Last time we explored the prokaryotic richness and diversity of hermatypic corals, which contextualised their role in homeostasis and the stability of the host-symbiont association, whereas herein we recapture our sense of wonder as we discover the microbial collaboration which protects and buffers the coral throughout environmental change.
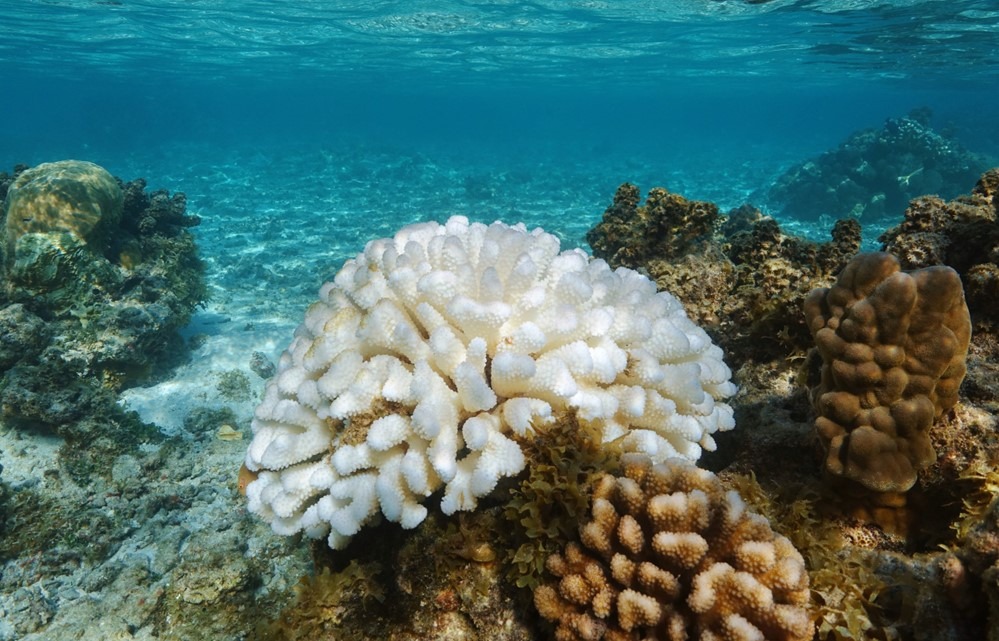
Fig 1. Phase 3. bleached Pocillopora species due to El Nino in Polynesian American Samoa in the Pacific.
Dysbiosis
The microbes of Scleractinia may serve as ecologically significant reservoirs of bacterial symbionts for diseased or dysbiotic colonies (McCauley et al. 2022). Replete mucosal microbes likely protect the coral by outcompeting opportunistic and obligate microscopic pathogens, and through manufacturing antimicrobial peptides (AMPs), bacteriocins, and fungicides (Nizet et al. 2000; Lopez et al. 2001; Fuller et al. 2002; Boilard et al. 2020; McCauley et al. 2022) whereas reactive oxygen species (ROS) are photosynthetic intermediates with biocidal properties (Boilard et al. 2020). Furthermore corals have an innate immune system that responds to injury or pathogenic invasion, where the conspicuous component the prophenoloxidase-activating system deposits infection-isolating and wound-sealing melanin after inactive prophenoloxidase is cleaved into active phenoloxidase (Cerenius et al. 2010, cited in Pollock et al. 2019).
Functional redundancy is likely pervasive amongst the stable microscopic communities of unstressed corals (Pollock et al. 2019). Prokaryotes likely bolster the host-symbiont association during mild to moderate stress through alterations in their gene expression and dynamic shifts in their population known as metagenomic plasticity (phase 1.). Microbial flexibility proposes corals restructure their communities via the sifting and selective nurture of residual symbionts, and/or environmental recruitment as a response to ecological change. Purging significant cross-sections of the microbiota is thus necessary albeit endurance relies upon timely reversion to richness and diversity. The coral adapts the types and numbers of its zooxanthellae when conditions exceed the restorative potential of the microbiota, which leads to variable and intended transitory states of bleaching (phase 2.). Nevertheless prolonged stress overwhelms the buffering capacity of the colony which elicits total loss of Symbiodiniaceae, significant microbiota variation, holobiont malfunction, disease, and moribundity (phase 3.; Fig 1.; Boilard et al. 2020).
