Speaking at the 1998 Society of Integrative and Comparative Biology annual meeting, Dr. Bruce Carlson outlined the Waikiki Aquarium’s success raising Acropora and other stony corals. The room full of professional coral reef scientists listened intently as Dr. Carlson explained that reef hobbyists were having the same success growing corals in their homes. After the meeting Dr. Carlson was quickly surrounded by a group of scientists who wanted to learn more about how we were able to keep corals alive for years, a feat few scientists had accomplished. The wonderment expressed by these scientists has been repeated many times since then. Invariably, coral reef scientists when seeing a reef tank for the first time are shocked and surprised by our success. They realize that we “civilians” have been accomplishing more in the area of coral husbandry than professionals in the field have. In that regard, the professionals appreciate even more then the
hobby does, what we have accomplished. Clearly it doesn’t take a PhD to create a successful reef tank, and a PhD does not guarantee success in the hobby.
One reason we don’t appreciate our success as much as we should is that we seem always to be just one step ahead of disaster. Whether it is the loss of a single prized animal or a total tank crash, we all experience our own share of personal failures. As much as any other emotion, the specter of failure seems to have driven the hobby’s advance over the past decade. How else can we explain the all too common act of successful hobbyists changing much of what they do because other hobbyists claimed success doing something completely different.
A reef tank is a complicated system of mechanical and biological components. There are a lot of moving parts. Disasters can generally be attributed to the failure of one or more of these parts. Whether it is the catastrophic failure of a powerhead, the inadvertent dosing of large amounts of kalkwasser, the unnoticed death of a tank inhabitant polluting the tank, or any other of a virtually limitless number of possible problems, the root cause of a problem can generally be identified. There are those events, however, that defy explanation. Inexplicable deaths where the animal is behaving normally one day and is dead the next. Old Tank Syndrome (OTS), a phenomenon observed many time whereby a consistently maintained tank thrives for years and then gradually deteriorates and perhaps even crashes. These are the most disturbing failures we face, because we have no explanation for them. We don’t know what we are doing wrong, so we have no way of determining what we should do differently.
Uncertainty creates vulnerability, and too often there are those that play on our fears as a means to advance agendas. Living as hobbyists do just one step ahead of disaster, too many are all too ready to blindly accept solutions that promise to pull us just one single step back from the precipice. Anyone who proposes a possible explanation and solution to these problems finds an attentive and accepting audience. The truth is, however, that these problems may be intractable. We may never determine a root cause of problems like OTS. There may not even be a single problem but instead a combination of factors. That’s why we should be wary of any simple explanation that claims to address a whole host of problems. We should critically examining the proposed explanation. What evidence supports the explanation? How strong is the evidence?
This third in a series of articles is a reexamination of one hobbyist’s view that many of the inexplicable problems we experience with reef tanks are caused by an accumulation of toxic heavy metals. “I think that the cause of many such unexplained mortalities lies hidden in our tanks, and is even abetted or accentuated by well-meaning aquarists. My hypothesis is that a primary cause of many, if not all, of these unexplained mortalities is heavy metal poisoning. I believe this metal poisoning is specifically due to excessively high concentrations of some of the trace elements that are present in tank water.” (Shimek 2002) While the author suggests that heavy metal poisoning is only a hypothesis, in an on-line exchange, he claimed that it was much more than a hypothesis. Shortly after this quote was published, the author responded to a questioner in this way: “You may consider the idea of tank mortality due to chemical build up as a theory. It isn’t. I have collected the necessary data, and I have done the necessary tests to show that it is a proven fact. Over the next month or so, additionally I will be doing some direct sea-urchin larval bioassays on various salt mixes to put the last nails in that coffin.” So even before conducting further study, the author considered the data on artificial seawater toxicity a “proven fact.”
Writing in Marine Pollution Bulletin, the editors decried the decline scientific objectivity. They wrote that, “It is both wrong and dangerous for a scientist to become personally and inflexibly ‘attached’ to a theory….Becoming inflexibly attached to a theory, whether or not a scientist considers it his or her own, prevents that scientist from thinking of more useful theories.” (Chapman & Giddings 1997) As we continue this reexamination, readers should keep the words of Chapman and Giddings in mind. The hobby benefits most by viewing any proposed explanation for inexplicable phenomena with some skepticism. As we will show, despite the author’s confidence, his hypothesis is far from proved, and his conclusions far from fact.
