Where would the reef aquarium hobby be without debate? Everyone has an opinion, and I have certainly embraced certain theories. One such theory is that artificially-produced ultraviolet radiation (UVR) has potentially harmful effects on marine invertebrates. While the evidence of natural ultraviolet bandwidths and intensities is more than enough scientific documentation to prove detrimental effects (see Gleason and Wellington, 1993 and a host of others), there is much less data supporting similar effects of artificial light sources on captive marine invertebrates (but see Masuda, et al., 1993) . I’ve speculated that UVR is potentially detrimental for a number of years now, and have finally worked out an experimental protocol to test the hypothesis.
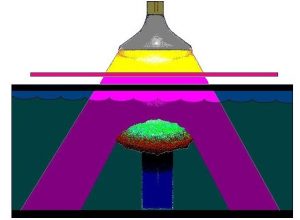
Figure 1: Ultraviolet radiation generated by a mercury vapor lamp is selectively transmitted by a filter that absorbs most visible light energy. The coral used in this experiment is a Montipora capitata. Not shown for clarity is a plywood-styrofoam laminate that insulated the aquarium from heat and rested upon the top of the aquarium.
This article documents the results of an experiment designed to examine the effects of artificially-produced UVR on symbiotic zooxanthellae. We’ll also examine inexpensive ways to test your system and, if necessary, corrective measures one can take.
Procedure
A stony coral, Montipora capitata (formerly known as M. verrucosa, and commonly called the “rice” coral) was legally collected, and maintained at the Natural Energy Laboratory Hawaii Authority (NELHA) complex in an outdoor 379 liter RubberMaid trough. A flow-through of approximately 200 liters per minute maintained chemical and physical parameters very close to those found in the ocean. This coral was allowed to photoadapt to relatively high light (~800 μmol.m2.sec, or ~40,000 lux) for a few months, and then was screened from UVR by a sheet of Lexan Solar acrylic for about 8 weeks.
The coral was transported to a darkened and air conditioned laboratory, where it was placed in an aerated 38 liter aquarium filled with natural seawater. Water motion remained consistent throughout the course of the experiment. See Figure 1. The coral was allowed to dark-adapt for 40 minutes before a PAM chlorophyll fluorometer determined baseline minimum chlorophyll fluorescence (Fo), maximum chlorophyll fluorescence (Fm), variable fluorescence (Fv) and photosynthetic yield of Photosystem II (Fv:Fm).
Originally, a 400-watt 6500K metal halide lamp was planned for use in this experiment, and its PAR would be ‘filtered out’ by using a glass filter that absorbs most visible light and transmits almost only UVR (Spectronics Corp., Westbury, NY). However, the filter while transmitting UVR, also attenuated UV-A by at least 42% (@ 365 nm) and UV-B (@ 310 nm) by 82%, so a stronger ultraviolet source was used (a 300-watt mercury vapor lamp) to obtain amounts of UVR (especially UV-B) consistent with those observed in marine aquaria using certain high intensity lamps.
The element mercury (Hg) is largely responsible for generation of ultraviolet radiation spikes in almost all ‘aquarium’ lamps (including fluorescent, mercury vapor and metal halide lamps). Figures 2, 3 and 4 demonstrate spectral signatures and characteristics of the mercury vapor lamp used during this experiment. A spectrometer, with upgrades for measuring UVR (USB2000, Ocean Optics Inc., Dunedin, FL) , was used to gage the spectral signatures of these lamps. A NIST-traceable digital radiometer (model UVX, UVP, Inc., San Gabriel, CA) measured the UV-A and UV-B output – the two sensors used are most sensitive to UV-A @ 365 nm, and UV-B @ 310 nm. A digital quantum meter (model LI-189, with a submersible 2 pi sensor, Li-Cor, Inc., Lincoln, NB) measured the small amount of visible radiation transmitted by the visible light filter (< 5 μmol.m2.sec).
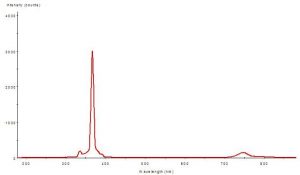
Figure 3: The Montipora capitata specimen was exposed to this light quality – the ‘filtered’ light of the mercury vapor lamp (full spectrum shown in Figure 2). Amplitudes indicate spectral quality only – Figs. 2 and 3 are not to scale.
