By Kenneth Wingerter
Of all of the flora and fauna that can be displayed in aquaria, fish have always, by far, received the most attention. Arguably, the lasting popularity of ornamental fish owes mainly to their often vibrant coloration. Seemingly few people who work with these animals—even fish biologists and professional aquarists—ever lose this special appreciation of them as “objects” of beauty.
That being said, much useful information can be gained from observing color characteristics in captive fish. Changes in their hues or intensity can serve as an indication of health, signal a readiness to spawn or even reveal how they are “feeling.” Consequently, a basic understanding of the processes through which fish coloration is produced and controlled can be of great value to any keeper.
This article, the second of a two-part series, examines the mechanisms through which colors are produced by fish, as well as ways that aquarists can manipulate diet and environment to maintain rich, natural coloration in captive specimens. The preceding article discussed the roles that fish coloration play in various survival strategies.
Sources of Fish Coloration
Of all vertebrates, fish have the greatest number of color producing cells. The countless colors and patterns expressed by fishes are principally determined through genetic mechanisms. It is therefore quite understandable why ichthyologists continue to use color dress to distinguish smaller taxonomic categories such as species and subspecies (Lagler et al, 1962; Malek, 2008).

One plausible reason for the seeming prevalence of red-orange animal coloration at higher latitudes is the great abundance of carotenoid yielding plants that grow there. Photo by Felicia McCaulley.
Usually, fish coloration is derived from a number of sources. A background coloration (or complexion) comes from underlying body tissues, bodily fluids and, sometimes, even gut content. Overlying hues are produced by specialized color cells called chromatophores or (less often) by bioluminescent structures called photophores (Lagler et al, 1962).
Chromatophores produce either structural or pigmentary coloration (Malek, 2008). Structural coloration is generated by the selective reflection of light of specific wavelengths. In fishes, this generally produces metallic hues, often with iridescence. Light reflected from multiple layers of transparent film results in iridescent colors that change with changes in the animal’s orientation to a light source (Hickman et al, 1995).

Early home aquarists were drawn to fish keeping by the vibrant hues of fish such as the common guppy (Poecilia reticulata).
Reflective crystal structures (or schemochromes) are primarily responsible for structural colors. The brilliant, iridescent blue hues of the male kyusen wrasse (Haliochoeres poecilopterus) are created by the laminar structure of its schemochromes (Hoar and Randall, 1969).
One well known function of structural coloration produced by schemochromes is that of concealment. Light is reflected from the bodies of these animals at an intensity that is equal to that of the background illumination. With skin that has variable reflective properties, these fishes appear silvery blue from the sides while appearing darker from above (Hoar and Randall, 1969).
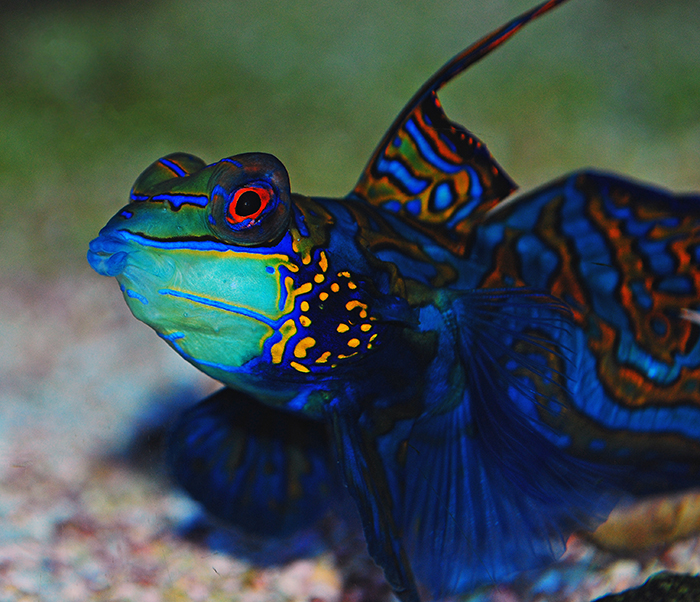
Highly unusual blue pigments are responsible for much of the striking coloration of the mandarin fish (Synchiropus splendidus). Photo by Felicia McCaulley.