If It’s In The Water, Is It Toxic?
To prove as the author asserts that high concentrations of some heavy metals cause unexplained mortalities in a reef tank, we need to prove that 1) heavy metal levels in the average reef tank significantly exceed levels found in natural seawater, 2) that these elevated metals in the concentrations found are toxic, and 3) that the toxicity “explains” unexplained deaths. If any part of this three part hypothesis is proved false, then we have to reject the hypothesis. Unfortunately, the third element is virtually impossible to prove short of necropsies of every organism that dies in a reef tank. To prove that toxic levels of any substance killed an organism, we have to test for the suspected substances. Clearly, this is impractical. Consequently, by the rules of hypothesis testing we have to reject the hypothesis even without examining the first two elements of the hypothesis. Nevertheless, the first two elements are intriguing enough to warrant this reexamination of the author’s
“proof.”
In part 2 of this series we showed that an ICP scan analysis of natural coral reef waters found that heavy metal concentrations in the collected water were considerably higher than published seawater data. (Harker 2004) More significantly, the analysis found levels of heavy metals in coral reef water to be very similar to the levels of metals in my own reef tanks and only slightly lower than those reported earlier for reef tanks. Perhaps natural coral reef seawater has elevated metal levels compared to the open ocean. Perhaps the complex nature of seawater prevents an ICP scan from providing an accurate view of metal content of seawater. In either case, we have insufficient evidence to conclude that reef tanks have elevated heavy metal levels. We therefore have to reject the first element of the author’s proposed hypothesis, that the average reef tank has concentrations of heavy metals much higher than those found in natural seawater.
Comparing the levels of metals in coral reef water to the levels deemed toxic according to Shimek in, “Our Coral Reef Aquaria-Our Own Personal Experiment in the Effects of Trace Element Toxicity,” one might conclude that natural coral reef water itself is toxic. (Shimek 2002) The author presents a litany of toxicity studies that show harmful effects of metals at levels lower than I reported for natural coral reef water. How can this be? It is because not all metal in seawater is toxic. In one toxicity study, the authors remind us that, “contaminants are only toxic if they are incorporated into an organism’s tissues.” (Hook & Fisher 2000) To be toxic, a metal must be bioavailable, available in a form that can be absorbed into the tissue of the organism. (Meyer 2002) The author writes, “The total concentration of a metal in the water column is not a good predictor of acute toxicity. Because of the presence of modifying factors (dissolved and particulate), the potential for the
metal to interact with biotic ligands often will be less than that indicated by the total metal concentration.” A recent Advanced Aquarist article explained that potentially toxic elements exist in many compounds, few of which are toxic. (Sekha 2003) Only a portion of metals found in seawater are bioavailable. Much of the metal found in seawater exists in a form that is not readily available to organisms, and is therefore not toxic.
The partitioning between the different forms of a metal is called speciation. Generally speaking, there are three dissolved forms of a metal. Metals can be present as a free hydrated ion, in inorganic complexes, or in organic complexes. (Valasquez, et al., 2002) The free ion form is considered bioavailable while the organic and inorganic complexes are generally considered not bioavailable. (Sundra & Guillard 1976) In addition to these dissolved forms, metals can be precipitated or sorbed onto solid surfaces, particularly carbonate surfaces. (Mwanuzi & De Smedt 1999) Since the ionic state is the only toxic form of a metal we need be concerned with, it is the ionic component that we need to measure. Unfortunately, the ICP scan cannot differentiate between the different forms of a metal. It only tells us the total of the combined ionic, inorganic and organic complexes. While there are analytical methods that can determine the speciation of heavy metals in seawater, these tests
have not been done on reef tanks.
