One of the most oft-discussed – if not the single most commonly mentioned – elements in the home aquarium is nitrogen. Although far from being the only element of interest to the typical reef aquarist, nitrogen is among the first elements the burgeoning aquarist learns about, and the canonical nitrogen cycle often lies at or near the center of most aquarium husbandry techniques. This attention is well deserved, of course, for the various nitrogen compounds, nitrate (NO3) especially, can have a controlling impact on the function of both natural and home reef ecosystems. Of particular interest to most reef hobbyists is the effect that nitrate concentrations can have on algae growth, with many hobbyists focusing on controlling nitrate concentration as a way to limit the growth of nuisance microalgae. On a global scale, nitrogen is often considered the limiting nutrient controlling primary productivity, and although many home aquaria may deviate from this rule, nitrate control remains one of the single most effective ways to influence primary productivity in our own systems.
Despite the importance of nitrogen in the home aquarium, among many aquarists there exists only a basic understanding of the dynamics that control the speciation and concentration of nitrogen. For many aquarists, our knowledge of the nitrogen cycle consists simply of the oxidation of ammonium (NH4) to nitrate and then reduction to nitrogen gas (N2). Although this is not an inaccurate understanding of aquarium nitrogen dynamics, what it fails to capture is the intricacy of the nitrogen cycle, especially in terms of how it is influenced by additional factors within our aquaria. Many of these additional factors may seem relatively unimportant at first glance, but I will argue that understanding them and the effect they have on nitrogen cycling can not only yield insight into how our systems work, but also make us better aquarists.
In this paper, I hope to shed light on to the home aquarium nitrogen cycle while providing additional insight into the factors that control the speed and magnitude of these processes. I will focus especially on factors that control the rates of denitrification, given the importance of this process as a sink for the removal of nitrogen from the system. In so doing, I will also present the relationship the nitrogen cycle has with the sand bed in an aquarium, as well as the twin concepts of bioirrigation and bioturbation that I argue are critical considerations when designing a natural and healthy reef aquarium. Finally, I will discuss the importance of carbon in this whole picture, with practical suggestions on ways to remove persistent high levels of nitrate and the broader implications for the aquarium ecosystem.
The Nitrogen Cycle
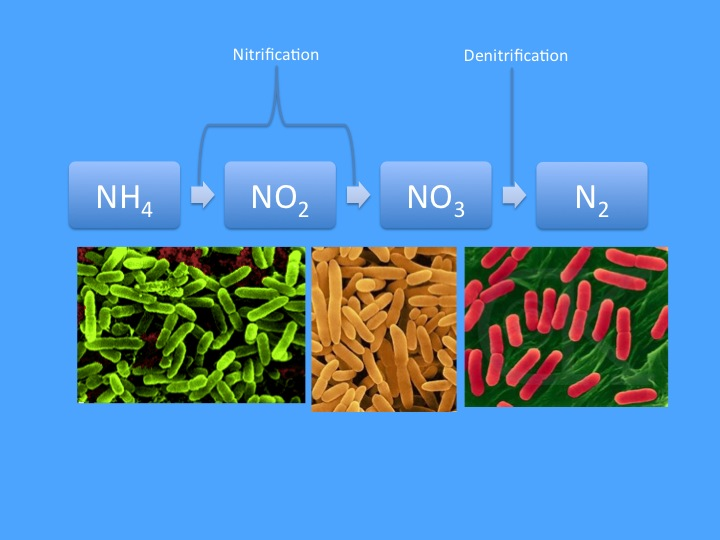
Figure 1: The classical nitrogen cycle as known by most aquarists consists of ammonium being oxidized by two groups of bacteria (Nitrosomonas and Nitrobacter are pictured) into nitrate, which is then reduced by a number of different species (Azomonas is pictured) into nitrogen gas, which then bubbles out of the aquarium.
