In my previous article I discussed the first of two important experiments that would advance our knowledge of coral propagation and husbandry. I started out by identifying some of the reasons why the experiment, as well as the approach to growing corals from larvae was essential towards developing techniques that would both produce corals for the aquarium trade and conserve our natural reefs. In the following experiment, I was similarly concerned about reef conservation, but an equally strong influence was a desire to develop new techniques relevant to current coral culture practices based on well designed scientific experiments.
Hypothesis Development
You may be wondering why I invested a few years, shed a few tears, and pulled out a few hairs to test a hypothesis that seems to have been addressed for many years within the aquarium trade. Most, if not all coral farmers have dealt with this issue to some degree and many have made adjustments to their methods as a direct result of interactions between two corals (E. Borneman pers. comm. 2006). In the literature, there are more than a few papers that investigated the effects of coral interactions with conclusions that all suggested that any type of interaction would result in a decrease in coral growth rate (Rinkevich and Loya 1985; Tanner 1997). I felt, however, that we still lacked a thorough understanding of coral interactions and that, through more knowledge; techniques could be refined to increase productivity for captive-raised corals.
Corals competition has been observed in both the field and aquaria (Lang and Chornesky 1990, Shimek 2003). Corals use a variety if competitive mechanisms which can be divided into two broad categories: Direct and Indirect (Connell 1973). While I’m not going to turn this into a discussion about all of the different ways corals compete, I do want to discuss the mechanism that was important towards the development of my hypothesis: the indirect mechanism called overtopping. Overtopping occurs when a coral grows above its neighbors to gain access to environmental resources such as sunlight and water circulation and indirectly restricts these resources to underlying colonies. Indirect mechanisms are used primarily by branching and massive corals such as acroporids and branching poritids (Baird and Hughes 2000, Connolly and Muko 2003). In defense of their space and existence, corals engaged in competition with another species must invest high energetic costs into the development and maintenance of competitive mechanisms (Chornesky 1989; Romano 1990). Overtopping also requires an energy investment, however, the energy is invested in growth rather than the development of structures used for physical confrontations.
This idea got me thinking which ultimately lead to the keystone point of my thesis: For corals that primarily use overtopping, their success is a direct function of their growth rate. Thus, in the presence of a competitor, growth rates must be maximized to ensure dominance. Could this be a passive way to increase growth rates? With that in mind I first want to discuss why this would be important for farmers who culture corals for the aquarium trade.
Since it has been well documented that corals engaged in direct competition will usually show signs of stress, including reduced growth rates and tissue loss (Rinkevich and Loya 1985), spacing corals to avoid competition can mitigate potential negative effects on growth. Due to the lack of information on proper spacing, farmers often make subjective decisions on how far apart each coral will be spaced. Charles Delbeek (pers. comm. 2006), for example, placed corals at least at 3 cm apart, and increased the distance as the corals grow larger. Arbitrary distances, however, may increase the costs associated with growing the coral to a marketable size. Food, lighting, water movement and water quality control all represent overhead costs in the form of equipment, electricity, and labor (Wheaton 1993). These costs can be a direct function of space in the tank. For example, the cost to provide adequate lighting to a culture tank is determined by the electrical costs, measured in watts / gallon. A general rule for maintaining healthy corals is 3-5 watts / gallon. The electrical costs are a function of the amount of wattage used; therefore the cost to provide adequate lighting for a 1000-gallon tank would be significantly higher than the cost for a 100-gallon tank. Profits for a coral-propagating facility are generated by the number of corals it can sell and the speed in which they can be grown to marketable sizes (Ellis and Ellis 2002). The number of corals in a facility is a function of the size of the culture tanks and the number of corals per tank, determined by the spacing. Spacing corals far apart would reduce the total number of corals per tank and require the facility to maintain larger tanks, resulting in increases in overhead costs. Growing corals quickly to marketable sizes would allow the facility to distribute a larger quantity faster. There is a significant difference, therefore, in the facility costs between corals that reach marketable sizes in three months versus six months. These issues can be summed to the question: Can the optimum spacing of corals be used to maximize the number of corals per tank and promote the fastest growth rates? In other words, could a more complete understanding of coral interactions be a passive and cost effective way to increase growth rates?