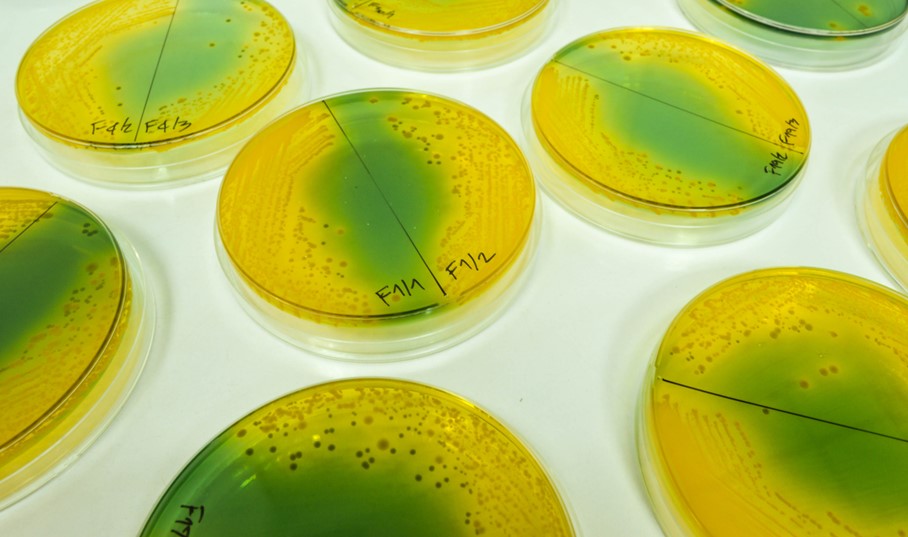
Fig 2. Laboratory agar culture of Vibrio species.
Extensive intrashelf colony degradation may induce wild ecological hypoxia (deoxygenation) that amplifies environmental anaerobia (Boilard et al. 2020) and lethal endogenous hydrogen sulphide (H2S).
LPS corals appear to regulate symbiont numbers yet this facility appears unavailable to SPS colonies which turn brown when their zooxanthellae over proliferate (Balling et al. 2008). Phase 2. and 3. bleaching is feasibly restricted to exocytosis in these corals (Starcevic et al. 2010) while symbionts leave LPS corals via the mesogleal canals and the mesenteries and exudation through the mouth (Rodriguez-Lanetty et al. 2005; Fujise et al. 2014). Alternatively they may escape from free-floating lysed cells that lack contact adhesion (Gates et al. 1992; Boilard et al. 2020; Xu et al. 2023) or become devoid of photoreactive pigments when ROS bleach the thylakoid membranes of their chloroplasts (Boilard et al. 2020; McCauley et al. 2022). Bleaching may be urgent and indiscriminate expulsion of cellular contents (cytosolic “bale out”) in response to a rise in ROS instigated by photosystem overload (Foyer et al. 1994; Fitt & Warner 1995; Müller-Moulé 2002; Starcevic et al. 2010; Fujise et al. 2014). However a recent study demonstrated that ROS are unlikely to be generated at a rate that would prove harmful and leak from zooxanthellae (Nielsen et al. 2018) yet a “quenching” mechanism in photosystem II of Cladocopium-like Symbiodiniaceae transfers surplus excitation energy to photosystem I which ceases photosynthetic electron transport and uses the energy to generate heat. Therefore the “trigger” may be a consequential loss of photosynthesis and nil exchange of oxygen and carbohydrates (Slavov et al. 2016). Nevertheless molecular trade in captive brown SPS corals is highly compromised (Balling et al. 2008; Cunning & Baker 2013) which enter a chronic dormancy with nil bleaching or polyp extension. Colony immunity may coordinate dysbiosis by initiating apoptotic, autophagic, or necrotic cascades (Gates et al. 1992; Boilard et al. 2020) whereupon adaptive signatures arise in the coral mucus which impact the composition of microbial populations (Ritchie & Smith 1995). Moreover corals undergoing stress or reproduction lose mucus and organic carbon which resists spring tide desiccation (Williams et al. 2015).