In its simplest form (presented below in figure 1), the nitrogen cycle is taught as a simple linear progression from ammonia (NH3), which is oxidized to nitrite (NO2) and then nitrate, which is then reduced to nitrogen gas, (N2) which bubbles out of the aquarium and acts as a sink for the removal of nitrogen. The last step occurs under anoxic conditions (i.e. in areas where oxygen has been depleted), but other than that requirement the activity of the nitrogen cycle is essentially a given. While this is certainly only a basic understanding of complicated marine nitrogen dynamics, for the vast majority of home aquarists this simple concept has proven sufficient for typical husbandry practices. The whole picture, however, is more detailed, and in truth can not be considered in isolation from other elements; for instance, the basic premise that denitrification occurs in anoxic zones implies that we must also consider oxygen dynamics in the aquarium. Furthermore, in addition to transitions between the dissolved inorganic nitrogen (DIN) species listed above, we must also consider the organic sources and potential organic sinks of nitrogen.
Sources of Nitrogen
One of the main sources of nitrogen in the home aquarium is in the form of organic nitrogen, either particulate organic nitrogen (PON) or dissolved organic nitrogen (DON). The sole difference between those two sources is whether the organic substance has been broken down from large solid particles such as proteins to smaller dissolved ones such as amino acids. For our purposes we can consider both groups to be essentially interchangeable; not only is the conversion from PON to DON relatively fast, but also PON can be directly acted upon by the microbes responsible for nitrogen cycling (Kristensen et al. 1987, Berg et al. 2003).
Organic nitrogen can enter the aquarium in a number of ways. Every commonly available food (whether for fish, invertebrates, or the entire tank) contains large amounts of proteins, amino acids, etc. which contribute to this pool. While some of this food directly and immediately enters the nitrogen cycle, most enters as digestion by-products in the form of organic waste (or whole cells in the case of microbes). These wastes are then broken down in the process of diagenesis, of which the nitrogen cycle is a part and which will be discussed below.
In addition to direct input in the form of food, organic nitrogen can also enter the system as a product of nitrogen fixation. Nitrogen fixation is the process whereby a select group of organisms (collectively called diazatrophs) convert nitrogen gas into ammonium, which is then usually used by the nitrogen-fixing organism to support its own growth. Of course, when the organism dies this nitrogen enters the system, making this a crucially important source of N. Nitrogen fixation is a globally very important process that controls production in many ocean systems (Gruber et al. 1997), but unfortunately it is currently unknown to what extent N-fixation occurs in the home aquarium. The process is known to occur on coral reefs (Larkum et al. 1988), but it is not known whether cyanobacteria in the home aquarium undertake the process under normal conditions. Anecdotally, I have personally observed heterocysts (the N-fixing “organ” in these cyanobacteria) in microscopic samples obtained from my own aquarium, so it is a safe assumption that N-fixation is a source of nitrogen in the aquarium.
Once it has entered the system in the form of either PON or DON, nitrogen in the home aquarium is invariably consumed in some form or another, which will be discussed below. In nature, a significant chunk of PON is buried, but our aquaria lack both the sedimentation rate and thick sediment beds necessary for burial. Instead, all organic nitrogen in our systems will undergo the processes called diagenesis, which is the breakdown and eventual fate of organic material.
Remineraliztion and nitrification
Compounds that contain organic nitrogen – as a rule – also contain organic carbon, collectively termed particulate or dissolved organic carbon (POC or DOC). Although all heterotrophic organisms feed on these compounds (and many autotrophs need at least some of their components), the cellular machinery can work only with the small building blocks that make them up, such as amino acids. So, the first step in diagenesis is the breakdown of large, complex organic molecules into their building blocks, the process called remineralization, the general form of which is:
(CH2O)x(NH3)y + xTER + yH+ –> xCO2 + yNH4+ + xH2O
Where I have used “TER” to indicate the terminal electron acceptor and x and y are subscripts indicating the makeup of the organic matter. Usually, these values are 106 and 16, respectively, indicating the famous Redfield ratio of these elements in plankton. However, many aquarium foods deviate from this ratio, so I have left the equation in general form. What we are most concerned with right now, though, is the ammonium that is produced by this reaction. This ammonium is the first substance that then enters the classical nitrogen cycle.