As a rule the toxic ionic proportion of a metal in seawater is generally low. Most heavy metals in seawater are organically or inorganically bound, and are therefore non-toxic. Depending on the study, ionic metals can range from 20-30% of total dissolved metals. That means that 70-80% of the concentration of heavy metals in seawater is not in a form that is toxic to reef tank inhabitants. That does not mean the metals are rendered permanently non-toxic, only that at the time of analysis, they were non-toxic. Any hobbyist who has dosed a tank with copper in an effort to rid fish of parasites has experienced this effect first hand. It is very difficult to maintain a constant level of ionic copper in a tank, particularly if the tank has sand or live rock. While the level of total copper remains unchanged, testing for copper will show that levels are declining. Hobbyist copper tests measure ionic copper. As copper complexes with organic compounds and is sorbed onto calcium carbonate,
the proportion of ionic copper declines as does the toxicity of the copper. That is the case with all metals that enter a reef tank.
What About Bioassays?
According to the author, the “last nail in the coffin” regarding toxicity of reef tank water was a bioassay of sea-urchins. (Shimek 2003) Inexplicably, however, the bioassay primarily evaluated “freshly mixed artificial seawater,” not reef tank water, so the study ended up shedding far less light on the issue of reef tank toxicity than if water from reef tanks had been used. A bioassay subject an organism to successively higher levels of a toxin to determine the level at which the animal becomes impaired or dies. Heavy metal bioassays for seawater organisms generally use metal compounds that dissociate in seawater. Adding copper sulfide or copper chloride to seawater releases controlled amounts of ionic metals in the water. Consequently, virtually all of the metal is bioavailable. When trying to relate lethal levels of metals in a bioassay to the levels of metals in a reef tank, it is important to keep in mind that virtually all of the metal in a bioassay is toxic whereas only a portion of the measurable metal in a reef tank is toxic. This is why the findings of published bioassays are of limited value for hobbyists.
Many delicate animals readily reproduce in a mature reef tank. The female Amphipod is carrying a cluster of eggs in her abdomen. The polychaete larvae is less than 0.1 mm long.
In part 1 of this reexamination, I mentioned that it is important to study the “methods” section of any article to determine whether the methods used are proper and able to accomplish the intended goal of the study. One way to do this is to read related scientific literature to see if the author followed accepted methods. Fortunately toxicity research is well funded, and the scientific literature abounds with examples. Perhaps the most interesting was a study examining the effects of copper on the larvae of a decopod. (Wong, et al., 1995). The authors used Instant Ocean artificial seawater as the control medium. The authors stated, “Artificial seawater have been used extensively in our laboratory for culturing Metapenaeus ensis larvae and postlarvae and have shown no adverse effects on growth and development.” When a team of professional scientists publishing studies in a well respected journal like Marine Pollution Bulletin use Instant Ocean to raise larvae, one should be skeptical about an assertion that artificial seawater is toxic.
In another bioassay paper, the authors used Forty Fathoms Biocrystals. (Rumbold & Snedaker 1997) The authors note that the artificial seawater was aged with vigorous aeration for more than 48 hours and filtered prior to use. This provides one clue as to why professional researchers would be comfortable using artificial seawater that a hobbyist author has labeled toxic. Bioassays do not use “freshly made” artificial seawater. Scientists go to great lengths to prepare control water that can support the health of the organism. If natural seawater is used, typically the water is prefiltered and then pH and salinity adjusted before use. If artificial seawater is used, the water is aged for some time and then prefiltered before use. For decades, research scientists have emphasized the importance of aging artificial seawater. (Bidwell & Spotte 1985). The Gosse formula for artificial seawater dating from 1854 as reported in Bidwell & Spotte recommends filtering the solution
through sponge and conditioning by adding, “a few clean shore pebbles and some fronds of green seaweed.” Gosse recommended adding animals after one week. One hundred and fifty years ago scientists appreciated the importance of aging artificial seawater, so it is not clear why a hobbyist author would not follow well accepted methods in preparing his samples. Freshly made artificial seawater is inherently toxic, but becomes less toxic as it ages. That’s why since 1854 researchers have aged their artificial seawater. Conducting a bioassay on freshly prepared artificial seawater may determine how quickly one salt dissolves compared to others, but it tells us nothing about whether any of the salt mixes are toxic after a reasonable length of time.