Corals express receptors on their epidermis which may selectively endocytose (internalise) species and strains of bacteria (Boilard et al. 2020) albeit this mechanism has facilitated the immunity insulated intracellular lifestyle of pathogenic bacteria in higher organisms (Andreoni & Magnani 2014). The vertical transmission of zooxanthellae from mother to offspring may promote the co-evolution of corals and their symbionts to suit regionalised environments (Boilard et al. 2020) which will impact their captive culture and preclude the seeding of specimens to geographically distant territories (Berger, personal communication). However the horizontal acquisition of free-swimming zooxanthellae (mastigotes) in skeleton-initiated first polyps (spats) and subsequent winnowing expedites environmentally aligned communities (Boilard et al. 2020).
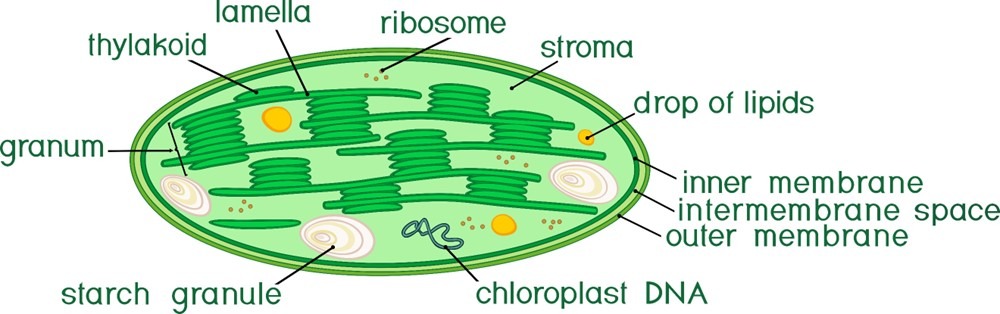
Fig 3. An illustration of chloroplast anatomy.
Short interfission, elevated mutation, gene dissemination, lysogenic/lytic ‘phage induction, contagion, and metabolic switching makes bacteria evolutionary prodigies. Prokaryotic gene expression adapts in phase 1., while photosynthetic Cyanobacteria and their plastids (@chloroplasts) proliferate which amplifies nitrogen fixation (diazotrophy) to compensate the colony in phase 2. Moreover microbiomes are flooded with bio-, fungi-cides, and ROS which precludes pathogen infiltration and expedites acclimation. 35 and 100 percent of the nutritional requirements of stage 3. Porites and Montipora species can be met by ingesting zooplankton (Boilard et al. 2020) which are only abundant in well-lit euphotic zones at night (Hays 2004).
The communities of corals endemic to unstable environments must attain greater metagenomic plasticity (Boilard et al. 2020). Mature colonies continuously shed mastigotes (Adams et al. 2009; Sharp et al. 2011) which elevates the local prevalence of heat tolerant Durusdinium-like species, whilst early vertical and horizontal transmission configures robust communities. Nonetheless evidence suggests heat-tolerant symbionts sustain decelerated growth (Boilard et al. 2020).
Symbiosis may shift to parasitisation where zooxanthellae instigate pathology in their host (Boilard et al. 2020) like brown SPS colonies chronically exposed to enriched nitrate where the coral becomes carbonate deprived (Hylkema 2014).
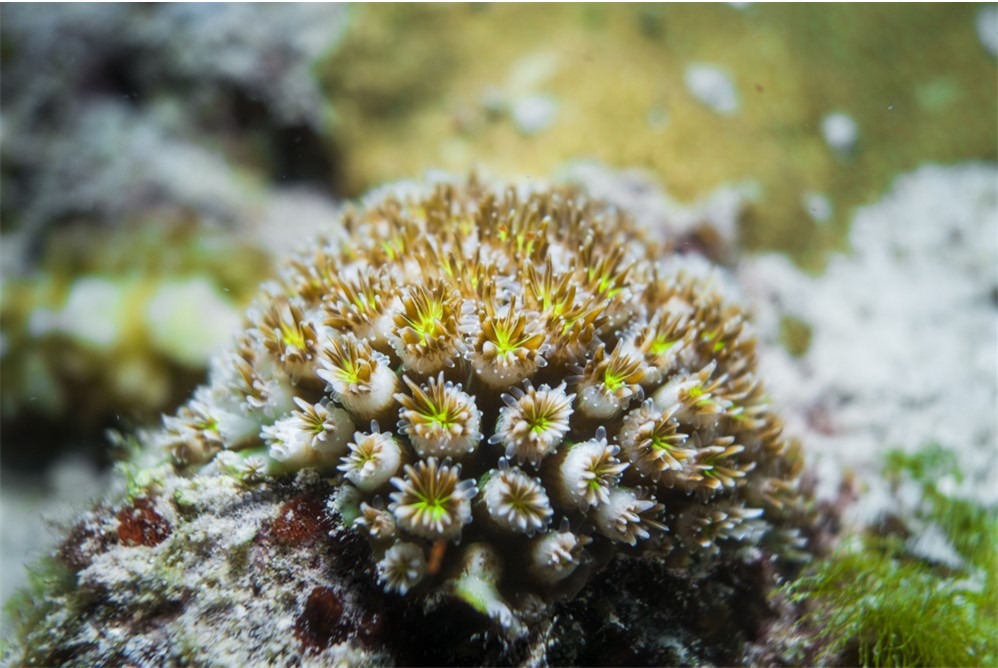
Fig 4. A juvenile colony of Galaxea species.