It should be noted that – unlike the other processes we have discussed and will discuss – remineralization is carried out by every organism present in the aquarium (and ourselves, using O2 as the terminal electron acceptor), which means that this process is always going on in our aquarium, typically producing a lot of ammonium. Thankfully, a great deal of this ammonium is incorporated into new growth (Herbert 1999, Fennel et al. 2006), but a large portion also does enter the nitrogen cycle via nitrification.
Nitrification is a process by which the ammonium produced in remineralization is oxidized to nitrate. This is a two-step process with nitrite (NO2) as an intermediate, and is typically performed by a consortium of bacteria, with one group excreting nitrite that is consumed and oxidized by the second group. Of these two steps, it is the first (oxidation of ammonium) that is very slow, which is why nitrite levels are rarely detected in the home aquarium after the bacterial populations have been established. The nitrate that is produced in this process can be incorporated into biomass, but the majority of it enters the process that aquarists are most often concerned with: denitrification.
Denitrification
Denitrification is a heterotrophic process (meaning the organisms involved must consume their carbon, rather than producing it themselves) that reduces nitrate into nitrogen gas, and follows the general equation:
(CH2O)106(NH3)16 + 85NO3 + 100H+ –> 106CO2 + 16NH4+ + 43N2 + 149H2O
(Please Note that the equation is very slightly imbalanced, as I have rounded the decimal points in the stoichiometric coefficients).
The net result of this equation is the relatively large removal of nitrogen in the form of dinitrogen gas. Globally, this represents a major control on primary productivity (which requires nitrogen), and in the aquarium this represents one of the most effective ways we can remove nitrogen that may reach excessive levels. Although this process has been discussed at great length in the primary literature, I think the most important thing we can take from it right now is that this process requires organic material. This is a crucially important point I will return to later.
Additional processes affecting nitrogen
Although the processes described above constitute a fairly complete list of what can happen in our own systems, the picture in nature is more complex. Specifically, there are a couple of additional processes that can take place, which are worth mentioning in the spirit of completeness. It should be noted that these processes are relatively new to the scientific community, and so our understanding of them is unfortunately not entirely clear as of yet.

Figure 2: An expanded version of the nitrogen cycle, including sources of nitrogen as well as the relatively newly discovered processes DNRA and annamox.
One relatively newly-discovered process impacting nitrogen in the natural ecosystem is anaerobic ammonium oxidation, better known as annamox. In this process, certain anaerobic bacteria and archaea are able to essentially short-circuit the typical nitrogen cycle and react ammonium with nitrite to produce nitrogen gas. This represents a potentially very important alternative sink of nitrogen (Engstrom et al. 2005), but it is still not entirely clear under what conditions this process becomes important. It is most often noted in areas of the ocean with low oxygen levels combined with low levels of organic carbon, which is almost certainly not representative of the typical aquarium. In conditions more similar to those expected in our systems, annamox is much less frequent and so is likely not an important issue (Porubsky et al. 2009).
Another relatively “new” process is called dissimilatory reduction of nitrate to ammonium, or DNRA. Although energetically very similar to traditional denitrification, this processes represents a way for organisms to recycle nitrate rather than allow it to leave the system. Thankfully for our purposes, DNRA predominates almost exclusively in highly sulfidic sediments (Porubsky et al. 2009), which do not reflect the conditions found in most reef systems.
Nitrogen and the Sand Bed
A discussion of nitrogen cycling is nearly impossible to separate from a discussion of the sand bed in our aquaria, because it is in the sand bed where most of the processes take place. Of course, the carbonate matrix of live rock is also the site of a very active microbial community, and I will be discussing that in line with this discussion of the sand bed.
