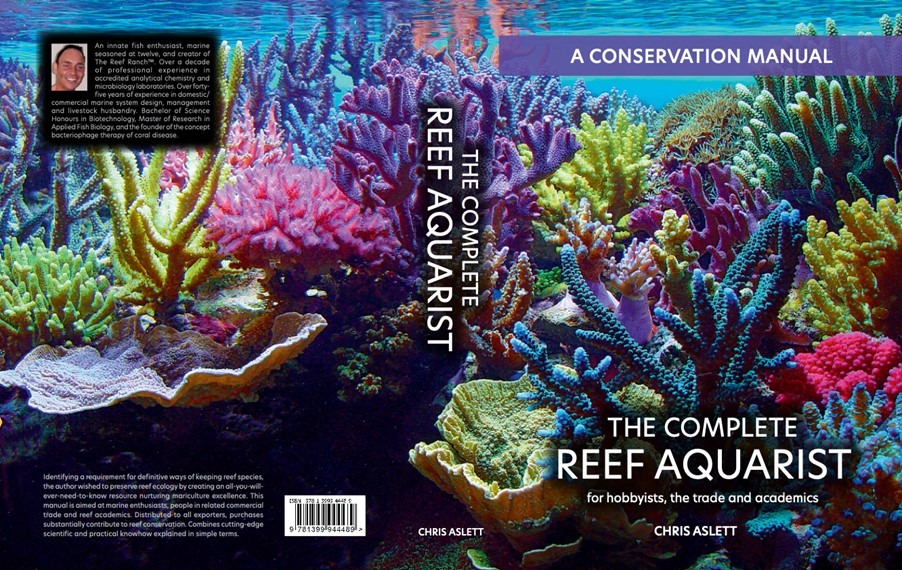
Available from late summer/autumn 2023
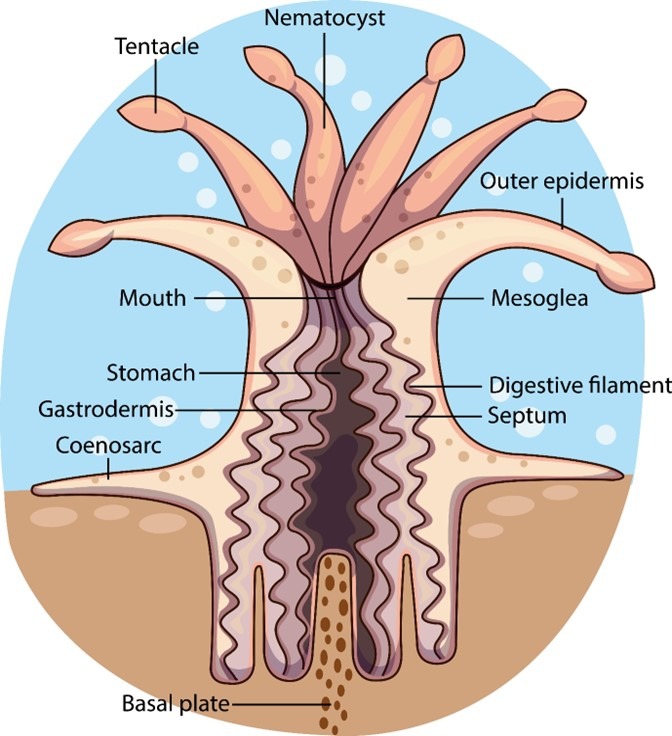
Fig 1. A whimsical representation of polyp morphology
Part I explored the food webs within captive reefs with reference to their micro- and macro-biota, nutrients and their management, and explained how imbalances can expedite and underpin malfunction, which is the foundation upon which we now broaden, deepen and consolidate our understanding. Comprehensive appreciation of the coral holobiont is central to the contextual positioning of Scleractinia within captive reef superorganisms (holosystems).
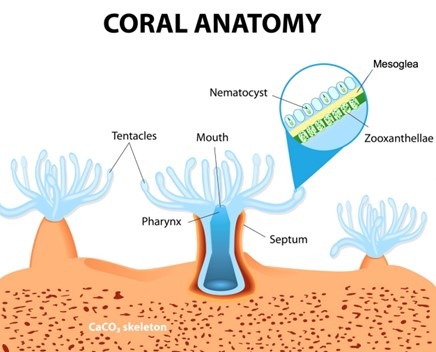
Fig 2. A representation of a 2 mm (0.08”) wide polyp where noncellular gelatinous mesoglea separates the ectoderm from the endoderm (Sarras et al. 1991).
“Hard rayed” corals of the order Scleractinia emerged approximately two hundred and fifty million years ago around the time of the first terrestrial dinosaurs (Waggoner et al. 1994) yet their evolution to present-day Scleractinia coincided with the rise of their dinoflagellate symbionts (zooxanthellae) ninety million years later. The delay in their development was a legacy from the mass extinction that occurred forty million years before which halted the epoch’s primordial photosynthetic endosymbiosis (LaJeunesse et al. 2018).