Pigmentary coloration is the most common source of color in fishes. It is generated by the absorption of light of specific wavelengths by integumentary pigments. The true pigments (or, biochromes) form a highly variable molecular group. Biochromes are rather adaptable, being responsive to signals brought about by certain stimuli in the animal’s environment. They might condense and concentrate at the center of the chromatophore (resulting in subdued coloration) or they might be distributed widely across the cell (resulting in intensified coloration) (Hickman et al, 1995). In fishes, unlike in birds and mammals, pigments travel discreetly throughout the cell along microtubules (Malek, 2008).
Workings of the Chromatophores
Different hues are produced by different types of chromatophores. Fish chromatophores are categorized by the type of pigments they contain. Seven distinct sorts of chromatophore can be found in fish— iridophores, leucophores, melanophores, xanthophores, erythrophores, cyanophores anderythro-iridophores (Hickman et al, 1995; Goda et al, 2013).
Iridophores contain schemochromes. Iridophore schemochromes are comprised of various purines (often guanine). These crystals are often quite large and are usually arranged in rigid, intricate configurations to produce light interference effects (Hickman et al, 1995).

The complex arrangement of chromatophores of fish such as the scrawled filefish (Aluterus scriptus) is under tight genetic control. Photo by Felicia McCaulley.
Leucophores also contain schemochromes. However, unlike those in iridiphores, the guanine crystals in leucophores are small, comparatively simple granules that are usually capable of moving freely throughout the cytoplasm to produce light scattering effects. The result is white coloration (Hoar and Randall, 1969).
Melanophores contain brown and black biochromes. Melanophore biochromes are comprised of melanin, an oxidized and polymerized derivative of tyrosine. Melanin is believed to be the most ancient and prevalent of all pigments. Though they are concentrated in the melanophores, melanins can be found in various parts of the bodies of vertebrates where they may perform a variety of functions (Hickman et al, 1995; Hoar and Randall, 1969).

Bright, unusual hues and a scarcity in the trade ensures a premium price for species such as blotched anthias (Holanthias borbonius). Photo by Felicia McCaulley.
Erythrophores contain mainly red biochromes. Erythrophore biochromes are usually rich in fat soluble pigments called carotenoids. One such group of pigments is the carotenoids, which are synthesized by plants. The carotenoids may be divided into two major groups: the xanthophylls, which contain oxygen, and the carotenes, which do not contain oxygen. One such group of pigments is the carotenoids, which are synthesized by plants. The carotenoids may be divided into two major groups: the xanthophylls, which contain oxygen, and the carotenes, which do not contain oxygen. Carotenes are most commonly found in erythrophores, whereas xanthopyhlls are most commonly found in xanthphores (Hemdal, 2006; Hickman et al, 1995; Hoar and Randall, 1969).

The metallic radiance of the wrought iron butterflyfish (Chaetodon daedalma) is produced by a distinctive interplay of melanophores and iridiphores. Photo by Felicia McCaulley.
Xanthophores contain mainly yellow biochromes. In addition to carotenoids, xanthophore biochromes contain water soluble pigments called pteridines. Pteridines may be red (e.g. drosopterins), yellow (e.g. sepiopterins) or colorless precursors of other pigments (e.g. leucopterins). Pteridines and/or carotenoid pigments are contained in structures called pterinosomes (or xanthosomes). Both xanthophores and erythrophores contain pigment granules that are believed to be storage vesicles for carotenoid compounds. Because carotenoids are fat soluble and are insoluble in water, the term “lipophore” is often used in reference to both xanthophores and erythrophores (Sloman et al, Hoar and Randall, 1969).
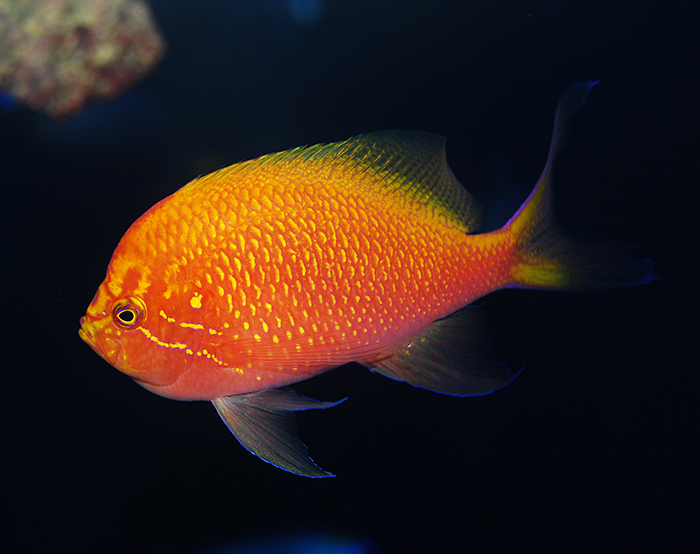
The fathead anthias (Serranocirrhitus latus) nearly glows under modern aquarium lighting. Photo by Felicia McCaulley.