The Montipora specimen was exposed to ~1,150 microwatts per square centimeter (μWcm2) UV-A and 145 μWcm2 UV-B for 4 hours. Temperature was monitored during this period, and, due to the lab’s air conditioning, fell from 27.8º to 26.7ºC during the course of the 6 hour experiment.
UV-B accounts for 2%; UV-A = 91%, and visible light (400-700 nm) = 7%.
Photosynthetic yield (Fv: Fm) was measured before and after exposure to UVR using a pulse amplitude modulation chlorophyll fluorometer (“Teaching PAM”, Heinz Walz GmbH, Effeltrich, Germany).
Results
Baseline yield and post-exposure yield are shown in Figure 5. If UVR causes photoinhibition, it would be apparent as reduced quantum yield of Photosystem II after sufficient time is allowed for recovery after exposure to UVR. Schreiber (1997) contends that 40 minutes is sufficient time for recovery, and depressed yield at this time will indicate photoinhibition. In this case, yield is depressed even after 60 minutes, strongly suggesting that UVR caused photoinhibition. Yield returned to baseline values overnight (mean yield of 0.524 after 13.5 hours). For an example of quick zooxanthellae recovery from dynamic photoinhibition induced by visible light, see Riddle (2004).
The green dashed line represents the baseline yield (before exposure). After 4 hours exposure to UVR, yield (solid line and plotted against recovery time at bottom) had dropped dramatically and showed no immediate recovery.
Discussion:
In my experiences, most Montipora capitata specimens are light sensitive, and a large pool of previous research conducted by others on this species made it an ideal candidate for this sort of experiment.
Although this coral species can grow in shallow water, it is regarded as a ‘shade tolerant’ coral and is found to depths of 150 feet (Hoover, 1998). Those specimens found in shallow water are often stunted in growth and lack rich coloration (‘zooxanthellae brown’). This coloration is seen in specimens growing in their preferred environments – under ledges, in caves or in deeper water (Coles and Jokiel, 1978).
There are a couple of possibilities as to why UVR inhibited photosynthesis. The zooxanthellae in this coral may have the ability to absorb UV-A, and use it in photochemistry. This should be an area of concern, especially with high output lighting systems. The thought is a little frightening that UV radiation (that is invisible to the human eye and expensive to quantify) could promote saturation, and ultimately photoinhibition, of photosynthesis. It is possible that the zooxanthellae may have been damaged by exposure to UV-B (see Gleason, 1993, and comments below), or that the combined effects of UV-A and UV-B are responsible. In any case, UVR seems to cause the chronic photoinhibition, and we see this evidenced as very slow recovery of photosynthetic yield.
Whether it is UV-A or UV-B is a moot point. UVR certainly seems responsible for this case of photoinhibition.
Don’t Corals Contain Natural Sunscreens?
Not all corals contain significant amounts. Natural UVR ‘sunscreens’ (mycosporine-like amino acids, or MAAs) are not produced by the coral animal at all, and not all ‘zooxanthellae’ ( Symbiodinium species) produce them (see below). Montipora verrucosa ( capitata ) are known to contain the zooxanthellae species S. kawagutii. This species of zooxanthellae is not known to produce MAAs (Iglesias-Prieto and Trench, 1997).
However, shallow-water M. verrucosa specimens in Hawaii are known to contain trivial amounts of mycosporine-like amino acids (MAAs) shinorine and palythine, along with an unknown MAA (Krupp and Blanck, 1995), mycosporine- glycine, porphyra, and palythine. These small amounts of MAAs found in M. capitata tissues are possibly obtained through the corals’ diets.*
Interestingly, the absorption bands of these MAAs closely match the UVR mercury spikes: Palythine (λmax = 360 nm), shinorine (λmax = 334), porphyra 334 (λmax = 334), palythinol (λmax = 332) and mycosporine-glycine (λmax = 310) (Baker, 1995; Kuffner et al., 1995).
Gleason (1993) believes those wavelengths responsible for zooxanthellae damage in Porites corals fall into the range of 310 – 350 nm. This is based on his observations of MAA concentrations in corals transplanted to differing light environments.
Bear in mind that some corals contain multiple zooxanthellae species, however, here’s a quick, abbreviated reference for zooxanthellae types, and their abilities:
Phylotype “A” – Characterized by production of MAAs.