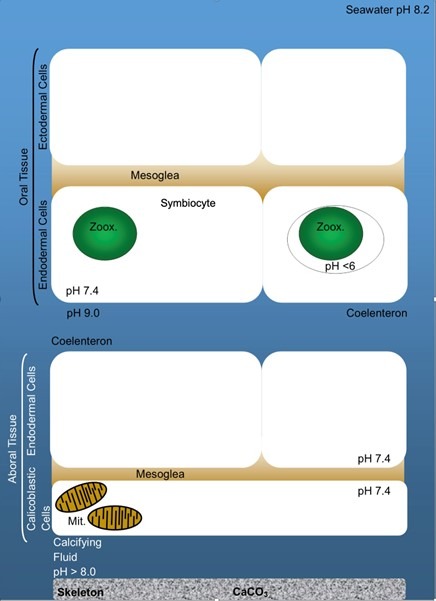
Fig 3. An illustration of coral cell types and zooxanthellae (Zoox.) occupying a symbiosome within a symbiocyte, where skeletal accretion occurs beneath mitochondrial (Mit.)-rich calicoblastic cells. Adapted from Zoccola et al. 2015.
The ectoderm and endoderm of a coral is derived from two types of embryonic stem cell which form the epidermis and gastrodermis, where multiple cell types develop from each progenitor (Fig 2.; Fig 3.; Putnam et al. 2017). Polyps have a tube-like body crowned by an oral disc fringed by tentacles tipped with stinging cnidocytes surrounding a central mouth adjoined by a layer of tissue called the coenosarc (Fig 1.; Allemand et al. 2004) which produce mucus, slough sediment, and capture and sting prey.
Zooxanthellae range from 6 to 12 microns (micrometres; µm; x 10-6 m) which are ranked in the kingdom Chromista, the subkingdom Harosa, the infrakingdom Alveolata, the phylum Myzozoa, the subphylum Dinozoa, the infraphylum Dinoflagellata, the class Dinophyceae, the order Suessiales, and the family Symbiodiniaceae (WoRMS 2021; LaJeunesse et al. 2018). Their host cultivates them in membranous organelles called symbiosomes within endodermal symbiocytes that are associated with a noncellular gelatinous matrix called mesoglea (Fig 3.; Putnam et al. 2017). They are mesokaryotes that comprise chloroplasts and nuclei with permanently condensed chromosomes while their free-swimming uniocular mastigote uses two flagella to locate a host. Their photopigments include chlorophylls a and c2, β-carotene and the xanthophylls: diatoxanthin, dinoxanthin, fucoxanthin, neodinoxanthin, neoperidinin, and peridinin (Fig 5.; Levy et al. 2003; LaJeunesse et al. 2018; Guiry 2019).
Microbes including symbionts are acquired in several ways. Maternal colonies infect brood larvae (planulae) or gamete mucus, or they are recruited from the environment through the mouths of first polyps, compound planktonic planulae, or mature colonies (Babcock 1985; Morse et al. 1988; Boch & Morse 2012; Sharp et al. 2012; Thompson et al. 2015) while symbiosome residency induces the coccoid form (Fig 4.; Putnam et al. 2017).
Distinct monophyletic lineages descended from a common ancestor (clades), species, and strains of zooxanthellae retain various proficiencies for manufacturing antioxidants, withstanding irradiance and heat, or exchanging photosynthate, while the coral liberates molecules that coordinate their photosynthesis and modify the permeability of their membrane (Munn 2019). Their alliance provides the coral with oxygen (O2), carbohydrates, glycerol, and amino acids whereas the “algae” receive nitrogen (N), phosphorus (P), and carbon dioxide (CO2; Dunn et al. 2012; Ferrier‐Pagès et al. 2016; Boilard et al. 2020).
The adaptive bleaching hypothesis proposes the coral constantly regulates the types and numbers of its “algal” partners to winnow the community to the prevailing environment (Fautin & Buddemeier 2004; Munn 2019). LPS corals liberate partially digested zooxanthellae from polyp mouths while nitrate causes harmful congestion of SPS corals (Rodriguez-Lanetty et al. 2005; Balling et al. 2008; Cunning & Baker 2013). The cells of heat stressed corals lose their cell-to-cell adhesion, float free, and degrade (Gates et al. 1992; Boilard et al. 2020; Xu et al. 2023) whereas photoreactive pigments are lost from chloroplast thylakoid membranes when they are exposed to reactive oxygen species (ROS; Boilard et al. 2020; McCauley et al. 2022). Yet merely nominal ROS amass (Nielsen et al. 2018) but photosystem II of Cladocopium-like Symbiodiniaceae conveys surplus excitation energy to photosystem I which becomes heat, as photosynthetic electron transport and photosynthate exchange are terminated (Slavov et al. 2016). Otherwise apoptotic, autophagic, exocytotic, or necrotic cascades may initiate loss of zooxanthellae (Gates et al. 1992; Boilard et al. 2020). Notwithstanding dysbiosis purges the biochemicals responsible for colouration because all zooxanthellae are brown (Yu 2000). Yet they comprise fluorescent and photoactive proteins and amino acids while they impart an opacity that host molecules augment (Béraud et al. 2013; Ferrier‐Pagès et al. 2016). Their mycosporine-like amino acids (MAAs) referred to as “sunscreens” are merely protective against 310-nanometre (nm) UVB and above, which include mycosporine-2 glycine, mycosporine-glycine, mycosporine-NMA serine, mycosporine-NMA threonine, palythine serine sulphate, palythine serine, phorphyra, playthine, playthinol, and shinorine (Fig 5.; Riddle 2004; Riddle 2014). Some hosts also produce MAAs (Shinzato et al. 2011).