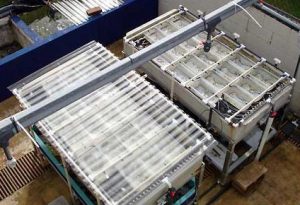
Water tables used for the experiment. One shown with the cover used to prevent rainwater from entering each container.
To determine the optimum spacing distances and the effects of interaction on two species of branching corals cultured together, I asked the following question in my thesis: Will growth be significantly affected for each of the two species of corals cultured together at three different spacing distances? For example, will linear growth be affected? Growth at the base of the fragment is important for stability will the presence of a neighbor affect growth and, thus, stability? Both species are exhibit branching morphologies; will the presence of a neighbor affect the number of new branches that develop on a fragment? Further, since corals can exhibit growth either towards or away from a competitor, will the orientation of new branches follow a pattern that is affected by a neighbor?
Methods
Selection of study species and culture set-up
I chose Acropora pulchra and Porites cylindrica as my test subjects because they both exhibit branching morphologies and both are popular within the aquarium trade. Two covered water tables (Figure 1) were placed side by side in direct sunlight on the East Lanai at the Laboratory. Epoxy base and container design are shown in Figure 2a-c. Exact orientation for each fragment within the container was obtained by photographing the initial layout of the fragments within the containers and making subsequent comparisons. Corals were fed daily with approximately 2.5 g (pre-hatched) Artemia franciscana (GSL© Premium, 90% hatch). Containers and epoxy bases were cleaned twice per week.
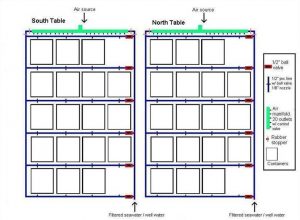
Figure 1. North and South water tables. Seawater from Pago Bay reef flat was filtered with 20 μm Pentair Aquatics© mechanical filters and fed each table via ½’’ line connected to the Laboratory’s main seawater source. Two nozzles distributed water into each container. There was no significant difference in the water flow rates between containers (Mean = 12. 31 ml/s-1, +/- 0.13; ANOVA; df = 79, F = 0.3623, P = 0.5547, p = 0.05, Tukey-Kramer test, North table = South table). Air was supplied to each container from lines connected to the main compressor. Rows and containers within each row were rotated on a weekly basis.
Experimental design
Clonal fragments of each species were exposed to three spacing treatments; Apart, Near, and Crowded. The distances for each treatment were as follows: ‘Apart’:140 mm; ‘Near’: 33 mm; ‘Crowded’: 8 mm. Each treatment consisted of one un-branched fragment of P. cylindrica and one un-branched fragment of A. pulchra in a single container positioned at one of the three spacing distances. Each replicate represented one clonal group of each species placed at the three spacing treatments (Figure 3). The ‘Apart’ treatment was designed so that corals would have no possibility of growing into contact within the six month period. The ‘Near’ treatment was designed so that the growth of the corals over a six-month period might result in contact between the two species. The ‘crowded’ treatment was designed so that the growth of the corals would likely result in physical contact between the two species within two months. This experiment was replicated twelve times, with each replicate containing different genotypes of P. cylindrica and A. pulchra. Rows and containers within each row were rotated on a weekly basis so that each container received similar exposure to the environment over the course of the experiment.
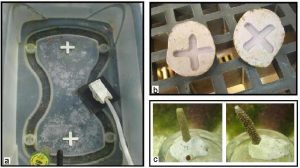
Figure 2a-c. Each container had a tile spacer glued into the appropriate distance location (a). The same tile spacer was used to create an indent in the fragment base (b).This ensured that the exact position of the fragment was maintained throughout the experiment. Fragments were embedded into the epoxy base and remained in the flow through seawater system for 24 hr to acclimate (c). Exact orientation for each fragment within the container was obtained by photographing the initial layout of the fragments within the containers and making subsequent comparisons.