Fortunately the bioassay also included water from two hobbyist reef tanks, so the results from these two tanks can offer some insights. The two hobbyists both used Instant Ocean. One prepares his water with RO/DI water while the other uses well water. We have one controlled variable, the salt mix, Instant Ocean. We have one known variable, the source water, RO/DI versus well water. The bioassay found that on average five times as many larvae survived in the water of the hobbyist using RO/DI water compared to the water made from well water. Since both hobbyists used Instant Ocean and there was a statistically significant difference between the two tanks, we have to eliminate the salt mix as a possible explanation for the difference.
Both hobbyists used Instant Ocean and yet only one was highly toxic to sea- urchin larvae. Why? We don’t know. Nothing in the study indicates that the waters were chemically analyzed. In the absence of metal analyses for the two tanks we cannot determine whether metal levels had anything to do with the outcome. Perhaps the well water contained higher levels of metals than the RO/DI water. Perhaps pesticides had contaminated the well water. Perhaps something unrelated to well water was the cause. In any case, we can eliminate the salt mix as the source of the problem. Despite the author’s confidence, the study falls well short of being the last nail in the debate’s coffin. Furthermore, since the majority of larvae in the one hobbyist’s water survived, it raises additional questions about whether reef tanks are really as toxic as they have been portrayed.
So What Is A Toxic Level?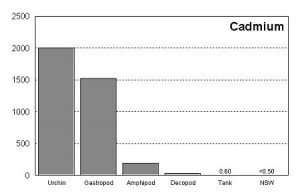
These graphs summarize the results of multiple bioassay studies. For nearly all of the metals studied, levels found in typical reef tanks are significantly lower than those found to be toxic to the organisms studied. See the references cited at the end of the article for complete details.
The majority of data on toxicity are based on bioassays where virtually all of the metal in solution is bioavailable. Since much of the heavy metal in our reef tanks is not bioavailable and therefore benign, it would be incorrect to conclude that a reef tank is toxic if metal levels are found to be at levels found to be toxic in a bioassay. Quite the contrary, we know that the majority of metals in seawater are not bioavailable. As a result, if a reef tank has a total metal concentration equal to that found to be toxic, it is safe to assume that the reef tank is not toxic. Furthermore, organisms have little difficulty tolerating metal concentrations just slightly below the published numbers. Unfortunately, we have no convenient means to determine what proportion of metals in a reef tank are bioavailable, so we may want to start by assuming that 100% of the metals are bioavailable and compare these numbers to the bioassay numbers. This is a highly unlikely assumption, but it provides a tremendous margin of error.
Select findings of several toxicity studies were reported by Shimek, and present a rather dire image of reef tanks. With regard to copper, the author writes, “(copper’s) effects on corals are profound and occur at concentrations below those typically found in aquaria.” (Shimek 2003) Reading the complete metal toxicity scientific literature reveals quite a different picture. Natural coral reef water was found to have 14 μg/l of copper. Typically bioassay results are presented in terms of LC50, the concentration that leads to 50% mortality over a stated period of time or IC50, the concentration that causes inhibition of some physiological function. Note that at the published LC50 levels, 50% of the organisms survive. One study found that 17.4 μg/l copper inhibited Acropora millepora fertilization, and 110 μg/l inhibited larval metamorphosis. (Negri, et al., 2001) In another study looking at Acropora tenuis, the authors found that 17.3 μg/l of copper did not significantly inhibit larvae settlement. (Reichelet-Brushett 2000). “However, concentrations of 42 μg/l and 80.5 μg/l results in significantly lower mean total settlement compared with the controls.” A study of Porites lutea found that 10 μg/l of copper had no effect on respiration, but 30 μg/l had a significant effect. (Alutoin, et al., 2001) In a study on gastropods, copper levels below 50 μg/l were safely tolerated by Nassarius festivus. (Cheng et al., 2002) In another study, median lethal concentrations for copper were 46μg/l for lobster larvae, 50 μg/l for spider crab larvae, and 3,304 μg/l for shrimp larvae. (Marino-Balsa, et al., 2000) Total copper in my tank measures 9.5 μg/l, the average of the 23 tanks tested was 24 μg/l, and the highest level of copper found was 38 μg/l. It is clear from these studies that the total copper levels typically found in a reef tank are quite safe for the tank’s inhabitants. And considering that the bioavailable proportion of
copper is a small fraction of the total level, the typical reef tank is far from toxic.