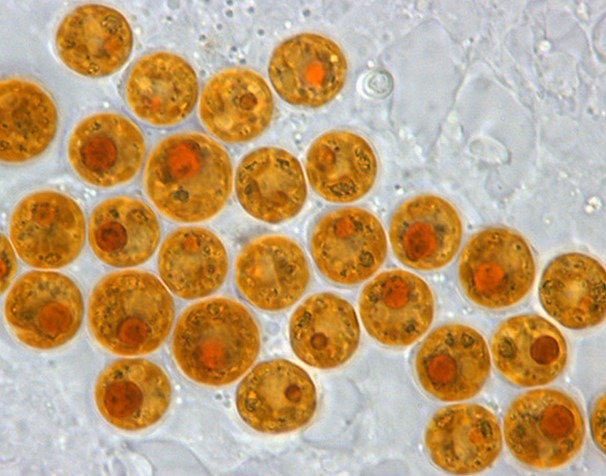
Fig 4. A micrograph of coccoid and reclassified Symbiodinium “fitti” common to Caribbean acroporids and giant clams. Image courtesy of Todd C. LaJeunesse©.
Ribosomes are intracellular machines that assemble and bond amino acids into the polypeptide backbone of a protein, which comprise protein subunits and functional molecules of ribosomal ribonucleic acid (rRNA). Ribosomes are enzymatically cleaved in laboratories to yield their constituent proteins and rRNA molecules which differ in sequence according to lineage (Stryer 1995b). rRNA analysis is thus used to phylogenetically discern the evolutionary development of species.
The svedberg (S) is a settlement coefficient that relates to the rapidity of sedimentation during centrifugation and thus particle size. 1S is equal to 10-13 seconds (100 femtoseconds) which expresses the size of proteins (Stryer 1995a).
Zooxanthellae have evolved divergently over one hundred and sixty million years during which they endured significant environmental change. Previously considered merely members of the genus Symbiodinium, they are a diverse supergroup of the family Symbiodiniaceae which may total several hundred species. Their diverse clades discerned using ecological and biogeographic evidence supported by nuclear large subunit (LSU) ribosomal deoxyribonucleic acid (rDNA) and mitochondrial cytochrome b (mt cob) sequence analysis, are considered the equivalent of genera which have been redefined using species type. Fifteen clades/subclades were proposed and ranked according to genus in 2018 which were subdivided according to host and climate: clade A (temperate); clade A (Symbiodinium); clade B (Breviolum); clade C (Cladocopium); clade D (Durusdinium); clade D (foraminifera); clade E (Effrenium); clade Fr 2; clade Fr 3; clade Fr 4; clade Fr 5 (Fugacium); clade G (Gerakladium); clade G (foraminifera); clade H, and clade I, which included a comprehensive account of the following seven (LaJeunesse et al. 2018).
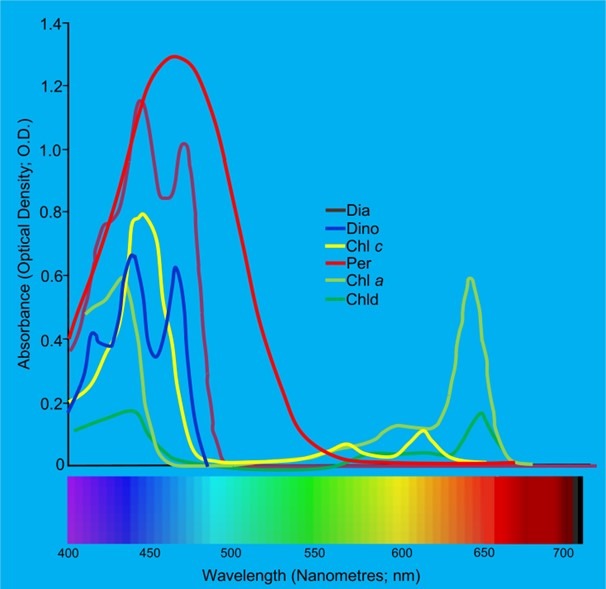
Fig 5. The absorption spectra of the various photoactive biochemicals found in the symbionts of Dipsastraea favus: Chl (chlorophyll); Chld (chlorophyllidae); Dia (diatoxanthin); Dino (diadinoxanthin), and Per (perdinin). Adapted from Levy et al. 2003.
The reclassification of clade A to the genus Symbiodinium refuted the previously described species S. microadriaticum and S. “fitti” and reaffirmed S. natans as the species type with affiliates ranging from free-living to opportunistic parasites/pathogens and endosymbionts, which are intense to variable irradiance adapted and MAA-secreting. Symbiodinium means “living together” (LaJeunesse et al. 2018).
Clade B was realigned with the genus Breviolum with a species type of B. minutum comprising undersized members associated with organisms of the class Cnidaria (LaJeunesse et al. 2018) like corals, anemones, jellyfish, and hydroids which utilise cnidocyte stinging cells (nematocysts; Fig 6.; Technau & Steele 2011; Beckmann & Özbek 2012). B. minutum-like zooxanthellae are cosmopolitan inasmuch as they occur in hosts from various climes, but they inhabit shallow inshore corals in temperate zones and those in euphotic to mesophotic elevations in tropical seas. Their name is derived from “small ones”, and a cluster of recently evolved sister taxa predominate in the wider Caribbean where they partner with “soft” and “hard” corals (LaJeunesse et al. 2018).
The genus Cladocopium (clade C) has C. goreaui-type affiliates that are extensively distributed in most habitats, with diverse morphotypes comprising mastigotes with compressed apical vesicles. They tolerate varying degrees of illumination and temperature and exhibit extraordinary host fidelity in ciliates, clams, numerous Cnidaria, flatworms, foraminifera, and sponges. Notwithstanding non-host-specialised members are abundant throughout the Indo-Pacific where their name means “plentiful” (LaJeunesse et al. 2018).