Acropora millepora were monitored mid-shelf at Hardy Reef 75 km off the Whitsunday Island group in the central region of the Great Barrier Reef (GBR). Prokaryotic amplicon sequence variants (ASVs; @genera) averaged 2,554 per sample from a possible 148,182. The phyla Proteobacteria, Xanthomonadales, and Oceanospirillales were common to 70, 33, and 15 percent of samples where Burkholderia species were identified in 80 percent. Baseline phenoloxidase activity was used as a proxy for immune function. The core prokaryotes of healthy A. millepora colonies diverged, and there was a loss of diversity and innate immunity two months before they developed white syndrome. Nosedives in micro- and macro-scopic heterogeneity have significantly perturbed captive and wild reef ecology (Aslett 2023b; Pollock et al. 2019) while functional redundancy declines during colonial stress, adaptation, and phase 1. and 2. dysbiosis (Pollock et al. 2019; Boilard et al. 2020). Moreover divergences in the microbial communities of intraspecific hosts, precede the onset of numerous coral diseases (Fig 2.; Pollock et al. 2019; Aslett 2024).
Vulnerability to any number of environmental conditions is conferred by genotype and varies amongst phylotypes (@ASVs), hence resilience to an infinite variety of external effects is stabilised by exceedingly heterogeneous and enriched communities. Population dynamics within limited ecological niches would thus moderate the disproportionate proliferation of a handful of affiliates that would otherwise outcompete and eradicate cross-sections of the holobiont, which would reinforce inertia with nominal host participation. However studies suggest that hosts raise prokaryotic function and place a ceiling on diversity (Xu et al. 2023).
The intracellular and intramucosal spaces in healthy corals are likely limited and finite like the internal surfaces of a captive reef system, whereas dysbiotic corals will be more analogous to a “blank canvas” where further colonisation and replication can occur. Thereupon environmental microbes would readily infect mucosal surfaces and would gain access to an intracellular lifestyle, where an increase in numbers and types of bacteria would expand rather than contract diversity (Xu et al. 2023). However replication of limited lineages within the confines of nonbleached corals would force taxa extinction and increase richness at the expense of multiplicity.
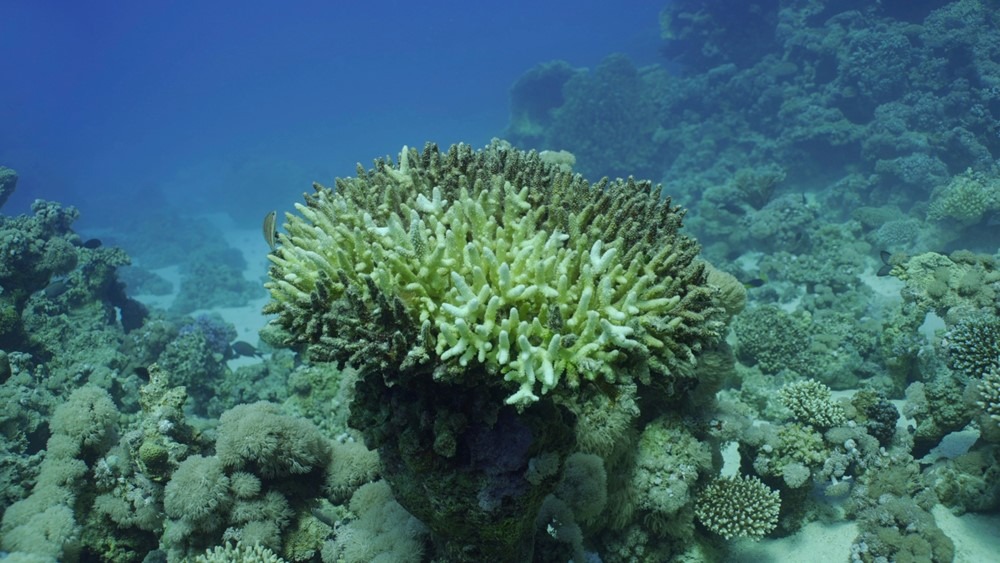
Fig 5. Partially bleached Acropora species.