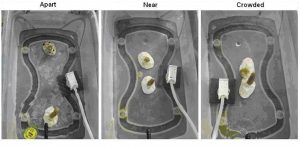
Figure 3. Clonal fragments of each species were exposed to three spacing treatments; Apart, Near, and Crowded. The distances for each treatment were as follows: ‘Apart’:140 mm; ‘Near’: 33 mm; ‘Crowded’: 8 mm. Each replicate represented one clonal group of each species placed at the three spacing treatments and was blocked together during the experiment. Each container had one air- source and two water delivery nozzles.
Test parameters for hypothesis
To test the hypothesis that growth of each species of coral will be affected when cultured together, I measured the total linear and basal width growth (Figure 4) and growth rate, total weight, counted the number of new branches (Figure 5) and determined the orientation of new branches for each fragment. To measure total weight of each fragment, first, based on the initial length and width measurements (35 mm long by 8 mm wide) I used a Sartorius Instruments© analytical balance to obtain the average (n = 20) dry weight of skeletal fragments, in air, for each species. Fragments were bleached and placed in the sun to air dry. Prior to weighing, fragments were placed in an oven (350º F for 15 minutes) to eliminate moisture. At the end of the experiment, I bleached and baked each fragment and used the same balance to obtain the final weight. The initial weight was subtracted from the final weight to determine the total weight. A two-factor ANOVA (treatments & clones) was used to analyze the data. Branch orientation was determined by two methods. First, new branches that developed on each clone were tested for uniformity around the axis of the main fragment with the Rayleigh’s test for circular uniformity. Rayleigh’s test generates a z statistic which was used in the two-factor ANOVA. Second, new branches were categorized as either facing towards or away from the neighbor (Figure 6).
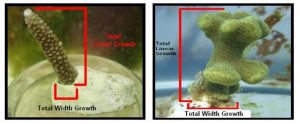
Figure 4. Measurements on total linear and basal width growth (mm) were obtained throughout the study period. For each fragment, measurements were taken once per month for six months.
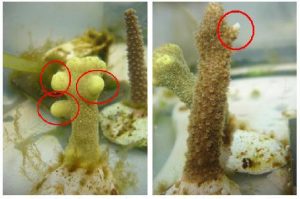
Figure 5. New branches were categorized as any protrusion growing off the main axis with a measurable length and direction.
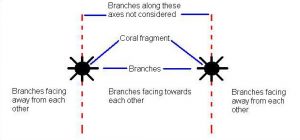
Figure 6. New branches were categorized as either facing towards or away from the neighbor. Branches growing orthogonal to the line of separation were not used in the analysis and a ratio was calculated as the number of branches facing toward the neighbor / total number of branches was determined. A ratio greater than 0.5 meant the majority of the branches were facing towards the neighbor. Similarly, a ratio less than 0.5 meant that the majority of the branches were facing away from the neighbor.
Results
With all of the test parameters, you can imagine I was able to develop quite a large set of results. Fortunately for everyone, I will only report on the parameters that yielded significant results; linear growth and growth rate, number of branches, and weight. Basal growth, which accumulated for both species and occurred in all treatments and branch orientation, did not yield significant results. Further, there was no significant difference among clones in their response to the treatments.

Porites cylindrica showed signs of significant tissue damage when engaged in physical contact with A. pulchra.
In January, A. pulchra began to show a difference in cumulative linear growth and growth rate between treatments, with fragments in the ‘Crowded’ treatment engaging in physical contact with P. cylindrica and growing more than fragments in the ‘Apart’ and ‘Near’ treatments (Figure 7 and 8). By the end of the experiment, fragments in the ‘Crowded’ treatment had grown significantly more than fragments the ‘Apart’ treatments. Porites cylindrica showed no significant difference in total cumulative linear growth or growth rate between treatments (Figure 9 and 10). Although both species had significant differences in the number of branches between ‘Crowded’ and ‘Apart’ treatments, the responses were just the opposite. Acropora pulchra had the highest number of branches in the ‘Crowded’ treatment (Figure 11) whereas P. cylindrica had the highest number of branches in the ‘Apart’ treatment (Figure 12). Corresponding to the significant difference growth rates between treatments, there was a significant difference between treatments in the mean total weight increase for A. pulchra (Figure 13) and P. cylindrica (Figure 14). Weight increases for A. pulchra were significantly greater in the ‘Crowded’ treatment than in the ‘Apart’ treatment. Fragments of P. cylindrica in the ‘Apart’ treatment were significantly heavier than in the ‘Crowded’ treatment.