Cyanophores contain blue biochromes. The chemical composition of these novel pigments is still being investigated, as they have only recently been discovered. In point of fact, cyanophores have thus far been found to exist in the integument of only two closely related species, the mandarin fish (Synchiropus splendidus) and the psychedelic fish (S. picturatus) (Goda et al, 2013).
Erythro-iridophores contain a combination of schemochromes and biochromes that both reflect and absorb light to generate reddish-violet hues. This novel dichromatic chromatophore was first observed during an investigation of the pigmentaion of the purple stripe dottyback (Pseudochromis diadema) (Goda et al, 2011).
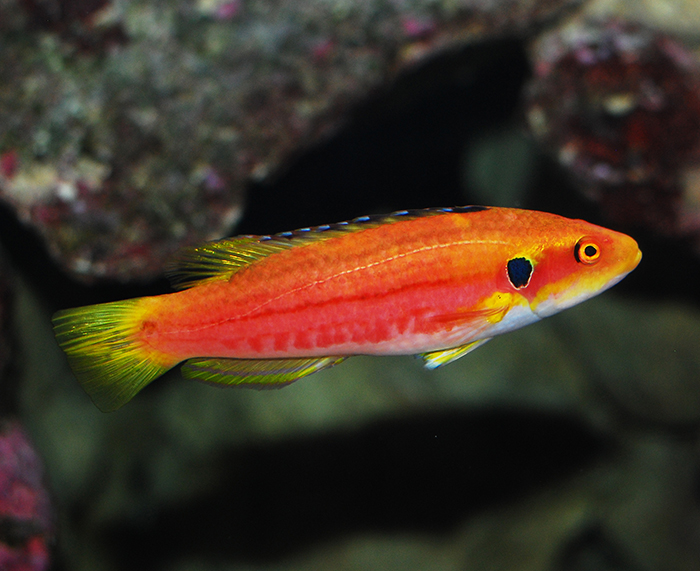
Adult specimens of strongly dichromatic genera such as Bodianus are typically easy to sex. Photo by Felicia McCaulley.
Green coloration is less common in fishes. Most typically, it is generated by way of yellow biochromes over some kind of bluish structural coloration (Hickman et al,1995).
Other interesting amalgamations of color cells may occur. In some cases, a single chromatophore may contain two or more types of biochromes. In some other cases, more complex coloration may be produced by a triple layer of chromatophores. For example, in the red seabream (Pagrus major), iridophores are overlaid with a layer of xanthophores and underlaid with a layer of melanophores. In yet some other cases, different types of chromatophores may be combined to form larger bodies called chromatosomes. For example, iridophores and melanophores may combine to form melaniridosomes (Hoar and Randall, 1969).
Workings of the Photophores
There are two ways in which objects can emit light; thermal radiation (or incandescence) which is related to the object’s temperature, or through chemical reactivity (or luminescence). Light produced by a living organism is referred to as bioluminescence. An enormous variety of terrestrial and aquatic plants, animals and microbes have the ability to generate visible light (Barnes-Svarney, 1995).
Although bioluminescence is not a function of fish coloration per se, it serves some of the same functions and is therefore worthy of discussion here. Bioluminescence has been observed in the Elasmobranchii as well as the Actinopterygii (Teleostei). Amongst the actinopterygians, it is believed to have independently evolved about 13 times. It is widely utilized by marine fishes, though it appears to be completely absent amongst freshwater species (Hoar and Randall, 1969; Sloman et al, 2005).