The microbial communities of bleached and nonbleached examples of Acropora digitifera, Galaxea fascicularis, and Porites pukoensis were studied off Luhuitou peninsula, Hainan Island, the South China Sea. Phylotypes ranged from ~1,700 to over 6,000 per colony where composition, richness, and diversity were peculiar to each species (Fig 4.; Fig 5.; McCauley et al. 2022; Xu et al. 2023).
Detected Bacteria and Archaea included 66 phyla, 183 classes, 456 orders, 790 families, 1,644 genera, and 3,283 species. The phyla Proteobacteria and Bacteroidota were most abundant whereupon Firmicutes, Cyanobacteria, and Actinobacteriota predominated. The classes Gammaproteobacteria and Bacteriodia were enriched in healthy corals whereas Alphaproteobacteria were conspicuous in bleached. The orders Rickettsiales, Chloroplast, and Chlorobiales were foremost in zooxanthellate A. digitifera, G. fascicularis, and P. pukoensis which were reduced along with Burkholderiales in azooxanthellate colonies. Dysbiosis instigated proliferation of Lachnospirales and Enterobacterales in A. digitifera; Rhodobacterales, Rhizobiales, and Peptostreptococcales-Tissierellales in G. fascicularis, and Rhodobacterales, Rhizobiales, and Flavobacteriales in P. pukoensis. The bacterial compositions of seawater and dysbiotic colonies were more alike than seawater and unbleached specimens, which appeared competent at moulding rich and diverse populations. The genera Ruegeria, Methyloceanibacter, Filomicrobium, Halioglobus, Rubripirellula, Rhodopirellula, Silicimonas, Blastopirellula, Woeseia, and members of the class Gammaproteobacteria multiplied disproportionately in bleached colonies (Xu et al. 2023).
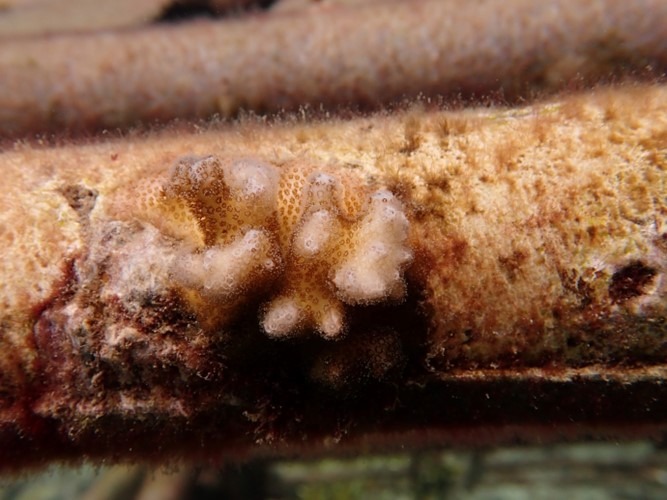
Fig 6. A juvenile colony of Pocillopora species encrusted on a pipe at a coral nursery in Malaysia.
Hitherto considered probiotic Erythrobacter, Endozoicomonas, and Ruegeria species predominated in dysbiotic colonies which suggests a protective role, whereas prokaryotes synthesised secondary metabolites, amino acids, and metabolised carbon (Xu et al. 2023).
The study concluded that healthy hosts modulate microbial populations which retain an inertia and are species-specific, however dysbiosis leaves the coral vulnerable to colonisation with waterborne bacteria which increases diversity. Furthermore these blooms may assist postbleaching recouperation or may facilitate colonisation with heterogeneity-curtailing pathogens (Rosenberg et al. 2007; Munn 2019; Xu et al. 2023).
The microscopic communities of corals revert to prestressed profiles following phase 2. and 3. dysbiosis, and thus modified populations are only transitory on the scale of colony recuperation which frequently takes years. The heat tolerance of zooxanthellae declines throughout viral lysogeny, whereas lytic induction is elicited by anomalous temperatures and irradiance (Munn 2019; Boilard et al. 2020).