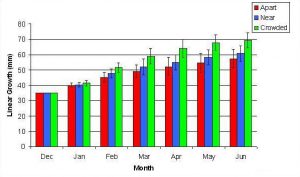
Figure 7. Mean (+/- SD) cumulative linear growth for A. pulchra in three spacing treatments (Apart, Near, Crowded, n = 12 per treatment). Data was analyzed with repeated measures, two-factor ANOVA without replication. Fragments in the Crowded treatment grew significantly more than fragments in the Apart treatment (ANOVA: df = 2, 33; F = 3.51; P = 0.0414, Tukey-Kramer test, p = 0.05).
By the second month 11 of 12 fragments in the ‘Crowded’ treatment had grown into physical contact of each other. This appeared to elicit a competitive response in which A. pulchra was dominant over P. cylindrica. This was apparent from observed tissue loss at the area of contact and reduced growth in P. cylindrica.
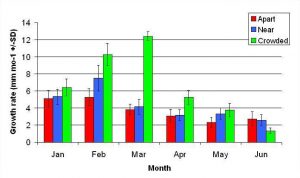
Figure 8. Mean monthly (30 day) linear growth rate (+/- SD) for A. pulchra in three spacing treatments (Apart, Near, Crowded, n = 12 per treatment). Data was analyzed with repeated measures, two-factor ANOVA without replication. Fragments in the Crowded treatment grew significantly more than fragments in the Apart treatment (ANOVA: df = 2, 33; F = 9.52; P < 0.0005; Tukey- Kramer test, p = 0.05).
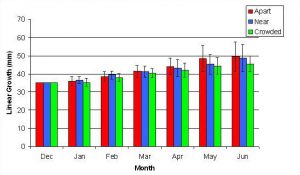
Figure 9. Mean (+/- SD) cumulative linear growth for P. cylindrica in three spacing treatments (Apart, Near, Crowded, n = 12 per treatment). Data were log transformed and analyzed with repeated measures, two-factor ANOVA without replication. There was no significant difference between treatments (ANOVA, df = 2, 33; F = 1.05; P = 0.3621, Tukey-Kramer test, p = 0.05). Untransformed means are reported for clarity.
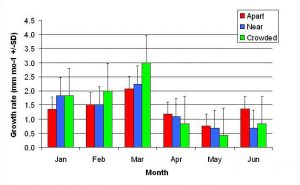
Figure 10. Mean monthly (30 day) linear growth rate (+/- SD) for P. cylindrica in three spacing treatments (Apart, Near, Crowded, n = 12 per treatment). Data were log transformed and analyzed with repeated measures, two-factor ANOVA without replication.There was no significant difference between treatments (ANOVA: df = 2, 33; F = 0.68; P = 0.5153, Tukey-Kramer test, p = 0.05). Untransformed means are reported for clarity.

Figure 11. Mean (+/- SD) number of branches for A. pulchra in three spacing treatments (Apart, Near, Crowded, n = 12 per treatment). Count data were square root transformed and analyzed with a two factor ANOVA without replication. There was a significant difference between treatments with fragments in the Crowded treatment growing more branches than fragments in the Apart treatment (ANOVA: df = 2, 33; F = 2.70; P = 0.0823, Tukey-Kramer test, p = 0.05). Untransformed means are reported for clarity.