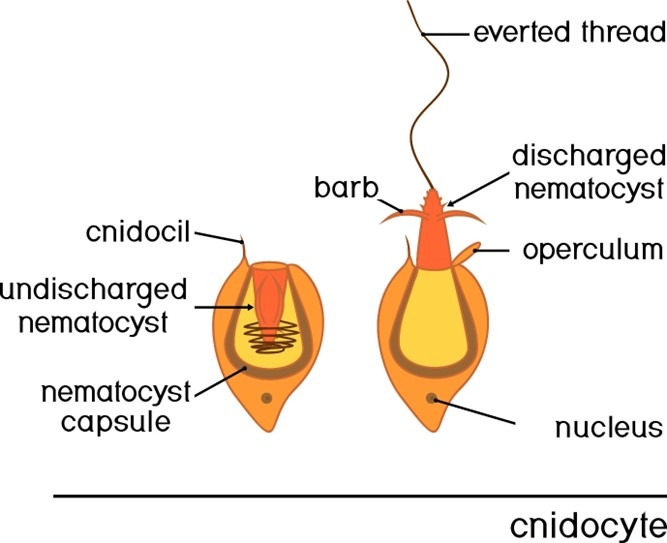
Fig 6. An illustration of a cnidocyte.
The chloroplasts of this clade quench surplus excitation energy which ceases photosynthesis and intraholobiont molecular exchange, which may trigger their expulsion (Slavov et al. 2016).
Hitherto clade D is now nested within Durusdinium with D. trenchii-like species with a genus that means “tough”. These extremophiles tolerate immoderations of temperature, irradiance and shade, that are regionalised in the Indo-West Pacific where their hosts become bleach-resistant (LaJeunesse et al. 2018).
Effrenium of clade E has merely the one nonsymbiotic bloom-forming species: E. voratum that peculiarly swims throughout the Calvin cycle, is large compared with other Symbiodiniaceae, and preys upon pro- and eu-karyotes using a peduncle basket (LaJeunesse et al. 2018) analogous to the cytopharyngeal tube of ciliates (Fig 7.). Their genus translates to “living unrestrained” where they remain common to the subtropical and temperate regions of the Pacific and Atlantic (LaJeunesse et al. 2018).
Fugacium which means “ephemeral” was formerly ranked within subclade Fr 5 with a species type of F. kawagutii. The ecological significance of these foram symbionts or free-living mastigotes is largely unexplored which may occur nominally in cnidarians, while subclades Fr 2 to Fr 4 remain yet-to-be-designated divergent lineages of clade F (LaJeunesse et al. 2018).
The uncommon genus Gerakladium which translates as “old” is a comparatively ancient previously described subclade of G comprising associates of multicellular organisms of the kingdom Metazoa, which was absorbed into the species type G. endoclionum. They have been isolated from boring sponges of the family Clionaidae from the Atlantic and Pacific or they have partnered with Pacific black corals of the subclass Hexacorallia and order Antipatharia, while their natural history remains largely enigmatic (Fig 8.). They are likely represented in benthos and phytoplankton that become negligeable and/or transitory residents of scleractinians (LaJeunesse et al. 2018).
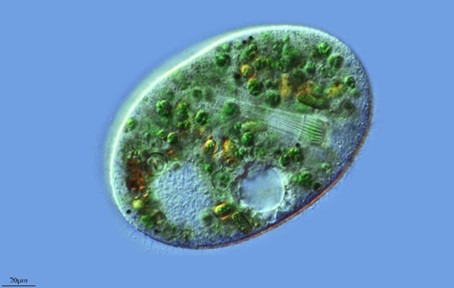
Fig 7. Nassula species (Ciliophora: Nassophorea) of chromist illustrating the cytopharyngeal tube lined with nematodesmata. Image courtesy of Antonio Guillén©, Proyecto Agua, https://www.Flikr.com/
Breviolum, Cladocopium, Durusdinium and Symbiodinium-like species are common symbionts of Scleractinia where the former species are suited to deeper habitats and the genera Fugacium and Gerakladium rarely occur (Boilard et al. 2020)
Microbial Populations
The phylogenetic data presented is informative yet it is unrepresentative of the microscopic communities of the coral holobiont. Here we revisit and combine information from part I, nevertheless the reader will gain maximum benefit from comprehension of that entire editorial. We know that microscopic photosynthetic autotrophic, heterotrophic, and mixotrophic prokaryotes and eukaryotes inhabit holobionts and holosystems and a downturn in heterogeneity is linked to dysfunction and disease. Coral-associated microbes include Bacteria, Cyanobacteria, Archaea, fungi, yeasts, endolithic algae, chromists, protozoa, viruses, and bacteriophage (Suchodolski 2012; Munn 2019) that differ in their susceptibility to extremes of temperature, dissolved oxygen (DO), irradiance, and nutrients yet we remain unaware of the gamut of their undiscovered physicochemical and biochemical frailties.
Population dynamics, toxicology, and pathology propose that members of a community continuously die and reproduce, and contaminant, condition, and trauma-survivorship contrasts inter- and intra-specifically and amongst strains and individuals. Apoptotic pathways also initiate preprogrammed cell death when cells are so old they cannot divide (Godar 1996; Zhang et al. 1999; Ainsworth et al. 2007), whereas some microbes enter viable but nonculturable (VBNC) stasis (Oliver & Bockian 1995; Sun et al. 2008; Colorni & Diamant 2014) or form impervious cysts, spores, and proliferate vegetatively and sexually. Organisms exploit all ecological niches where pathogen, stress, or apoptosis-derived cellular debris facilitates the disproportionate multiplication of a handful of opportunistic affiliates, where diversity is curtailed which expedites malfunction.