Although at times the presence of a sand bed is a contentious issue, I would argue that having a sand bed is a necessity for natural nitrogen cycling (please note, however, that I am not claiming that natural nitrogen cycling is the only valid aquarium husbandry method). Many aquarists might disagree with me on this point, and many in the hobby have argued that sand beds do more harm than good in the long run. This is often in part due to a fairly poor understanding of the processes that take place in marine sediments, but there are legitimate issues that must be considered in sand bed maintenance that I will discuss below. First, however, I must discuss the actual processes that take place in sediments, especially the ways in which they are connected to nitrogen cycling.
The Redox Cascade
Worldwide and especially in coastal areas including coral reefs, benthic sediments are the primary site of the diagenesis and remineralization that have been discussed above (Galloway et al. 2004). One reason for their primacy is the simple fact that organic wastes (which consist of dead organisms, feces, and nutrient-rich marine snow) end up in and make up the sediment layer; the majority of organic matter decomposition is simply where the organic matter ends up.
When organic matter ends up being deposited to the sediment, it immediately begins to undergo diagenesis. First and foremost, organic matter is consumed and metabolized using aerobic respiration, which uses oxygen as the terminal electron acceptor (“TER” in equation 1). However, the amounts of organic matter typically exceed the levels of oxygen, so organisms then begin to use “substitute” electron receptors in place of oxygen. The first of these is nitrate, via denitrification, which is thermodynamically not as “profitable” for organisms as aerobic respiration. This is why denitrification is always associated with anoxic sediments or water: oxygen is thermodynamically a favorable electron acceptor, and nitrate is only used as a last resort.
Once both nitrate and oxygen have been consumed, any remaining organic material continues to be broken down by energetically less favorable terminal electron acceptors according to a well-established cascade, continuing next with iron reduction, and finally with sulfate reduction. In most cases, organic matter is consumed long before sulfate is depleted, so our discussion can end with that step. Collectively, all of these metabolic pathways are termed the redox cascade, referring to the cascade of less desirable terminal electron acceptors as heterotrophic organisms consume organic matter in the sediment.
For most aquarists, our interest in the redox cascade can end with nitrate reduction, but as a side note I think it necessary to briefly discuss sulfate reduction. One potential argument that is used against sand beds is that it can potentially lead up to the buildup of toxic sulfides. However, this argument ignores the fact that iron reduction takes place before sulfate reduction. That fact is important for two reasons: 1) iron is plentiful in marine sediments and thus highly unlikely to be depleted in the relatively low nutrient loading we have in our aquaria and 2) any sulfide that is produced in the sediments will react to precipitate with the iron produced in iron reduction to form insoluble iron sulfide (FeS), thus acting to remove any of the potential sulfide. Of course, this is a complex subject worthy of its own article, so I will leave it with only this brief discussion.
Live Rock
As I mentioned above, live rock is also a major site for microbial activity including nitrogen cycling. Unfortunately, the particular dynamics are not as well studied as those specific to the sand bed, but the same basic principles apply in terms of the redox cascade. The primary difference between the live rock and sand bed is simple structure, which has pronounced effects on the speed of diffusion, the process that supplies most of the nitrate and other compounds that are metabolized in the redox cascade. One other major difference between the two sites of metabolism is one I have not yet mentioned: the possibility of bioturbation and bioirrigation.
Critters in the Mud
To me, one of the most fascinating aspects of the aquarium hobby isn’t the livestock that I purchase and add to my system, it’s the unintended occupants, the hitchhikers that end up in my tank either on purchases or as hitchhikers on the original live rock. However, the importance of these organisms goes beyond simple curiosity: these organisms are typically responsible for a pair of intricately related processes that play a pivotal role in the nitrogen cycle: bioturbation and bioirrigation. Although these two are technically distinct processes, they are nearly impossible to extricate from one another, and are often performed simultaneously by the same organisms.