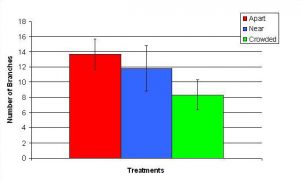
Figure 12. Mean (+/- SD) number of branches for P. cylindrica in three spacing Treatments (Apart, Near, Crowded, n = 12 per treatment). Count data were square-root transformed and analyzed with a two factor ANOVA without replication. There was a significant difference between treatments with fragments in the Apart treatment growing more branches than fragments in the Crowded treatment (ANOVA: df = 2, 33; F = 2.70; P = 0.0823, Tukey-Kramer test, p = 0.05). Untransformed means are reported for clarity.
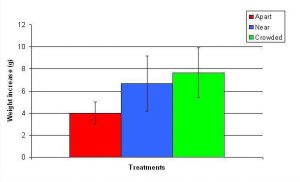
Figure 13. Mean (+/- SD) total growth (weight) increase for A. pulchra in three spacing treatments (Apart, Near, Crowded, n = 12 per treatment). Data were log transformed and analyzed with a two factor ANOVA without replication. There was a significant difference between treatments with fragments in the Crowded treatment weighing more than fragments in the Apart treatment (ANOVA: df = 2, 33; F = 5.03; P = 0.0124, Tukey-Kramer test, p = 0.05). Untransformed data are reported for clarity.
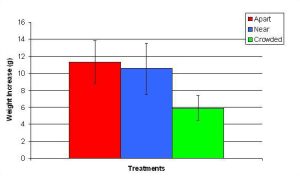
Figure 14. Mean (+/- SD) total growth (weight) increase for P. cylindrica in three spacing treatments (Apart, Near, Crowded, n = 12 per treatment). Data were log transformed and analyzed with a two factor ANOVA without replication. There was a significant difference between treatments with fragments in the Crowded treatment weighing more than fragments in the Apart treatment (ANOVA: df = 2, 33; F = 3.77; P = 0.0336, Tukey-Kramer test, p = 0.05). Untransformed data are reported for clarity.
Discussion
This study showed that culturing A. pulchra and P. cylindrica at three spatial distances from each other resulted in different responses in growth and morphology. Growth in P. cylindrica was directed towards the increase in the number of branches and growth in A. pulchra involved linear extension with branch development at the tips (Figure 15). Although I predicted that some of the growth patterns and competitive interactions for each species would be consistent with previous findings, the increase in growth rates of A. pulchra in response to direct contact with P. cylindrica was not predicted. Previous studies have demonstrated that corals engaged in physical contact show reduced growth rates due either to the stress of physical engagement or the development and maintenance of competitive mechanisms (Rinkevich and Loya 1985; Chornesky 1989; Tanner 1997).
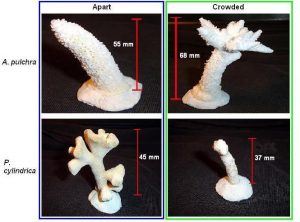
Figure 15. Fragments in the ‘Apart’ and ‘Crowded’ treatment. Two things were clear: 1) Acropora pulchra showed more growth in treatments where physical contact occurred, whereas P. cylindrica showed more growth in treatments where no physical contact occurred and, 2) growth for A. pulchra involved linear extension with branch development at the tips and growth for P. cylindrica was directed towards the increase in number of branches.
The growth of undisturbed fragments of A. pulchra and P. cylindrica were consistent with previous findings (Soong & Chen 2003; Smith 2004). For basal width growth, Clark and Edwards (1995) found that basal growth stabilized growing colonies and was important to later growth and survival of transplanted fragments. The higher basal width growth rates in the first three months suggest that fragments of both species were attempting to stabilize on the substrate. Each species initially exhibited reduced growth rates relative to that of parent colonies on the reef which was most likely due to transplant stress (Yap and Gomez 1984; Raymundo 2001).