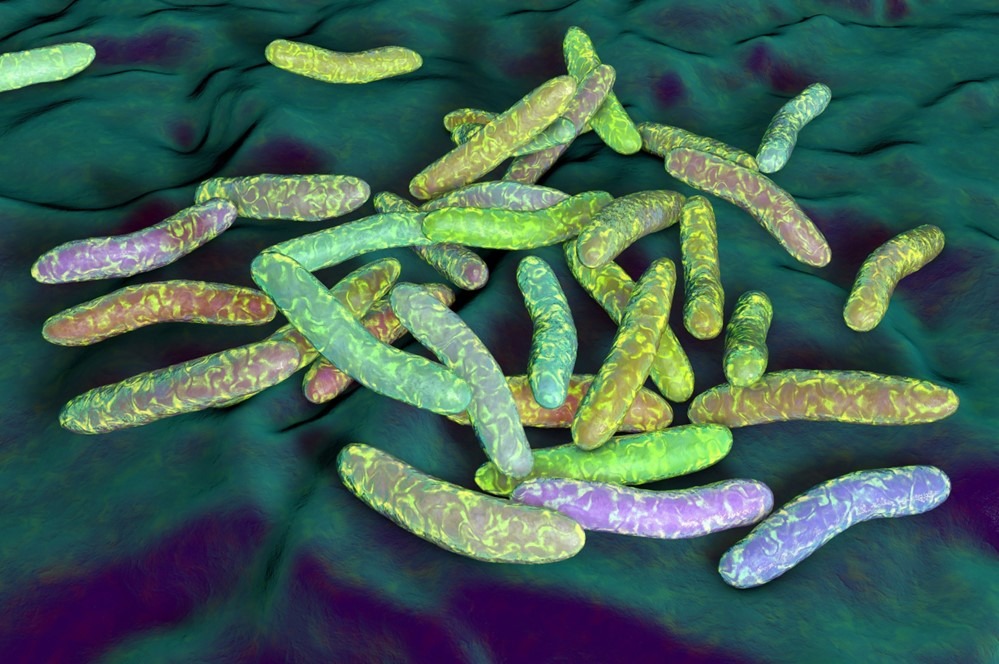
Fig 7. A representation of tetrodotoxin-secreting Pseudoalteromonas tetraodonis living in pufferfish mucus.
Prokaryotic communities are susceptible to probiotic manipulation or restoration with engineered microbes. Moreover beneficial microorganisms for corals (BMCs) including five species of Pseudoalteromonas, Halomonas taeanensis and a Cobetia marina-related strain have enhanced stress tolerance and postbleaching survivorship and recuperation (Fig 7.; Rosado et al. 2019; Boilard et al. 2020; Santoro et al. 2021).
The hitherto overlooked and hidden heterogeneity of the microeukaryotes of the coral holobiont are explored in part V.
Learn more about the author and his amazing work HERE!
References
Adams, L., M., Cumbo, V., R. & Takabayashi, M. (2009) Exposure to sediment enhances primary acquisition of Symbiodinium by asymbiotic coral larvae. Marine Ecology Progress Series. 377, 149-156.
Ainsworth, D., T., Krause, L., Bridge, T., Torda, G., Raina, J., Zakrzewski, M., Gates, R., Padilla-Gamiño, J., Spalding, H., Smith, C., Woolsey, E., Bourne, D., Bongaerts, P., Hoegh-Guldberg, O. & Leggat, W. (2015) The coral core microbiome identifies rare bacterial taxa as ubiquitous endosymbionts. The ISME Journal. 9(10), 2261-2274.
Andreoni, F. & Magnani, M. (2014) Photobacteriosis: Prevention and Diagnosis. Journal of immunology research. 2014,.
Aslett, C., G. (2024) The Complete Reef Aquarist, for hobbyists, the trade and academics – A Conservation Manual. Aslett, C., G. (ed.). Reef Ranch Publishing Ltd, Leeds, UK. In Press.
Aslett, C., G. (2023b) Holosystemics Part I: Captive Reef Function versus Malfunction. https://reefranch.co.uk
Balling, H., W., Janse, M. & Sondervan, P. (2008) Trace elements, functions, sinks and replenishment in reef aquaria. Advances in coral husbandry in public aquariums. Leewis, R., J. & Janse, M. (eds.). Burgers’ Zoo, Arnhem, The Netherlands. pp 143-156.