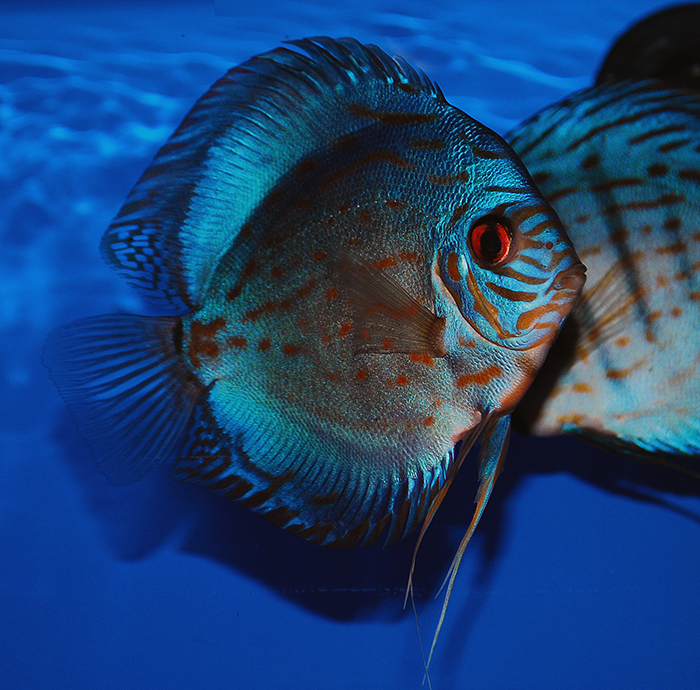
Over the last several decades, dedicated discus breeders have developed a number of attractive color varieties. Photo by Felicia McCaulley.
Fish produce some of the most intense bioluminescent displays of all animals. Among the very brightest is the flashlight fish (Anomalops spp.), which may be visible from as far away as 100 feet (30 meters) (Barnes-Svarney, 1995). Interestingly, the peak wavelength of this light is 500 nm, which is very close to that of peak light transmission in clear seawater as well as the peak spectral sensitivity of many fish opsins (Sloman et al, 2005).
Bioluminescent light is produced by photophores. The photophores of some fish species are rather simple, while those of some other species are highly complex. Depending upon the species, the placement of these light-emitting organs can occur anywhere on the body—in a central location (such as barbels), scattered over the body entire surface or embedded in the abdomen. The basic modes of light production in fishes include intracellular luminescence (i.e. light generated by the animal’s own tissues), extracellular luminescence (i.e. light generated by the secretion of a light-producing substance) and bacterial luminescence (i.e. light produced by endosymbiotic prokaryotes) (Hoar and Randall, 1969).
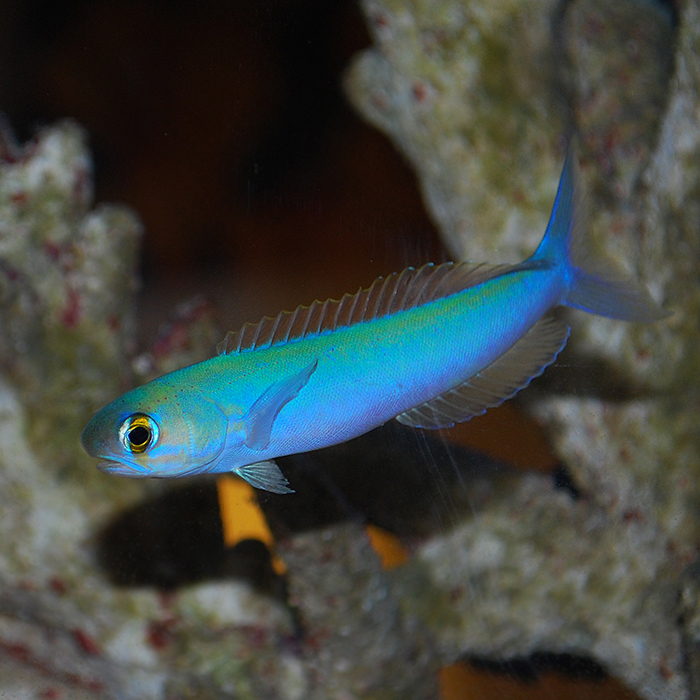
The speed with which the flashing tilefish (Hoplolatilus chlupatyi) can change color is astonishing. Photo by Felicia McCaulley.
The chemical process of bioluminescence involves the exposure of certain proteins (which some authors incorrectly refer to as pigments) to certain reactants. The most common of these proteins is luciferin. Luciferin emits heatless light when combined with oxygen by the enzyme luciferase (Levinton, 2001; Barnes-Svarney, 1995).
The luciferin-luciferase system generally emits bluish green light. However, the deep water-dwelling loosejaws (Malacosteidae) modify bioluminescent light with red fluorescent protein and several light filters, generating far-red light (over 700 nm). Not only do they use this light to locate prey, but can also use it to secretly communicate with conspecifics (most deep water fishes cannot detect light of this wavelength). Loosejaws themselves lack long-wave sensitive opsins and so rely on a special long-wave photosensitizer that is obtained from dietary chlorophyll (Sloman et al, 2005).