Growth of Acropora pulchra
The high linear growth rates exhibited by fragments in the ‘Crowded’ treatment suggested that a competitive response was initiated due to the physical contact with its neighbor (Figure 7 and 8). However, by the third month, the main fragments had reached the surface of the water. At that time, a change in growth patterns was observed. Linear growth rate declined and new branch development at the top of the fragment started to increase. The reduction in linear growth rate was probably due to the limit set by reaching the water surface. While branching may be attributed to the restriction in the ability to grow in length, the increase in branching at the tip of the fragments in the ‘Crowded’ treatment suggested that contact with P. cylindrica may be further evidence of competitive response. In the ‘Near’ treatment many of the fragments grew to reach the surface of the water but did not branch as extensively as fragments in the ‘Crowded’ treatment. Soong and Chen (2003) found that branches developed quicker on longer fragments. While this observation may be a genetically fixed growth pattern, the increase in growth of fragments in the ‘Crowded’ treatment meant that they attained the minimum linear growth more quickly than fragments in other treatments and, thus developed new branches sooner. In this case, overtopping as an indirect mechanism to dominate neighboring corals is most likely a function of available resources and growth morphology and not a direct response to the presence of a competitor.

Undisturbed fragments grew as predicted, with linear extension and some branching for fragments of A. pulchra and extensive branching occurring for fragments of P. cylindrica.
I did not determine if A. pulchra was feeding directly on tissue or mucus (Kato 1987) of P. cylindrica, however, it is possible that fragments of A. pulchra in the ‘Crowded’ treatment were exposed to these additional food sources. Another type of competitive mechanism employed by corals including A. pulchra is a direct mechanism called extracoelenteric digestion. This is where mesenterial filaments originating from the gut are used to damage the soft tissue of a neighboring coral. While it has not been shown that corals are able to digest other corals as food, they are predators and eat a variety of reef organisms (Goreau et al. 1971). Further, coral mucus, which contains high levels organic matter, is consumed as food by many benthic organisms including corals (Wild et al. 2004). Moreover, another additional food source could have also come from the exposure to higher amounts of Artemia franciscana. Ferrier-Pages et al. (2004) observed that colonies of Stylophora pistillata continue to consume prey as long as they were available, never becoming satiated. As a result of the tissue loss to P. cylindrica, a higher amount of supplemented food may have been available to A. pulchra. Therefore, while the higher growth of fragments in the ‘Crowded’ treatment may be an overtopping response, the success in being able to do so may be due to the increase in resources resulting from additional resources.
Growth of Porites cylindrica
In treatments growth appeared to be consistent with growth patterns observed in other studies (Custodio and Yap 1997; Smith 2004). The significant tissue loss exhibited by P .cylindrica in treatments where physical contact occurred suggested that being physically subordinate to A. pulchra can have a negative effect upon growth. Similar to A. pulchra, the least amount of linear growth occurred at the end of the study period. Unlike A. pulchra, fragments did not reach the water surface and, therefore, the limited linear growth was not due to that particular barrier. Rather, fragments in the ‘Crowded’ treatment were damaged severely by physical contact and no longer appeared to grow. Even though fragments in the other treatments continued to grow longer, they appeared to direct most of the new growth into the development of new branches. Branching patterns did not orient towards or away from A. pulchra. In the ‘Near’ treatment, however, branches that grew into contact with A. pulchra subsequently experienced tissue damage at the point of contact. Rinkevich and Loya (1983) suggested an allelopathic mechanism was at play when they showed that subordinate colonies of Stylophora pistillata grew away from the dominant colony prior to any signs of physical contact. This was not observed in this experiment and would suggest that P. cylindrica may not be able to detect the presence of A. pulchra until they come into physical contact with each other. While no measurements where made on the growth rate of individual branches, no further growth was apparent on those branches that had made contact with A. pulchra. The experiment was terminated prior to determining if new branches would have followed a new pattern of orientation once they came into direct contact with A. pulchra.
The clonal design of our experiment allowed us to test for a genetically-based response to competition, and by minimizing variation in the physico-chemical environment of the fragments; we could conclude that any response observed was triggered by an effect of neighboring competitor. Clones in all treatments and both species showed no observable difference in their phenotypic response to the varying distances of their neighbor; responses from all clones in all treatments were similar. This provides evidence that our observed results were the product of interspecific competition which triggered a genetically-controlled, morphologically-plastic response. Environmental conditions are known to trigger changes in morphologically plastic organisms such as corals (Bruno and Edmunds 1997; Muko et al. 2000). In this case, the close proximity of a competing neighbor triggered a change in linear growth and branching rate. These changes reflected both the competitive status of each species (i.e., A. pulchra, dominant; P. cylindrica, subordinate) and in species-specific strategies (linear upward growth vs. lateral branching).
