, H. & Pukall, R. (2005) Dinoroseobactershibae gen. nov., sp. nov., a new aerobic phototrophic bacterium isolated from dinoflagellates. Int J Syst Evol Microbiol. 55, 1089-1096.
Boilard, A., Dubé, C., E., Gruet, C., Mercière, A., Hernandez-Agreda, A. & Derome, N. (2020) Defining Coral Bleaching as a Microbial Dysbiosis within the Coral Holobiont. Microorganisms. 8, 1682. doi:10.3390/microorganisms8111682
Cerenius, L., Kawabata, S., Lee, B., L., Nonaka, M. & Soderhall, K. (2010) Proteolytic cascades and their involvement in invertebrate immunity. Trends Biochem. Sci. 35, 575-583. doi:10.1016/j.tibs. 2010.04.006
Cunning, R. & Baker, A., C. (2013) Excess algal symbionts increase the susceptibility of reef corals to bleaching. Nature Climate Change. 3, 259-262.
evolution, development, and ecological interactions. Frontiers in Cellular and Infection Microbiology. 4,.
Fuhrman, J., A. & Campbell, L. (1998) Microbial microdiversity. Nature. 393, 410-411.
Fujise, L., Yamashita, HY., Suzuki, G., Sasaki, K., Liao, L., M. & Koike, K. (2014) Moderate Thermal Stress Causes Active and Immediate Expulsion of Photosynthetically Damage Zooxanthellae (Symbiodinium) from Corals. PLOS ONE. DOI:10.1371/journal.pone.0114321
Garren, M., Raymundo, L., Guest, J., Harvell, C., D. & Azam, F. (2009) Resilience of coral¬ associated bacterial communities exposed to fish farm effluent. PLoS One. 4, e7319. doi: 10.1371/journal.pone.0007319
Gates, R., D., Baghdasarian, G. & Muscatine, L. (1992) Temperature stress causes host cell detachment in symbiotic cnidarians: implications for coral bleach-ing. Biol Bull. 182, 324–332. https://doi.org/10.2307/1542252
Guo, C., Chen, W., Yuan, L., Yang, L., Weng, S., Yu, X. & He, J. (2011) The viral ankyrin repeat protein (ORF124L) from infectious spleen and kidney necrosis virus attenuates nuclear factor-κB activation and interacts with IκB kinase β. The Journal of general virology. 92(7), 1561-1570.
Hays, G., C. (2004) A review of the adaptive significance and ecosystem consequences of zooplankton diel vertical migrations. Hydrobiologia. 503, 163-170.
Hylkema, A. (2014) Decreased growth of Stylophora pistillata with nutrient-driven elevated zooxanthellae density is largely explained by DIC limitation. Reefs.com. https://reefs.com/magazine/decreased-growth-of-stylophora-pistillata-with-nutrient-driven-elevated-zooxanthellae-density-is-largely-explained-by-dic-limitation/
McCauley, M., Goulet, T., L., Jackson, C., R. & Loesgen, S. (2022) Systematic review of cnidarian microbiomes reveals insights into the structure, specificity, and fidelity of marine associations. Nature Communications. 14, 4899. https://doi.org/10.1038/s41467-023-39876-6
Mouchka, M., E., Hewson, I. & Harvell, C., D. (2010) Coral¬associated bacterial assemblages: current knowledge and the potential for climate-driven impacts. Integr. Comp. Biol. 50, 662-674. doi: 10.1093/icb/icq061. pmid:21558231
Nielsen, D., A., Petrou, K. & Gates, R., D. (2018) Coral bleaching from a single cell perspective The ISME Journal. 12, 1558–1567. https://doi.org/10.1038/s41396-018-0080-6
Nissimov, J., Rosenberg, E. & Munn, C., B. (2009) Antimicrobial properties of resident coral mucus bacteria of Oculina patagonica. FEMS Microbiol Lett. 292, 210-215. doi: 10.1111/j.1574¬6968.2009.01490.x.
Pollock, F., J., Lamb, J., B., van de Water, J., Smith, H., A., Schaffelke, B., Willis, B., L. & Bourne, D., G. (2019) Reduced diversity and stability of coral-associated bacterial communities and suppressed immune function precedes disease onset in corals. R Soc Open Sci. 6, 190355. https://doi.org/10.1098/rsos .190355
Pollock, F.,J., McMinds, R., Smith, S., Bourne, D., G., Willis, B., L., Medina, M., Thurber, R., V. & Zaneveld, J., R. (2018) Coral-associated bacteria demonstrate phylosymbiosis and cophylogeny. Nat Commun. 9, 4921.