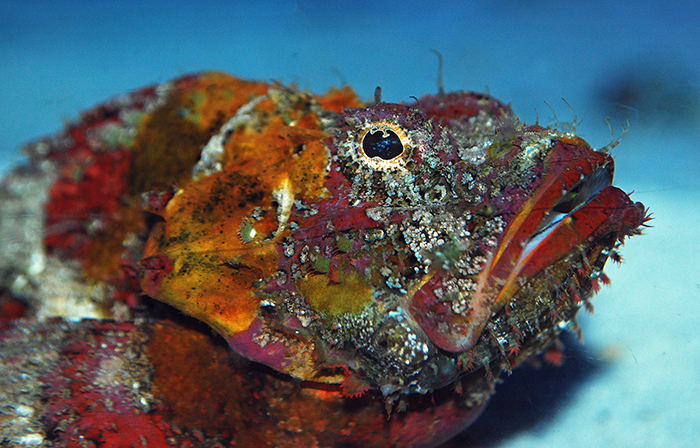
The vivid colors and complex patterns of many reef fishes account for much of the appeal of marine aquarium keeping. Photo by Felicia McCaulley.
Coloration of Captive Fishes
Ornamental fish keepers, diligently working to expand their palettes, have been selectively breeding fish for centuries; our obsession with brightly colored specimens is hardly new. That being said, modern technologies have enabled producers to dramatically modify these animals at an unprecedented pace. This has resulted in some highly questionable practices. To give consumers that which nature cannot speedily provide alone, ornamental fish producers have resorted to “improving” the appearance of numerous species by injecting them with dyes, by juicing them with hormones and even by genetically modifying them. While some simply dismiss such methods as distasteful, others go so far as to condemn them as unethical.
Maybe it is not the aim of every aquarist to perfectly simulate some singular natural habitat. Still, for any serious aquarist, the health of the animals in his or her keep should be a far greater concern than their value as “ornaments.” While it is not in any way wrong to be fond of the beauty of fish coloration, one should perhaps regard it primarily in terms of biology, and secondarily in terms of aesthetics. Accordingly, we should find the greatest satisfaction in coloration that indicates optimal health.
Captive fish will, in some cases, fade in coloration. To promote prime coloration, much less natural behavior, much less healthfulness, certain steps have to be taken to provide an ideal captive environment (Hemdal, 2006). One critical (yet frequently ignored) element here is lighting. The enclosure should be illuminated with light (including moonlight) that is as close as possible to the photoperiod, spectrum and intensity of that in the animal’s natural environment. Obviously, the outward appearance of a fish will change perfunctorily under different lighting. What is less evident, but much more significant, is that because they are physiologically responsive to changes in illumination (regulation of diurnal pattern, synchronization of spawning, etc.), the actual appearance of a fish may change under different lighting. For example, male clown wrasses (Halichoeres maculipinna) can be induced to display their bright, nuptial coloration if photoperiod (in tandem with temperature) is made to simulate the transition from spring to summer conditions (Holt and Riley, 1999).

In part because of its heavily pigmented teeth, the harlequin tuskfish (Choerodon fasciatus) makes for an especially striking ornamental fish. Photo by Felicia McCaulley.
To some extent, the physical layout of the aquarium can have an impact on a fish’s coloration. For example, if an aquascape is lacking in adequate places of shelter, subordinate members in a group of blue damselfish (Chrysiptera cyanea) will assume a darker, less conspicuous color (i.e. fright coloration). Even more dramatically, cryptic fishes that are capable of physiological color change will vary in appearance with changes in the appearance of the rock and substrate in their environment.
Arguably, the greatest influence on fish coloration is diet. Like all animals, a fish’s food must include carbohydrates, fats, proteins, vitamins, minerals and water. While plants and prokaryotes are capable of synthesizing all of the organic compounds needed for their survival, animals must obtain certain compounds indirectly from a dietary source. “Essential nutrients” are required for normal biological function but cannot be endogenously synthesized and therefore must be obtained through the animal’s diet (Hemdal, 2006; Hickman et al, 1995). A good deal of fish pigments are derived from essential nutrients.