Conclusions
Clearly, A. pulchra exhibited a dominant competitive response, but was the higher growth a competitive response to the neighbor (overtopping) or an indirect result of the elevated nutritional resources gained from the use of a direct competitive mechanism (extracoelenteric digestion)? Probably a little of both as they are intimately linked; additional resources equal additional growth. In this case, growth was directed towards linear extension (and perhaps branch development). For P. cylindrica, clearly, they did not like to be in physical contact with A. pulchra. Ultimately, what we are interested in is how these results can be integrated into coral culture techniques.
Implications for Aquaculture
Currently, many coral farmers believe coral health and growth are optimized when they are spaced away from each other and grown in tanks containing similar species. I have shown, however, that careful attention to spacing distances and the poly-culture of different corals can result in higher growth rates. Spacing fragments, either away from a neighbor to inhibit physical contact, or within physical contact to promote a competitive response, can be a cost effective way to increase the growth rate and optimize culture tank space. Financial sustainability for many coral farms, however, often requires that all of their corals be healthy in order to increase the number of marketable corals. Therefore, the increase in growth at the expense of another coral colony may not be desirable. Although the exact mechanism for the increased growth found in this study has not been identified, it is most likely the subordinate coral that provided extra nutrition to the other coral. Land-based coral farms may be able to achieve similar results if they are able to provide constant food; however this is not cost effective. One possible scenario could be the growth of a less marketable coral species to act as a donor colony for fragments that may be used to promote a competitive response in corals that have high market values. Further, the subordinate coral may take the place of food additions, thus reducing the labor and costs associated with live food supplementation.
Another possible scenario is one that is not much different from what many coral farmers indirectly contend with. Corals grown in a tank are spaced as close as possible and moved apart at the first signs of interaction. Charles Delbeek (Pers comm. 2006) practices this technique in his culture tanks; however, he cultures similar species of corals together and is concerned with the possibility of corals fusing once, upon physical contact, they recognize each other. However, this experiment as well as other studies (Dizon and Yap, 2000) suggests that polyculturing may be more appealing. Thus another scenario may be to stimulate early growth, overtopping corals that are grown in crowded multi-species environments maybe induced to grow faster compared to those grown in isolation. Once the corals come into contact they can be moved apart. To incorporate the knowledge gained from this experiment, the only difference would be that farmers become more familiar with how two species of corals interact and use species that would demonstrate overtopping as a competitive response.
An indirect result of this experiment is the response of fragments upon reaching the water surface. In the aquarium trade, corals that have extensive branching are more marketable (E. Borneman, pers. comm. 2005). Because extensive branching happened once the fragment reached the water surface, branch development may be stimulated in shallow tanks. The combination of more resources and shallow water levels may further reduce the amount of time for grow out at a facility.
While this study demonstrated that the spatial distance between two species of coral cultured together can have an affect on growth and morphology, it is clear that further investigation into the mechanisms that caused the increase in growth for A. pulchra and the long-term effects on polycultured corals is required. This experiment is another step towards refining culturing techniques that will lead ultimately towards the reduction of harvesting wild stock corals for the aquarium trade.
Author Biography
Lee Goldman earned his Masters degree in Marine Biology at the University of Guam, where he also works as a research associate at the College of Natural and Applied Sciences, Guam Aquaculture and Development Training Facility. He will be speaking about this experiment and other research work at the upcoming MACNA XIX in September.