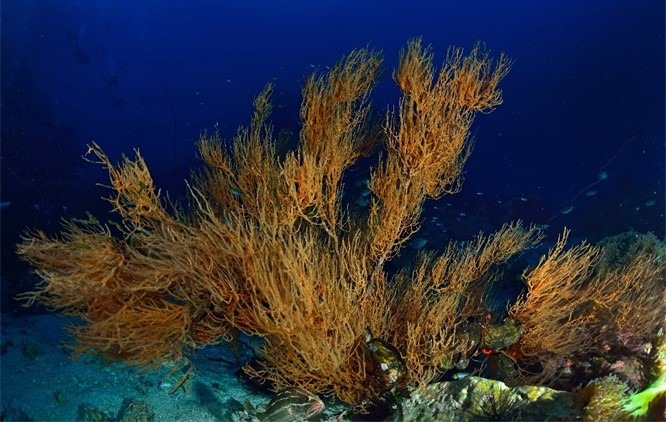
Fig 8. Black coral (Antipathes cf. grandis; Hexacorallia: Antipatharia).
Prokaryotic generations are brief and their mutation rate is elevated which makes them highly adaptable, whereas eukaryotes can exploit epigenetic manipulation and phenotypic plasticity (Gittins et al. 2015; Calixto-Botía & Sánchez 2017; Dubé et al. 2017; Putnam et al. 2017). Bacteria produce antimicrobial peptides (AMPs) and fungicides (Nizet et al. 2000; Lopez et al. 2001; Fuller et al. 2002; Shabir et al. 2018; Valero et al. 2019; Boilard et al. 2020), while phototrophs liberate biocidal ROS (Boilard et al. 2020), and fungi manufacture penicillins and antibiotics that kill neighbouring microbes yet many bacteria carry conjugatable antibiotic resistance genes. Several use autoinducers to communicate via quorum sensing to offer a coordinated response to their environment yet these signals can be quenched by others (Vattakaven et al. 2006; Sarjito et al. 2009; Tait et al. 2010; Haenen et al. 2014), whereas secretion systems can inject effector molecules into their neighbours, export microbe-digesting enzymes extracellularly, or express them on their cell-surface (Cherrak et al. 2019). Moreover viruses including those of bacteria (bacteriophage) can enter lytic or lysogenic phases where pathogenesis may instigate cellular lysis and/or degradative necrosis or arm the cell with a resilient genotype (Stencel-Baerenwald et al. 2014; Wagstaff et al. 2018a; Wagstaff et al. 2018b). Furthermore climate influences viral life cycles and the influx of vital nutrients (Send & Nam 2012). Cell lines are thus vulnerable where a single strain or species can experience overnight extinction. Therefore an extensive variety of nonidentical populations retains greater inertia and subsistence compared with a mere handful of clones.
The coral holobiont offers oxic, microoxic, anoxic, acid, alkaline, light, dark, eutrophic, mesotrophic, and oligotrophic environments which are likely reef zone and depth dependent, yet core communities of microorganisms occur in several if not most scleractinian genera (Hernandez-Agreda et al. 2018; Munn 2019).
Comparable populations were observed in analogous microbiomes from the same genus living within similar zones, where core holobiont affiliates performed beneficial roles (Ainsworth et al. 2015; van de Water et al. 2016; Hernandez-Agreda et al. 2017; Munn 2019).
Comparable populations were observed in analogous microbiomes from the same genus living within similar zones, where core holobiont affiliates performed beneficial roles (Ainsworth et al. 2015; van de Water et al. 2016; Hernandez-Agreda et al. 2017; Munn 2019).
The microbes are diverse, transient, and attuned to the prevailing conditions apart from a nominal population common to most hermatypes (Hernandez-Agreda et al. 2018).
The coral secretes nutrifying carbohydrates and proteins for its heterotrophs that reciprocate with co-factors, vitamins, and macronutrients (Munn 2019).
The microorganisms of the mucosal microbiome are the coral’s first line of defence, where multiplicity is once again key to maintaining an effective barrier like the mucosal lining of a fish’s gut. A network of molecular signals may warn the coral of reduced microbial diversity where gene expression determines colony recuperation or demise (Boilard et al. 2020; Santoro et al. 2021). Too replete to evaluate species and strains of zooxanthellae and microorganisms are continuously evolving where the former are multiplied, recruited, or purged, which guarantees the robust heterogeneous community within each coral optimises photosynthesis and endurance. Nonetheless sustained extremes of illumination and heat overwhelm all but the most resilient.
Anticipate reclassification of unspecified clades and species, whilst the molecular and empirical framework defined by LaJeunesse and collaborators continues to elucidate the phylogeny of these important dinoflagellates (LaJeunesse et al. 2018) where a loss of holobiont diversity results in coral disease and dysbiosis (Rosenberg et al. 2007; Rosado et al. 2018; Munn 2019; Pollock et al. 2019; Santoro et al. 2021) which is a microcosm of the malfunctioning holosystem of part I.
Next we discover the hidden richness and diversity of the symbiotic prokaryotes of the coral holobiont of the kingdoms Bacteria and Archaea in part III.