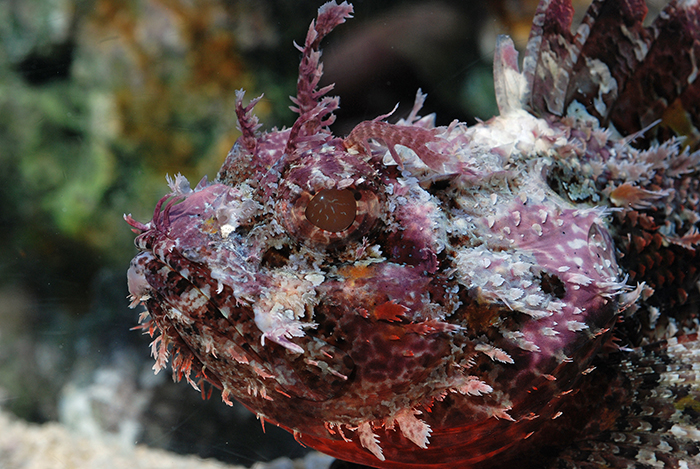
Nervous and hormonal control over chromatophores allows for rapid physiological color change. Photo by Felicia McCaulley.
The carotenoids are patent examples of such pigments. These compounds (over 600 thus far discovered) are synthesized by a great diversity of plants ranging from diatoms to sugar maples. Astaxanthin—an especially important carotene—is more directly taken up by fishes and appears to attach to special proteins, altering the refractive index of the animal’s tissue. Supplemental astaxanthin is responsible for the “natural” red hue of the flesh of farm-raised salmon (Hemdal, 2006).
Wild fishes, of course, obtain carotenoids as they are passed up the food chain. Captive fishes depend upon the inclusion of key carotenoids to their feed preparations. Many fishkeepers opt for a combination of carotenoid-rich whole items such as certain shrimp, krill and red marine algae. Those who rely on more heavily processed feeds (flakes, pellets, etc.) must see to it that they contain the necessary range and quantity of carotenoids. In addition to pure, extracted forms of carotenoids (e.g. astaxanthin), synthetic forms (e.g. canthaxanthin)are available for use as supplements (Hemdal, 2006; McKinnon, 2013).
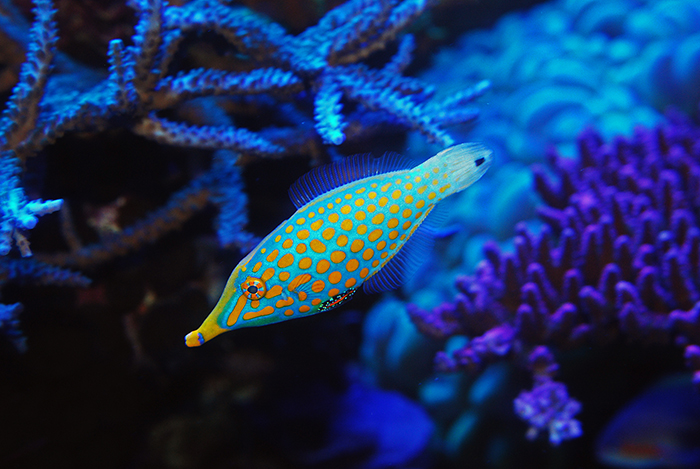
Captive fish will display their most natural coloration only under ideal living conditions. Photo by Felicia McCaulley.
Many feed recipes (e.g. frozen artemia) include spiralina. It is for good reason; this cyanobacterium is an excellent source of protein, as well as important pigments including beta carotene, lutein, chlorophyll a, xanthophyll and zeaxanthin. Certain phycocyanins found in spiralina are thought to contribute to blue coloration in fishes (McKinnon, 2013).
For those who wish to formulate and prepare their own fish feeds, a wide variety of pigment-rich fruits and vegetables such as bell pepper, blueberries, broccoli, brussel sprouts, cantaloupe, carrot, collard, dill weed, kale, mustard greens, peas, pumpkin, romaine lettuce and winter squashhas been suggested .A list of purported color-enhancing herbs includes paprika, rosehips and Goji cherry as well as the flowers of dandelion, nasturtium and marigold (McKinnon, 2013).