This is true for all the other potentially lethal metals. Metal levels in the average reef tank are well below toxic levels-even when we consider the total concentration of metals, not just the bioavailable portion. This should not be surprising. Copepods, amphipods, nematodes, and polychaetes readily reproduce in most mature reef tanks. Larvae are the most sensitive organisms in our tanks, and they survive quite well.
A Lurking Catastrophe?
It has been suggested that even if most metals in a reef tank are not generally bioavailable, that the accumulation of metals can lead to disaster if unexpected events suddenly make them bioavailable. A typical senario is a decline of pH. If the tank pH declines, a portion of the sand will dissolve and the metals adsorbed on the calcium carbonate might be released resulting in a lethal spike of metals. While this might be theoretically possible, what is the likelihood? We know that metals can be chemically bound to both organic and inorganic compounds. We also know that a relatively small proportion of ionic metals exist in seawater because of the propensity for metals to bind with organic and inorganic compounds. What is the likelihood that a high proportion of the bound metals might be released and made toxic?
We can gain some perspective by examining the laboratory procedure for reducing metals to their ionic state. To determine total metal content, the EPA requires that samples be reduced to a pH of less than 2 and then boiled in hydrochloric acid. Strong acids and an extremely low pH will release all bound metals, but these conditions are so removed from anything that a reef tank might encounter that we can safely rule out any similar release of metals in a reef tank. More likely in a reef tank with low pH is a slow release from the sand. However, a reef tank with low pH is generally one with a high organic load, so as the metals are released from the sand, they would most likely bind to organic compounds.
Conclusions
After reviewing the scientific literature and reviewing the series of hobbyist articles on metal toxicity, it is clear that evidence supporting metal poisoning as a source of inexplicable deaths in a reef tank is far from conclusive. Metal levels in the average reef tank are not significantly elevated, and the levels are below toxic levels in the scientific literature. A bioassay of reef tank water supports this conclusion. Artificial seawater made from Instant Ocean supported sea-urchin larvae survivability despite the fact that the author had previously identified Instant Ocean as one of the most heavy metal laden salt he examined.
As Chapman and Giddings wrote in their editorial, “We realize that theories and ideas require a great deal of effort, sometimes over many years. In this light, new ideas or criticisms which may not appear to be fully justified can be upsetting, and it is tempting to provide an abrupt, dismissive, or even hostile reply….Valid criticism can, at best, only temporarily be deflected. If a theory has serious flaws (or even subtle ones), they will show up eventually. Sooner or later the theory will crack under the weight of conflicting evidence. Persuasive arguments, rhetoric, and personal attacks on critics won’t protect a flawed theory forever.” Clearly the theory that much of what befalls a hobbyist is a result of heavy metal poisoning falls into this category. It is a flawed theory that cracks under the weight of conflicting evidence. The evidence presented in this series shows that it is possible to have heavy metal concentrations near that of coral reef water and well below levels
that have been shown to be toxic.
References
- Alutoin, S., J. Boberg, M. Nystrom, M. Tedengren. 2001. Effects of the multiple stressors copper and reduced salinity on the metabolism of the hermatypic coral Porities lutea. Marine Environmental Research 52:289-299.
- Bidwell, J.P., S. Spotte. 1985. Artificial Seawater Formulas and Methods. Jones & Bartlett Publishers, Boston MA.
- Chapman, P.M., J.M. Giddings. 1997. Scientists need good manners to be scientists. Marine Pollution Bulletin 34:852.
- Cheung, S.G., K.K. Tai, C.K. Leung, Y.M. Siu. 2002. Effects of heavy metals on the survival and feeding behavior of the sandy shore scavenging gastropod Nassarius festivus. Marine Pollution Bulletin 45:107-113.