Darwin’s Last Idea
Although certainly less well known than his theory of evolution, bioturbation as a phenomenon was actually first described by Charles Darwin in one of his last works (Meysman et al. 2006). In short, “bioturbation” is the term for the collective action of burrowing organisms that causes or enhances the physical mixing of sediments. Related to this is “bioirrigation,” which is the term for any active ventilation of burrows or surrounding sediments by the organisms that live within them. Together these processes play a vital role in coastal and benthic systems, to the point where such organisms are considered “ecosystem engineers,” organisms whose presence has a formative effect on the ecosystem around them (Jones et al. 1994).
One of the most important ways that these infauna (organisms that live within sediments) structure their environment is the formation of burrows within the sediment (see figure 3 for examples). These burrows represent a dramatic increase in the surface area of the sediment-water interface (SWI), the surface across which solutes such as nitrogen are exchanged between sediment and the overlying water. This exchange process is known as benthic-pelagic coupling, and serves as an important avenue of “communication” between coastal waters and the sediment that serves as their natural filter. More importantly, these burrow structures usually extend into the anoxic zone, and thus serve as important sources of oxygen to be used in nitrification. Further down the nitrogen cycle, the presence of oxic zones caused by burrows in otherwise anoxic sediments stimulates what is called “coupled denitrification”, which is simply denitrification that is “powered” by nitrate that has just been generated in nitrification (Kristensen et al. 1987).
In addition to simply extending the surface area for diffusive exchange, the active irrigation of burrows often actively supplies both oxygen and nitrate to be used in both nitrification and denitrification at a rate much faster than could be supported by molecular diffusion (Henriksen et al. 1983, Huettel 1990 among many others). This can result in rates of denitrification that are orders of magnitude higher than those in “barren” sediments, and these organisms can in fact be responsible for ecosystem recovery after periods of nitrogen eutrophication (Bartoli et al. 2000). On a more physical basis, the action of bioirrigating and bioturbating organisms can actually control the physical makeup of the sediment (Volkenborn et al. 2007), preventing the type of “clogging” that is often raised as a potential long-term issue with sand beds.
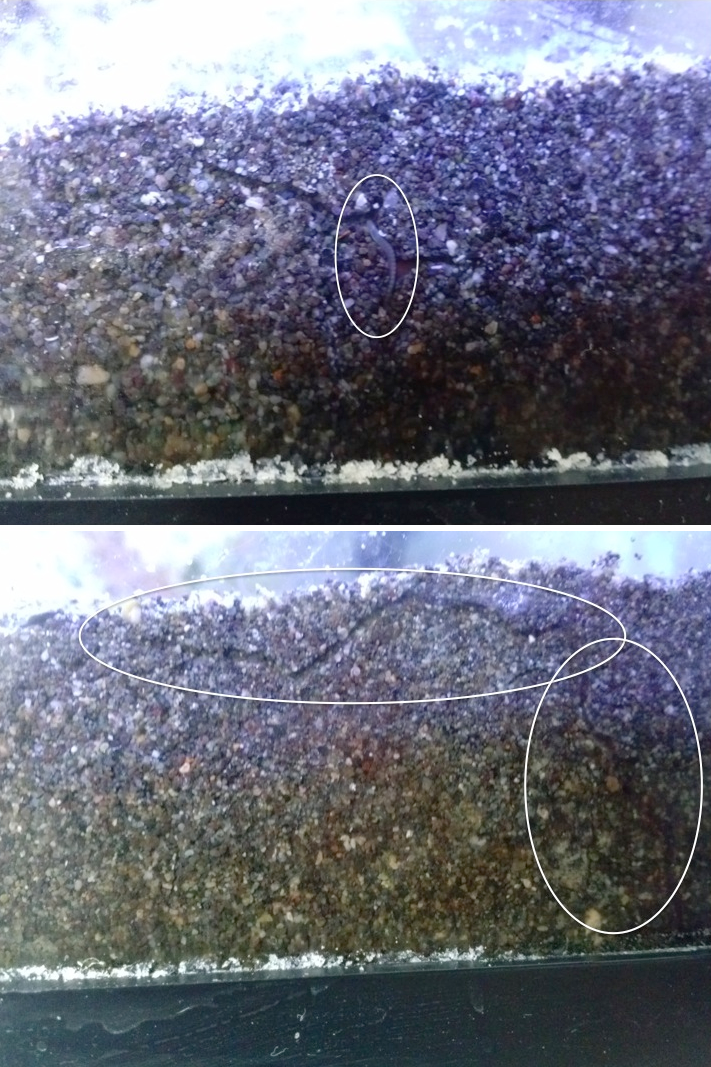
Figure 3: Evidence of bioturbation in the home aquarium. TOP shows a polychaete worm within its burrow and BOTTOM shows multiple burrow traces, including one extending down into the anoxic zone (seen as darker sediment, though the author’s use of black sand makes this difficult to see)
Bioturbation in the home aquarium