Preston, E. (2015) Deadly “White Syndrome” Kills Coral, and it’s Spreading. Hakai Magazine, Costal Science and Societies. https://www.hakaimagazine.com/news/deadly-white-syndrome-kills-coral-and-its-spreading/
Ritchie, K., B. & Smith, G., W. (1995) Preferential carbon utilization by surface bacterial communities from water mass, normal, and white-band diseased Acropora cervicornis. Mol Mar Biol Biotechnol. 4, 345-354.
Rodriguez-Lanetty, M., Scaramuzzi, C., Quinnell, R. & Larkum, A. (2005) Transport of symbiotic zooxanthellae in mesogleal canals of Zoanthus robustus? Coral Reefs. 24, 195-196.
Rohwer, F., L., Seguritan, V., Azam, F. & Knowlton, N. (2002) Diversity and distribution of coral-associated bacteria. Mar Ecol Prog Ser. 243, 1–10.
Rosado, P., M., Leite, D., C., Duarte, G., A., Chaloub, R., M., Jospin, G., da Rocha, U., N., Saraiva, J., P., Dini-Andreote, F., Eisen, J., A. & Bourne, D., G. (2019) Marine probiotics: Increasing coral resistance to bleaching through microbiome manipulation. ISME J. 13, 921.
Santoro, E., P., Borges, R., M., Espinoza, J., L., Freire, M., Messias, C., S., M., A., Villela, H., D., M., Pereira, L., M., Vilela, C., L., S., Rosado, J., G., Cardoso, P., M., Rosado, P., M., Assis, J., M., Duarte, G., A., S., Perna, G., Rosado, A., S., Macrae, A., Dupont, C., L., Nelson, K., E., Sweet, M., J., Voolstra, C., R. & Peixoto, R., S. (2021) Coral microbiome manipulation elicits metabolic and genetic restructuring to mitigate heat stress and evade mortality. Science advances. 7(33), 3088.
Sekar, R., Kaczmarsky, L., T., Richardson, L., L. & Chardson, L., L. (2008) Microbial community composition of black band disease on the coral host Siderastrea siderea from three regions of the wider Caribbean. Mar Ecol Prog Ser. 362, 85-98.
Sharp, K., H., Distel, D. & Paul, V., J. (2011) Diversity and dynamics of bacterial communities in early life stages of the Caribbean coral Porites astreoides. The Isme Journal. 6, 790.
Slavov, C., Schrameyer, V., Reus, M., Ralph, P., J., Hill, R., Büchel, C., Larkum, A., W., D. & Holzwarth, A., R. (2016) “Super-quenching” state protects Symbiodinium from thermal stress — Implications for coral bleaching. Biochimica et Biophysica Acta. 1857, 840–847.
Smith, H., A., Fulton, S., E., McLeod. I., M. & Bourne, D., G. (2023) Sea-weeding: Manual removal of macroalgae facilitates rapid coral recovery. Journal of Applied Ecology. 00, 1-13. DOI: 10.1111/1365-2664.14502
Sunagawa, S., Woodley, C. M. & Medina, M. (2010) Threatened corals provide underexplored microbial habitats. PLoS ONE. 5, e9554.
Tait, K., Hutchison, Z., Thompson, F., L. & Munn, C., B. (2010) Quorum sensing signal production and inhibition by coral‐associated vibrios. Environmental Microbiology Reports. 2, 145-150.
Williams, A., D., Brown, B., E., Putchim, L. & Sweet, M., J. (2015) Age-related shifts in bacterial diversity in a reef coral. PLoS ONE. 10, 1–16.
Xu, M., Cheng, K., Xiao, B., Tong, M., Cai, Z., Jong, M-C., Chen, G. & Zhou, J. (2023) Bacterial Communities Vary from Different Scleractinian Coral Species and between Bleached and Non-Bleached Corals. Microbiology Spe ctrum. 11(3),.
Ziegler, M. et al. (2016) Coral microbial community dynamics in response to anthropogenic impacts near a major city in the central Red Sea. Mar. Pollut. Bull. 105, 629–640.
0 Comments