Berger, M. The University of Leeds, UK.
Biebl, H., Allgaier, M., Tindall, B., J., Koblizek, M., Lunsdorf, H. & Pukall, R. (2005) Dinoroseobactershibae gen. nov., sp. nov., a new aerobic phototrophic bacterium isolated from dinoflagellates. Int J Syst Evol Microbiol. 55, 1089-1096.
Boilard, A., Dubé, C., E., Gruet, C., Mercière, A., Hernandez-Agreda, A. & Derome, N. (2020) Defining Coral Bleaching as a Microbial Dysbiosis within the Coral Holobiont. Microorganisms. 8, 1682. doi:10.3390/microorganisms8111682
Cerenius, L., Kawabata, S., Lee, B., L., Nonaka, M. & Soderhall, K. (2010) Proteolytic cascades and their involvement in invertebrate immunity. Trends Biochem. Sci. 35, 575-583. doi:10.1016/j.tibs. 2010.04.006
Cunning, R. & Baker, A., C. (2013) Excess algal symbionts increase the susceptibility of reef corals to bleaching. Nature Climate Change. 3, 259-262.
Hays, G., C. (2004) A review of the adaptive significance and ecosystem consequences of zooplankton diel vertical migrations.
Hydrobiologia. 503, 163-170.
Hylkema, A. (2014) Decreased growth of Stylophora pistillata with nutrient-driven elevated zooxanthellae density is largely explained by DIC limitation. Reefs.com. https://reefs.com/magazine/decreased-growth-of-stylophora-pistillata-with-nutrient-driven-elevated-zooxanthellae-density-is-largely-explained-by-dic-limitation/
Fuhrman, J., A. & Campbell, L. (1998) Microbial microdiversity. Nature. 393, 410-411.
Fujise, L., Yamashita, HY., Suzuki, G., Sasaki, K., Liao, L., M. & Koike, K. (2014) Moderate Thermal Stress Causes Active and Immediate Expulsion of Photosynthetically Damage Zooxanthellae (Symbiodinium) from Corals. PLOS ONE. DOI:10.1371/journal.pone.0114321
Garren, M., Raymundo, L., Guest, J., Harvell, C., D. & Azam, F. (2009) Resilience of coral¬ associated bacterial communities exposed to fish farm effluent. PLoS One. 4, e7319. doi: 10.1371/journal.pone.0007319
Gates, R., D., Baghdasarian, G. & Muscatine, L. (1992) Temperature stress causes host cell detachment in symbiotic cnidarians: implications for coral bleach-ing. Biol Bull. 182, 324–332. https://doi.org/10.2307/1542252
Guo, C., Chen, W., Yuan, L., Yang, L., Weng, S., Yu, X. & He, J. (2011) The viral ankyrin repeat protein (ORF124L) from infectious spleen and kidney necrosis virus attenuates nuclear factor-κB activation and interacts with IκB kinase β. The Journal of general virology. 92(7), 1561-1570.
McCauley, M., Goulet, T., L., Jackson, C., R. & Loesgen, S. (2022) Systematic review of cnidarian microbiomes reveals insights into the structure, specificity, and fidelity of marine associations. Nature Communications. 14, 4899. https://doi.org/10.1038/s41467-023-39876-6
Mouchka, M., E., Hewson, I. & Harvell, C., D. (2010) Coral¬associated bacterial assemblages: current knowledge and the potential for climate-driven impacts. Integr. Comp. Biol. 50, 662-674. doi: 10.1093/icb/icq061. pmid:21558231
Munn, C., B. (2019) Marine Microbiology: Ecology & Applications, Third Edition. Munn, C., B. (ed.). CRC Press, Taylor & Francis Group, London. pp 273-326.
Nielsen, D., A., Petrou, K. & Gates, R., D. (2018) Coral bleaching from a single cell perspective The ISME Journal. 12, 1558–1567. https://doi.org/10.1038/s41396-018-0080-6
Nissimov, J., Rosenberg, E. & Munn, C., B. (2009) Antimicrobial properties of resident coral mucus bacteria of Oculina patagonica. FEMS Microbiol Lett. 292, 210-215. doi: 10.1111/j.1574¬6968.2009.01490.x.