References
Ainsworth, D., T., Krause, L., Bridge, T., Torda, G., Raina, J., Zakrzewski, M., Gates, R., Padilla-Gamiño, J., Spalding, H., Smith, C., Woolsey, E., Bourne, D., Bongaerts, P., Hoegh-Guldberg, O. & Leggat, W. (2015) The coral core microbiome identifies rare bacterial taxa as ubiquitous endosymbionts. The ISME Journal. 9(10), 2261-2274.
Ainsworth, T., Kvennefors, E., Blackall, L., Fine, M. & Hoegh-Guldberg, O. (2007) Disease and cell death in White Syndrome of Acroporid corals on the Great Barrier Reef. Marine Biology. 151(1),.
Allemand, D., Ferrier-Pages, C., Furla, P., Houlbreque, F., Puverel, S., Reynaud, S., Tambutte, A., Tambutte, S. & Zoccola, D. (2004) Biomineralisation in reef-building corals: from molecular mechanisms to environmental control. Comptes Rendus Palevol. 3(6), 453-467.
Babcock, R., C. (1985) Growth and mortality in juvenile corals (Goniastrea, Platygyra and Acropora): the first year. In: Proceedings 5th Int. Coral Reef Congress, Tahiti. 4, 355-360.
Balling, H., W., Janse, M. & Sondervan, P. (2008) Trace elements, functions, sinks and replenishment in reef aquaria. Advances in coral husbandry in public aquariums. Leewis, R., J. & Janse, M. (eds.). Burgers’ Zoo, Arnhem, The Netherlands. pp 143-156.
Beckmann, A. & Özbek, S. (2012) The nematocyst: a molecular map of the cnidarian stinging organelle. The International journal of developmental biology. 56(6-8), 577-582.
Béraud, E., Gevaert, F., Rottier, C. & Ferrier-Pagès, C. (2013) The response of the scleractinian coral Turbinaria reniformis to thermal stress depends on the nitrogen status of the coral holobiont. The Journal of experimental biology. 216(14), 2665-2674.
Boch, C., A. & Morse, A., N., C. (2012) Testing the effectiveness of direct propagation techniques for coral restoration of Acropora spp. Ecological Engineering. 40, 11-17.
Boilard, A., Dubé, C., E., Gruet, C., Mercière, A., Hernandez-Agreda, A. & Derome, N. (2020) Defining Coral Bleaching as a Microbial Dysbiosis within the Coral Holobiont. Microorganisms. 8, 1682. doi:10.3390/microorganisms8111682
Calixto-Botía, I. & Sánchez, J., A. (2017) A case of modular phenotypic plasticity in the depth gradient for the gorgonian coral Antillogorgia bipinnata (Cnidaria: Octocorallia). BMC Evol Biol. 17, 55.
Cherrak, Y., Flaugnatti, N., Durand, E., Journet, L. & Cascales, E. (2019) Structure and Activity of the Type VI Secretion System. Microbiology spectrum, 7(4), 1.
Colorni, A. & Diamant, A. (2014) Infectious diseases of warmwater fish in marine and brackish waters. Diseases and Disorders of Finfish in Cage Culture: Second Edition. Woo, P., T., K. & Bruno, D., W. (eds.). Centre for Agriculture and Bioscience International, Wallingford, UK. pp 155-192.
Cunning, R. & Baker, A., C. (2013) Excess algal symbionts increase the susceptibility of reef corals to bleaching. Nature Climate Change. 3, 259-262.
Fautin, D. & Buddemeier, R. (2004) Adaptive bleaching: a general phenomenon. Hydrobiologia. 531(3), 459-467.
Ferrier‐Pagès, C., Godinot, C., D’Angelo, C., Wiedenmann, J. & Grover, R. (2016) Phosphorus metabolism of reef organisms with algal symbionts. Ecological Monographs. 86(3), 262-277.
Fuller, J., Camus, A., Duncan, C., Nizet, V., Bast, D., Thune, R., Low, D. & de Azavedo, J. (2002) Identification of a Streptolysin S-Associated Gene Cluster and Its Role in the Pathogenesis of Streptococcus iniae Disease. Infection and Immunity. 70(10), 5730.
Gates, R., D., Baghdasarian, G. & Muscatine, L. (1992) Temperature stress causes host cell detachment in symbiotic cnidarians: implications for coral bleach-ing. Biol Bull. 182, 324–332. https://doi.org/10.2307/1542252
Gittins, J., R., D’Angelo, C., Oswald, F., Edwards, R., J. & Wiedenmann, J. (2015) Fluorescent protein-mediated colour polymorphism in reef corals: multicopy genes extend the adaptation/acclimatization potential to variable light environments. Mol. Ecol. 24, 453-465.
Godar, D. (1996) Preprogrammed and Programmed Cell Death Mechanisms of Apoptosis: UV‐Induced Immediate and Delayed Apoptosis. Photochemistry & Photobiology. 63(6),.
Guiry, M., D. (2019) Dinophyta (Pyrrophyta): Dinoflagellates. The Seaweed Site: information on marine algae. http://www.seaweed.ie/algae/dinophyta.php
Haenen, O., van Zanten, E., Jansen, R., Roozenburg, I., Engelsma, M., Dijkstra, A., Boers, S., Voorbergen-Laarman, M. & Möller, A. (2014) Vibrio vulnificus outbreaks in Dutch eel farms since 1996: strain diversity and impact. Diseases of aquatic organisms. 108(3), 201-209.
Hernandez-Agreda, A., Gates, R. & Ainsworth, T. (2017) Defining the Core Microbiome in Corals’ Microbial Soup. Trends in microbiology. 25(2), 125-140.