Although the vast majority of bioturbating organisms that have been studied are relatively large and unlikely to be encountered in the aquarium (such as the lugworm, Arenicola marina, which many European aquarists might be familiar with from the Wadden Sea), there are a number of organisms that are responsible for sediment mixing and burrow formation that can be found within the home aquarium. Chief among these are the numerous polychaete species that can usually be found actively burrowing in most aquaria (Figure 3 has an example). Yes, believe it or not, those bristleworms many of us once feared can actually be responsible for enhancing nitrate removal from our systems!
Another group of organisms responsible for bioturbation in the home aquarium are the amphipods. Indeed, large amphipod burrows can often be seen against the side of the aquarium glass (see Figure 3 for a possible example), and these organisms can be responsible for moving very large volumes of overlying water through their burrow as they ventilate it to provide oxygen. Sadly, there is currently little to no direct study of bioturbation in the home aquarium, but there is no reason to believe it does not play as important a role in the hobbyist’s tank as it does in nature. In fact, it is distinctly possible that one factor that leads to the stability of a mature aquarium is the development of a healthy population of sediment infauna. Furthermore, one possible explanation of “old tank syndrome” is the decline of the infaunal population, with a subsequent decline in rates of nitrification and denitrification. Of course, this possible explanation is at this point just speculation, and would need rigorous experimentation to move beyond conjecture.
One thing that I have not yet mentioned is the role of bioirrigation within live rock. Although the solid nature of the rock prevents bioturbation (mixing) from playing any sort of role, the organisms that live within live rock must still ventilate burrows to provide oxygen, and so they can have many of the same effects as their relatives that live in the sand bed. Unfortunately, bioirrigation within solid structures such as live rock is entirely uninvestigated, though that does provide an exciting possible area of study. What we do have, however, is the observation that our systems seem to mature and stabilize at the same time as populations of polychaetes and microcrustaceans become well established and visible on both live rock and the sand.
Carbon Matters
Having introduced what I hope is a more comprehensive picture of nitrogen cycling and examined the roles that both the sand bed and sand bed organisms play in the nitrogen cycle, I have one final aspect of this whole picture that I wish to discuss: carbon.
If there has been one overall trend in the 20 years I’ve been a hobbyist that has promised to enhance the viability of our captive systems, it has been the movement from relatively restricted nutrient input to active supplementation of carbon via a number of methods. On its surface the argument is clear: carbon is the basic food for the ecosystem, and more food enhances growth. Although this is a deliberate oversimplification of the idea to save space, I hope to expand on this idea by directly linking carbon to the nitrogen cycle in two distinct ways.