- Gilbert, A.L. and Hector M. Guzman. 2001. Bioindication potential of carbonic anhydrase activity in anemones and corals. Marine Pollution Bulletin 42:742-744.
- Gustafsson, O., P.M. Gschwend. 1997. Aquatic colloids: concepts, definitions, and current challenges. Limnology and Oceanography 42:519-528
- Harker, R. 2004. Is it really in the water? Advanced Aquarist Volume 3 number 1.
- Hook, S.E., N.S. Fisher. 2001. Reproductive toxicity of metals in calanoid copepods. Marine Biology 138:1131-1140.
- Izquierdo, C., J. Usero, I. Gracia. 1997. Speciation of heavy metals in sediments from salt marshes on the southern Atlantic Coast of Spain. Marine Pollution Bulletin 34:123-128.
- King, C.K., M.J. Riddle. 2001. Effects of metal contaminants on the development of the common Antarctic sea urchin Sterechinus neumayeri and comparisons of sensitivity with tropical and temperate echinoids. Marine Ecology Progress Series 215:143-154.
- Lam, M.H., A.Y. Tjia, C. Chan, W. Chan, W. Lee. 1997. Speciation study of chromium, copper, and nickel in coastal estuarine sediments polluted by domestic and industrial effluents. Marine Pollution Bulletin 34:949-959.
- Lee, R.F., Kristen O’Malley, Yugi Oshima. 1996. Effects of toxicants on developing oocytes and embryos of the blue crab, Callinectest sapidus. Marine Environmental Research 42:125-128.
- Marino-Balsa, J.C., E. Poza, E. Vazquez, R. Beiras. 2000. Comparative toxicity of dissolved metals to early larval stages of Palaemon serratus, Maja squinado, Homarus gammarus. Archives of Environmental Contamination and Toxicology 39:345-351.
- Meyer. J.S. 2002. The utility of the terms “bioavailability” and “bioavailable fraction” for metals. Marine Environmental Research 53:417-423.
- Mwanuzi, F., F. De Smedt. 1999. Heavy metal distribution model under estuarine mixing. Hydrological Processes 13:789-804.
- Reichelt-Brushett, A.J. and P.L. Harrison. 2000. The effect of copper on the settlement success of larvae from the scleractinian coral Acropora tennis. Marine Pollution Bulletin 41:385-391.
- Roux, L.L., S. Le Roux, P. Appriou. 1998. Behavior and speciation of metallic species Cu, Cd, Mn, Fe during estuarine mixing. Marine Pollution Bulletin 36:56-64.
- Rumbold, D.G., S.C. Snedaker. 1997. Evaluation of bioassays to monitor surface microlayer toxicity in tropical marine waters. Archives of Environmental Contamination and Toxicology 32:135-140.
- Sekha, H. 2003. Toxicity of trace elements: truth or myth. Advanced Aquarist Volume 2 issue 5 may 2003.
- Shimek, R.L. 2003. The toxicity of some freshly mixed artificial seawater. Reefkeeping.com March 2003.
- Shimek, R.L. 2002. Our coral reef aquaria-Our own personal experiments in the effects of trace element toxicity. Reefkeeping.com August 2002.
- Valdovinos, C., M. Zuniga. 2002. Copper acute toxicity tests with the sand crab Emerita analoga: A biomonitor of heavy metal pollution in Chilean coastal seawater. Bulletin of Environmental Contamination and Toxicology 69:393-400.
- Velasquez, I.B., G.S. Jacinto, F.S. Valera. 2002. The speciation of dissolved copper, cadmium, and zinc in the Manila Bay, Philippines. Marine Pollution Bulletin 45:210-217.
- Wen, L., P. Santschi, G. Gill, C. Paternostro. 1999. Estuarine trace metal distribution in Galveston Bay. Marine Chemistry 63:185-212.
- Wong, C.K., J.K.Y. Cheung, K.H. Chu. 1995. Effects of copper on survival, development, and growth of Metapenaeus ensis larvae and postlarvae. Marine Pollution Bulletin 31:416-419
0 Comments