Hernandez-Agreda, A., Leggat, W., Bongaerts, P., Herrera, C. & Ainsworth, T. (2018) Rethinking the Coral Microbiome: Simplicity Exists within a Diverse Microbial Biosphere. mBio. 9(5),.
LaJeunesse, T., C., Parkinson, J., E., Gabrielson, P., W., Jeong, H., J., Reimer, J., D., Voolstra, C., R. & Santos, S., R. (2018) Systematic Revision of Symbiodiniaceae Highlights the Antiquity and Diversity of Coral Endosymbionts. Curr Biol. 28, 2570-2580.
Levy, O., Dubinsky, Z. & Achituv, Y. (2003) Photobehavior of stony corals: responses to light spectra and intensity. Journal of Experimental Biology. 206, 4041-4049.
López, R., González, M., García, E., García, J. & García, P. (2001) Biological roles of two new murein hydrolases of Streptococcus pneumoniae representing examples of module shuffling. Research in microbiology. 151(6), 437-443.
McCauley, M., Goulet, T., L., Jackson, C., R. & Loesgen, S. (2022) Systematic review of cnidarian microbiomes reveals insights into the structure, specificity, and fidelity of marine associations. Nature Communications. 14, 4899. https://doi.org/10.1038/s41467-023-39876-6
Morse, D., E., Hooker, N., Morse, A., N., C. & Jensen, R., A. (1988) Control of larval metamorphosis and recruitment in sympatric agariciid corals. J. Exp. Mar. Biol. Ecol. 116,193-217.
Munn, C., B. (2019) Marine Microbiology: Ecology & Applications, Third Edition. Munn, C., B. (ed.). CRC Press, Taylor & Francis Group, London. pp 273-326.
Nielsen, D., A., Petrou, K. & Gates, R., D. (2018) Coral bleaching from a single cell perspective The ISME Journal. 12, 1558–1567. https://doi.org/10.1038/s41396-018-0080-6
Nizet, V., Beall, B., Bast, D., Datta, V., Kilburn, L., Low, D. & De Azavedo, J. (2000) Genetic Locus for Streptolysin S Production by Group A Streptococcus. Infection and Immunity. 68(7), 4245.
Oliver, J., D. & Bockian, R. (1995) In vivo resuscitation, and virulence towards mice, of viable but nonculturable cells of Vibrio vulnificus. Appl Environ Microbiol. 61, 2620-2623.
Putnam, H., Barott, K., Ainsworth, T. & Gates, R. (2017) The Vulnerability and Resilience of Reef-Building Corals. Current Biology. 27(11), 1551-1706.
Riddle, D. (2004) Feature Article: Playing With Poison – Ultraviolet Radiation. https://www.advancedaquarist.com/2004/8/aafeature
Riddle, D. (2014) Effects of Yellow Water on Light Transmission in an Aquarium – Why Should You Care. AdvancedAquarist.com. https://www.advancedaquarist.com/2014/1/aafeature
Rodriguez-Lanetty, M., Scaramuzzi, C., Quinnell, R. & Larkum, A. (2005) Transport of symbiotic zooxanthellae in mesogleal canals of Zoanthus robustus? Coral Reefs. 24, 195-196.
Rosado, P., Leite, D., Duarte, G., Chaloub, R., Jospin, G., Nunes da Rocha, U., P., Saraiva, J., Dini-Andreote, F., Eisen, J., Bourne, D. & Peixoto, R. (2018) Marine probiotics: increasing coral resistance to bleaching through microbiome manipulation. The ISME Journal. 13(4), 921-936.
Rosenberg, E., Koren, O., Reshef, L., Efrony, R. & Zilber-Rosenberg, I. (2007) The role of microorganisms in coral health, disease and evolution. Nature Reviews Microbiology. 5(5), 355-362.
Santoro, E., P., Borges, R., M., Espinoza, J., L., Freire, M., Messias, C., S., M., A., Villela, H., D., M., Pereira, L., M., Vilela, C., L., S., Rosado, J., G., Cardoso, P., M., Rosado, P., M., Assis, J., M., Duarte, G., A., S., Perna, G., Rosado, A., S., Macrae, A., Dupont, C., L., Nelson, K., E., Sweet, M., J., Voolstra, C., R. & Peixoto, R., S. (2021) Coral microbiome manipulation elicits metabolic and genetic restructuring to mitigate heat stress and evade mortality. Science advances. 7(33), 3088.
Sarjito, R., Radjasa, O., K., Sabdono, A., Prayitno, S., B. & Hutabarat, S. (2009) Phylogenetic diversity of the causative agents of vibriosis associated with groupers fish from Karimunjawa Islands, Indonesia Curr. Res. Bacteriol. 2, 14-21.
Sarras, M., P., Madden, M., E., Zhang, X., Gunwar, S., Huff, J., K. & Hudson, B., G. (1991) Extracellular matrix (mesoglea) of Hydra vulgaris: I. Isolation and characterization. Developmental Biology. 148(2), 481-494.
Send, U. & Nam, S. (2012) Relaxation from upwelling: The effect on dissolved oxygen on the continental shelf. J. Geophys. Res. 117, C04024.
Shabir, U., Ali, S., Magray, A., Ganai, B., Firdous, P., Hassan, T. & Nazir, R. (2018) Fish antimicrobial peptides (AMP’s) as essential and promising molecular therapeutic agents: A review. Microbial pathogenesis. 114, 50-56.