Carbon and Nitrogen removal
When I was first introduced to the idea of adding carbon, the basic science supporting the idea was that of the Redfield ratio. In this justification, it is posited that our aquaria have an excess of nitrogen compared to carbon, which in nature typically exist at a C:N ratio of 106:16. While not an untrue statement, the Redfield ratio indicates the elemental makeup of plankton, and so invoking the ratio as an argument assumes that nitrate is consumed and incorporated as biomass. Although this is certainly the fate of some of the nitrate in the system, the vast majority of natural removal is through the process of denitrification rather than assimilation. This is not, however, to suggest at all that carbon and the C:N ratio are unimportant, but rather that the reasoning has less to do with the Redfield ratio, and much more to do with the redox cascade.
As I present above, carbon is the overall fuel that powers the redox cascade that takes place within sediments and live rock, and of which denitrification is one major step. What this means is that in order for denitrification to take place, there must be sufficient organic material to not only consume oxygen, but also to be used in denitrification itself. In most aquaria, the former condition is usually met, and the formation of anoxic zones can be assessed easily by judging the color of the sediment. However, especially in cases of very large excesses of nitrate, there may be insufficient carbon to fuel enough denitrification to remove all nitrates present. In these cases, addition of carbon provides the essential fuel for this process, which is what leads to the reduction of nitrate in cases where carbon is limited.
This is one way that carbon addition can impact nitrogen cycling, but I wish to propose an additional mechanism that directly invokes the bioturbation discussed above, but is significantly more complex and related more to our system ecology.
Carbon and bioturbation
As I describe above, the presence and activity of infauna can have a profound impact on nitrogen cycling, directly enhancing rates of nitrification and especially denitrification. In many cases, the impact of infauna is directly linked to diversity (Norling et al. 2007) and/or organism density (Waldbusser et al. 2004), such that increasing diversity and density either linearly or even exponentially increases the rates of denitrification. In all cases, these infauna are heterotrophic, so increasing the supply of nutrients such as carbon directly impacts the availability of their food. The increased food availability then supports larger populations, directly increasing the density of these beneficial organisms.
In addition to simply supporting larger populations, increased flow of energy in the form of carbon (and nitrogen, though that is a subject worthy of an article on its own) supports more diversity, in part due to decreased competitive pressures (though the particular mechanism is not entirely known, even if the relationship is well documented). Because faunal diversity is positively correlated with the sediment’s ability to remove nitrogen via denitrification, this is another way that carbon enhances our system’s ability to remove and control nitrogen levels. So although it’s not new advice, hopefully this gives some added scientific impetus to the growing trend of carbon and nutrient supplementation to our systems.
Conclusion
Although most home aquarists have a basic understanding of the nitrogen cycle from almost the very first day of aquarium keeping, the understanding of many is fairly limited. The classical nitrogen cycle is not incorrect, but the nuances of the cycle and especially the factors that control it are largely unreported in common aquarium literature. In addition to details of the nitrogen cycle itself, I have provided what I hope is valuable insight into the different factors that control the ways and speeds with which nitrogen moves through our systems. I have also introduced the concepts of bioturbation and bioirrigation, the ways in which all the miscellaneous and often unexpected organisms that find their way into our tanks contribute to their overall health. It is my hope that this information will not only foster an appreciation for organisms that in the past have been considered pests, but also foster an understanding of aspects of ecology that we as aquarists can use to our advantage. Finally, I have discussed the importance of carbon in this entire big-picture discussion of nitrogen cycling, and I hope that I have provided additional encouragement to – if not exactly actively dose carbon – move away from the old paradigm of essentially starving our reefs.