Literature Cited
- Baird, A.H. and Hughes, T.P., 2000. Competitive dominance by tabular corals: an experimental analysis of recruitment and survival of understorey assemblages. J. Exp. Mar. Biol. Ecol. 251, 117-132
- Chornesky, E.A., 1989. Repeated reversals during spatial competition between corals. Ecology 70, 843-855
- Clark, S. and Edwards, A.J., 1995. Coral transplantation as an aid to reef rehabilitation: evaluation of a case study in the Maldive Islands. Coral Reefs 14, 201-213
- Connell, J.H., 1973. Population ecology of reef-building corals. In: Biology and Geology of coral reefs. (O.A. Jones and R. Endean, eds.). Academic Press. pp. 205-246
- Connolly, S.R. and Muko, S., 2003. Space pre-emption, size-dependent competition, and the coexistence of clonal growth forms. Ecology 84, in press.
- Custodio III, H.M. and Yap, H.T., 1997. Skeletal extension rates of Porites cylindrica and Porites rus after transplantation to two depths. Coral Reefs, 16: 267-268
- Dizon, R.M. and Yap, H.T., 2000. Growth differences in Porites cylindrica nubbins transplanted to monospecific and multispecific plots. Proc 9th Int Coral Reef Symp Progr Abstr. pp. 132
- Ellis, S. and E. Ellis, 2002. Recent Advances in Lagoon-based Farming Practices for Eight Species of Commercially Valuable Hard and Soft Corals – A Technical Report. CTSA Publication No. 147. pp. 63
- Ferrier-Pages, C., Witting, J., Tambutte, E., Sebens, K.P., 2004. Effect of natural zooplankton feeding on the skeletal growth of the scleractinian coral Stylophora psitillata. Coral Reefs 22, 229-240
- Goreau, T.F., Goreau, N.I., Yonge, C. M., 1971. Reef corals: Autotrophs or heterotrophs. Biol. Bull. 141, 247-260
- Kato, M., 1987. Mucus-sheet formation and discoloration in the reef-building coral, Porites cylindrica: Effects of altered salinity and temperature. Galaxea 6, 1-16
- Lang, J and Chornesky, E.A., 1990. Competition between scleractinian reef corals – A review of mechanisms and effects. In: Ecosystems of the World (Z. Dubinsky, ed.). Coral Reefs 25, 209-252
- Raymundo, L.J., 2001. Mediation of growth by conspecific neighbors and the effect of site in transplanted fragments of the coral Porites attenuata Nemenzo in the central Philippines. Coral Reefs 20, 263-272
- Rinkevich, B and Loya, Y., 1983. Intraspecific competitive networks in the Red Sea coral Stylophora pistillata. Coral Reefs 1, 161-172
- Rinkevich, B and Loya, Y., 1985. Intraspecific competition in a reef coral: effects on growth and reproduction. Oecologia 66, 100-105
- Romano, S.L., 1990. Long-term effects of interspecific aggression on growth of the reef-building corals Cyphastrea ocellina and Pocillopora damicornis. J. Exp. Mar. Biol. Ecol. 140, 135-146
- Shimek, R.L., 2003. http://reefkeeping.com/issues/2003-09/rs/feature/index.php. Reefkeeping Magazine. Reef Central, LLC
- Smith, L., 2004. Influence of water motion on resistance of corals to high temperatures: Evidence from a field transplant experiment. Master thesis. Univ. of Hawaii at Manoa. pp.19.
- Soong, K. and Chen, T., 2003. Coral transplantation: Regeneration and growth of Acropora fragments in a nursery. Res. Ecol. 11, 62-71
- Tanner, J.E., 1997. Interspecific competition reduces fitness in scleractinian corals. J. Exp. Mar. Biol. Ecol. 214, 19-34
- Wheaton, F.W., 1993. Aquacultural Engineering. Krieger Publishing Company. Malabar, Florida.
- Wild, C., Huettel, M., Klueter, A., Kremb, S.G., Rasheed, M.Y.M., Jergensen, B.B., 2004. Coral mucus functions as an energy carrier and particle trap in the reef ecosystem. Nature 428, 66-70
- Yap, H.T. and Gomez, E.D., 1984. Growth of Acropora pulchra: II Responses of natural and transplanted colonies to temperature and day length. Mar. Biol. 81, 209-215
0 Comments