Sharp, K., Distel, D. & Paul, V. (2012) Diversity and dynamics of bacterial communities in early life stages of the Caribbean coral Porites astreoides. ISME J. 6, 790-801.
Shinzato, C., Shoguchi, E., Kawashima, T. et al. (2011) Using the Acropora digitifera genome to understand coral responses to environmental change. Nature. 476, 320-323.
Slavov, C., Schrameyer, V., Reus, M., Ralph, P., J., Hill, R., Büchel, C., Larkum, A., W., D. & Holzwarth, A., R. (2016) “Super-quenching” state protects Symbiodinium from thermal stress — Implications for coral bleaching. Biochimica et Biophysica Acta. 1857, 840–847.
Starcevic, A., Dunlap, W., C., Cullum, J., Shick, J., M., Hranueli, D. & Long, P., F. (2010) Gene expression in the scleractinian acropora microphthalma exposed to high solar irradiance reveals elements of photoprotection and coral bleaching. PLoS ONE. 12. 5(11), 13975.
Stencel-Baerenwald, J., Reiss, K., Reiter, D., Stehle, T. & Dermody, T. (2014) The sweet spot: defining virus–sialic acid interactions. Nature Reviews Microbiology. 12(11), 739-749.
Stryer, L. (1995a) Biochemistry 4th Edition. Stryer, L. (ed.). W. H. Freeman and Company, New York. pp 653-682.
Stryer, L. (1995b) Biochemistry 4th Edition. Stryer, L. (ed.). W. H. Freeman and Company, New York. p 888.
Suchodolski, J. (2012) Gastrointestinal Microbiota. Canine and Feline Gastroenterology. 32-41.
Sun, F., Chen, J., Zhong, L., Zhang, X., Wang, R., Guo, Q. & Dong, Y. (2008) Characterization and virulence retention of viable but nonculturable Vibrio harveyi. FEMS Microbiology Ecology. 64(1), 37-44.
Tait, K., Hutchison, Z., Thompson, F., L. & Munn, C., B. (2010) Quorum sensing signal production and inhibition by coral‐associated vibrios. Environmental Microbiology Reports. 2, 145-150.
Technau, U. & Steele, R., E. (2011) Evolutionary crossroads in developmental biology: Cnidaria. Development (Cambridge, England). 138(8), 1447-1458.
Thompson, J., Rivera, H., Medina, M. & Closek, C. (2015) Microbes in the coral holobiont: Partners through evolution, development, and ecological interactions. Frontiers in Cellular and Infection Microbiology. 4,.
Valero, Y., Saraiva‐Fraga, M., Costas, B. & Guardiola, F., A. (2019) Antimicrobial peptides from fish: beyond the fight against pathogens. Reviews in Aquaculture. 12, 224-253.
van de Water, J., Melkonian, R., Junca, H., Voolstra, C., Reynaud, S., Allemand, D. & Ferrier-Pagès, C. (2016) Spirochaetes dominate the microbial community associated with the red coral Corallium rubrum on a broad geographic scale. Scientific Reports. 6,.
Vattakaven, T., Bond, P., Bradley, G. & Munn, C., B. (2006) Differential Effects of Temperature and Starvation on Induction of the Viable-but-Nonculturable State in the Coral Pathogens Vibrio shiloi and Vibrio tasmaniensis. Applied and Environmental Microbiology. 72(10), 6508-6513.
Waggoner, B., M., Collins, A. & Smith D. (1994) Introduction to the Anthozoa. Berkeley University of California Museum of Palaeontology. UCMP.Berkeley.edu. https://ucmp.berkeley.edu/cnidaria/anthozoa.html
Wagstaff, B., Hems, E., Rejzek, M., Pratscher, J., Brooks, E., Kuhaudomlarp, S., O’Neill, E., Donaldson, M., Lane, S., Currie, J., Hindes, A., Malin, G., Murrell, J. & Field, R. (2018a) Insights into toxic Prymnesium parvum blooms: the role of sugars and algal viruses. Biochemical Society transactions. 46(2), 413-421.
Wagstaff, B., Rejzek, M. & Field, R. (2018b) Identification of a Kdn biosynthesis pathway in the haptophyte Prymnesium parvum suggests widespread sialic acid biosynthesis among microalgae. The Journal of biological chemistry. 293(42), 16277-16290.
WoRMS (2021) World Register of Marine Species. Dinophyceae. https://www.marinespecies.org/aphia.php?p=taxdetails&id=19542
Xu, M., Cheng, K., Xiao, B., Tong, M., Cai, Z., Jong, M-C., Chen, G. & Zhou, J. (2023) Bacterial Communities Vary from Different Scleractinian Coral Species and between Bleached and Non-Bleached Corals. Microbiology Spe ctrum. 11(3),.
Yu, J., Liao, T., Chen, C., Liao, T., Fang, L. & Tsai, W. (2000) Do color patterns of Pocillopora damicornis reflect Zooxanthellae diversity? Coral Reefs. 19(1), 98-99.
Zhang, X., Mar, V., Zhou, W., Harrington, L. & Robinson, M., O. (1999) Telomere shortening and apoptosis in telomerase-inhibited human tumor cells. Genes & development. 13(18), 2388-2399
Zoccola, D., Ganot, P., Bertucci, A., Geminti-Segonds, N., Techer, N., Voolstra, C., R., Aranda, M., Tambutte, E., Allemand, D., Casey, J., R. & Tambutte, S. (2015) Bicarbonate transporters in corals point towards a key step in the evolution of cnidarian calcification. Scientific Reports. 5, 9983
0 Comments