Literature Cited
- Bartoli, M., D. Nizzoli, D.T. Welsh, and P. Viaroli. 2000. Short-term influence of recolonisation by the polychaete worm Nereis succina on oxygen and nitrogen fluxes and denitrification: a microcosm simulation. Hydrobiologia 431: 165-174
- Berg, P., S. Rysgaard, and B. Thamdrup. 2003. Dynamic modeling of early diagenesis and nutrient cycling. A case study in an arctic marine sediment. American Journal of Science 303: 905-955
- Engstrom, P., T. Dalsgaard, S. Hulth, and R.C. Aller. 2005. Anaerobic ammonium oxidation by nitrite (annamox): implications for N2 production in coastal marine sediments. Geochimica et Cosmochimica Acta 69: 2057-2065
- Fennel, K., J. Wilkin, J. Levin, J. Moisan, J. O’Reilly, and D. Haidvogel. 2006. Nitrogen cycling in the Middle Atlantic Bight: results from a three-dimensional model and implications for the North Atlantic nitrogen budget. Global Biogeochemical Cycles 20: GB3007 doi: 10.1029/2005GB002456
- Galloway, J.N., F.J. Dentener, D.G. Capone, E.W. Boyer, R.W. Howarth, S.P. Seitzinger, G.P. Asner, C.C. Cleveland, P.A. Green, E.A. Holland, D.M. Carl, A.F. Michaels, J.H. Porter, A.R. Townsend, and C.J. Vorosmarty. 2004. Nitrogen cycles: past, present, and future. Biogeochemistry 70: 153-226
- Gruber, N. and J.L. Sarmiento. 1997. Global patterns of marine nitrogen fixation and denitrification. Global Biogeochemical Cycles 11: 235-266
- Henriksen, K., M.B. Rasmussen, and A. Jensen. 1983. Effect of bioturbation on microbial nitrogen transformations in the sediment and fluxes of ammonium and nitrate to the overlying water. Environmental Biogeochemistry 35: 193-205
- Herbert, R.A. 1999. Nitrogen cycling in coastal marine ecosystems. FEMS Microbiology Reviews 23: 563-590
- Huettel, M. 1990. Influence of the lugworm Arenicola marina on porewater nutrient profiles of sand flat sediments. Marine Ecology Progress Series 62:241-248
- Jones, C.G., J.H. Lawton, and M. Shachak. 1994. Organisms as ecosystem engineers. Oikos 29: 373-386
- Kristensen, E. and T.H. Blackburn. 1987. The fate of carbon and nitrogen in experimental marine sediment systems: influence of bioturbation and anoxia. Journal of Marine Research 45: 231-257
- Larkum, A.W.D., I.R. Kennedy, and W.J. Muller. 1988. Nitrogen fixation on a coral reef. Marine Biology 98: 143-155
- Meysman, F.J.R., J.J. Middelburg, and C.H.R. Heip. 2006. Bioturbation: a fresh look at Darwin’s last idea. Trends in Ecology and Evolution 21: 688-695
- Norling, K., R. Rosenberg, S. Hulth, A. Gremare, and E. Bonsdorff. 2007. Importance of functional biodiversity and species-specific traits of benthic fauna for ecosystem functions in marine sediment. Marine Ecology Progress Series 332: 11-23
- Porubsky, W.P., N.B Weston, and S.B. Joye. 2009. Benthic metabolism and the fate of dissolved inorganic nitrogen in intertidal sediments. Estuarine, Coastal and Shelf Science 83: 392-402
- Volkenborn, N., L. Polerecky, S.I.C. Hedtkamp, J.E.E. van Beusekom, and D. de Beer. 2007. Bioturbation and bioirrigation extend the open exchange regions in permeable sediments. Limnology and Oceanography 52: 1898-1909
- Waldbusser, G.G., R. L. Marinelli, R.B. Whitlach, and P.T. Visscher. 2004. The effects of infaunal biodiversity on biogeochemistry of coastal marine sediments. Limnology and Oceanography 49:1482-1492
About the Author
Tommy Dornhoffer is currently (as of Spring 2014) a fifth-year marine sciences PhD student at the University of Georgia in Athens, GA, as well as an avid aquarist with nearly two decades of aquarium experience. He specializes in benthic ecology (the study of the organisms in sediments and their effects) and nitrogen cycling, and loves doing anything he can to further our understanding of these fields. He is also an active instructor at UGA, and loves teaching almost as much as he loves his tanks.
0 Comments