Pollock, F., J., Lamb, J., B., van de Water, J., Smith, H., A., Schaffelke, B., Willis, B., L. & Bourne, D., G. (2019) Reduced diversity and stability of coral-associated bacterial communities and suppressed immune function precedes disease onset in corals. R Soc Open Sci. 6, 190355. https://doi.org/10.1098/rsos .190355
Pollock, F.,J., McMinds, R., Smith, S., Bourne, D., G., Willis, B., L., Medina, M., Thurber, R., V. & Zaneveld, J., R. (2018) Coral-associated bacteria demonstrate phylosymbiosis and cophylogeny. Nat Commun. 9, 4921.
Preston, E. (2015) Deadly “White Syndrome” Kills Coral, and it’s Spreading. Hakai Magazine, Costal Science and Societies. https://www.hakaimagazine.com/news/deadly-white-syndrome-kills-coral-and-its-spreading/
Ritchie, K., B. & Smith, G., W. (1995) Preferential carbon utilization by surface bacterial communities from water mass, normal, and white-band diseased Acropora cervicornis. Mol Mar Biol Biotechnol. 4, 345-354.
Rodriguez-Lanetty, M., Scaramuzzi, C., Quinnell, R. & Larkum, A. (2005) Transport of symbiotic zooxanthellae in mesogleal canals of Zoanthus robustus? Coral Reefs. 24, 195-196.
Rohwer, F., L., Seguritan, V., Azam, F. & Knowlton, N. (2002) Diversity and distribution of coral-associated bacteria. Mar Ecol Prog Ser. 243, 1–10.
Rosado, P., M., Leite, D., C., Duarte, G., A., Chaloub, R., M., Jospin, G., da Rocha, U., N., Saraiva, J., P., Dini-Andreote, F., Eisen, J., A. & Bourne, D., G. (2019) Marine probiotics: Increasing coral resistance to bleaching through microbiome manipulation. ISME J. 13, 921.
Santoro, E., P., Borges, R., M., Espinoza, J., L., Freire, M., Messias, C., S., M., A., Villela, H., D., M., Pereira, L., M., Vilela, C., L., S., Rosado, J., G., Cardoso, P., M., Rosado, P., M., Assis, J., M., Duarte, G., A., S., Perna, G., Rosado, A., S., Macrae, A., Dupont, C., L., Nelson, K., E., Sweet, M., J., Voolstra, C., R. & Peixoto, R., S. (2021) Coral microbiome manipulation elicits metabolic and genetic restructuring to mitigate heat stress and evade mortality. Science advances. 7(33), 3088.
Sekar, R., Kaczmarsky, L., T., Richardson, L., L. & Chardson, L., L. (2008) Microbial community composition of black band disease on the coral host Siderastrea siderea from three regions of the wider Caribbean. Mar Ecol Prog Ser. 362, 85-98.
Sharp, K., H., Distel, D. & Paul, V., J. (2011) Diversity and dynamics of bacterial communities in early life stages of the Caribbean coral Porites astreoides. The Isme Journal. 6, 790.
Slavov, C., Schrameyer, V., Reus, M., Ralph, P., J., Hill, R., Büchel, C., Larkum, A., W., D. & Holzwarth, A., R. (2016) “Super-quenching” state protects Symbiodinium from thermal stress — Implications for coral bleaching. Biochimica et Biophysica Acta. 1857, 840–847.
Sunagawa, S., Woodley, C. M. & Medina, M. (2010) Threatened corals provide underexplored microbial habitats. PLoS ONE. 5, e9554.
Tait, K., Hutchison, Z., Thompson, F., L. & Munn, C., B. (2010) Quorum sensing signal production and inhibition by coral‐associated vibrios. Environmental Microbiology Reports. 2, 145-150.
evolution, development, and ecological interactions. Frontiers in Cellular and Infection Microbiology. 4,.
Williams, A., D., Brown, B., E., Putchim, L. & Sweet, M., J. (2015) Age-related shifts in bacterial diversity in a reef coral. PLoS ONE. 10, 1–16.
Xu, M., Cheng, K., Xiao, B., Tong, M., Cai, Z., Jong, M-C., Chen, G. & Zhou, J. (2023) Bacterial Communities Vary from Different Scleractinian Coral Species and between Bleached and Non-Bleached Corals. Microbiology Spe ctrum. 11(3),.
Ziegler, M. et al. (2016) Coral microbial community dynamics in response to anthropogenic impacts near a major city in the central Red Sea. Mar. Pollut. Bull. 105, 629–640.
0 Comments