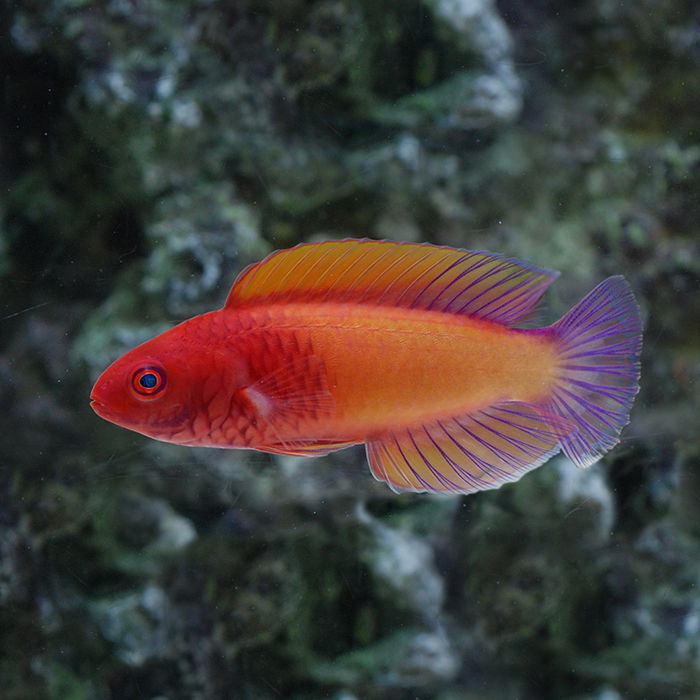
A ruddy fish such as the red velvet wrasse (Cirrhilabrus rubrisquamis) presumably will look its best only when fed a diet rich carotenes and xanthophylls. Photo by Felicia McCaulley.
Pigment-rich whole food items as well as supplements can be added to specialized color-enhancing gel diets. One example of a basic gel-based fish food formula can be obtained at http://edis.ifas.ufl.edu/fa097.
References
Campbell, Neil A. and Jane B. Reece. Biology, Sixth ed. San Francisco: Benjamin Cummings, 2002.
Freeman, Scott. Biological Science. New Jersey: Prentice-Hall Inc., 2002.
Goda, Makoto, Yoshinori Fujiyoshi, Masazumi Sugimoto and Ryozo Fujii. “Novel Dichromatic Chromatophores in the Integument of the Mandarin Fish Synchiropus splendidus.” Biol. Bull. February 1, 2013 vol. 224 no. 1 14-17.
Hemdal, Jay F. Advanced Marine Aquarium Techniques. neptune City: T.F.H. Publications, Inc., 2006.
Hickman, Cleveland P. Jr. Integrated Principles of Zoology. Dubuque, IA: Wm. C. Brown Publishers, 1995.
Hoar, W.S. and D.J. Randall (eds). Fish Physiology. Vol. 3. Reproduction and Growth. Bioluminescence, Pigments and Poisons. New York: Academic Press, 1969.
Holt, Joan G. and Cecilia M. Riley. “Laboratory Spawning of Coral Reef Fishes – Effects of Temperature and Photoperiod.” U.S.-Japan Coop Program in Natural Resources (UJNR) Technical Report 28. 1999.
Lagler, Karl F., John E. Bardach and Robert R. Miller. Ichthyology. New York: John Wiley & Sons, Inc., 1962.
Livinton, Jeffrey S. Marine Biology: Function, Diversity, Ecology. New York: Oxford University Press, 2001.
Malek, Tiffany Bedizon. The Genetic Basis of Male Nuptual Color in the Threespine Stickleback, Gasterosteus aculeatus. Seattle: Proquest, 2008.
McKinnon, Melody. “Naturally enhance the color of aquarium fish.” http://allnaturalpetcare.com/blog/2013/12/28/naturally-enhance-color-aquarium-fish. 2013.
Michael, Scott W. Wrasses and Parrotfishes: The Complete Illustrated Guide to Their ID, Behaviors, and Captive Care. neptune City, NJ: T.F.H. Publications, 2009.
Sloman, Katherine A., Rod W. Vilson and Sigal Balshine. Behavior and Physiology of Fish, Volume 24. San Diego: Elsevier Science Publishing Co. Inc., 2005.
Springer, Victor G. and William F. Smith-Vaniz. Mimetic Relationships Involving Fishes of the Family Blenniidae. Washington: Smithsonian Institution Press, 1972.
Solomon, Eldra P., Linda R. Berg and Diana W. Martin. Biology, Sixth ed. South Melbourne: Thomson Learning Inc., 2002.
Thresher, R.E. Reproduction in Reef Fishes. neptune City, NJ: T.F.H. Publications, 1984.
0 Comments