S. microadriaticum. Acclimates to high and low light levels and synthesizes natural ultraviolet radiation sunscreens – MAAs – (even in the absence of UV), but has low tolerance of temperature swings. Protective xanthophylls are produced in super-saturating light intensities. Deane and O’Brien (1981) found Symbiodinium (=_Gymbiodinium_ ) microadriaticum in the clam Tridacna maxima (but view the results of this very early work with some caution). Pochon reports Millepora intricata and an unidentified Millepora species, Porites nigrescens, and an Acropora species also contain clade ‘A’.
S. pilosum. High light adapted (responds poorly to low light levels), tolerates high temperatures swings. Isolated from Zoanthus sociatus. Able to produce protective xanthophylls.
Phylotype “B” – Characterized by tolerance of high light levels and no MAA production.
Symbiodinium species. Isolated from the stony coral Pocillopora damicornis (but see below) and Plesiastrea verispora.
Tolerates high light, does not synthesize MAAs and tolerates high temperatures.
S. pulchorum. Tolerates high light, does not synthesize MAAs and has a low tolerance of temperature swings.
Phylotype “C” – Characterized by tolerance of low light levels and no MAA production.
Symbiodinium kawagutii and other species. No protective xanthophylls are produced as a response to super-saturating irradiance (Iglesias-Prieto and Trench, 1997). Isolated from the Giant Clam, Hippopus hippopus, “Mushroom Corals_” Discosoma sanctithomae_, and stony corals Montipora verrucosa (capitata) and Meandrina meandrites. Also found in some eastern Pacific specimens of certain species, including Pocillopora damicornis, P. elegans, P. eydouxi, Psammocora stellata, P. superficialis, Gardineroseris planulata, Porites lobata, P. panamensis, Pavona varians, P. gigantean, P. clavus and Montastraea cavernosa (Baker and Rowan, 1997). Interestingly, the difficult coral genus Alveopora contains clade “C” (Rodriguez-Lanetty et al., 2000, and see below).
Phylotype “D”
Chen et al., 2003, found this clade within a high latitude coral ( Oulastrea crispata ) and Goniastrea aspera inhabiting marginal sites (extreme temperatures, turbidity and irradiance) This zooxanthellae is thus considered extremely stress tolerant.
Corals containing clade “D” (specifically D2) are Goniopora fruticosa, Pavona decusata, Pocillopora elegans, and several Acropora species (Pochon et al., 2001).
Phylotype “E”
None of the references available to me show this clade to be found in corals.
Phylotype “F”
Normally found in foraminiferans, researchers were surprised when it was found in isolated ‘daisy coral’ specimens ( Alveopora japonica ) in Korea. (Rodriguez-Lanetty et al., 2000).
Symbiont populations tend to follow Fisher log-normal distribution patterns characterized by ‘generalist’ zooxanthellae (common) and rare zooxanthellae (‘specialists’) hosted by specific coral species (Pochon et al., 2001).
There is a terrific amount of research available on clades of Symbiodinium-like dinoflagellates. I’ll expound on this subject in a following article.
*In contrast, Kinzie (1993) found this coral species in Hawaii to have modulating MAA concentrations (measured as absorbance at 320 nm) according to environmental conditions. Kinzie’s work, while interesting, has been usurped by later, more sophisticated works. However, I use the reference to document potential differences among this coral species (and further justification for shielding the coral from UVR prior to the experiment).
How Can I Tell if My Corals Have MAAs?
You can’t simply by looking at them. You’re in good shape if you are a chemist or geneticist and have access to a well-stocked laboratory and possess necessary skills to perform and interpret results of HPLC or other testing.
My Coral is Fluorescent Green – Doesn’t This Protect the Coral from UVR?
Green fluorescent proteins can absorb some UVR (but mostly violet and blue wavelengths) and fluoresce it as visible light. However, the process is not efficient enough to protect the coral from UVR. See Mazel et al., 2003 for details.
Aren’t Mogul-base (Single-Ended) Metal Halide Lamps Shielded For UVR?
Yes, and No. The outer glass envelope surrounding the inner arc tube attenuates UVR but very poorly in many cases. See Figure 6. Compare these with Figure 7 – many lamps produce sizeable areas of high-intensity UVR, sometimes more intense than natural sunlight!
- Lamp A = 400-watt 10,000K mogul base (single ended) metal halide lamp in pendent fixture, 9.5″ from sensor, no shield
- Lamp B = Lamp A in different pendent fixture, 9.5″ from sensor, no shield
- Lamp C = 400-watt 6,500K mogul base metal halide lamp in pendent fixture, 9.5″ from sensor, no shield
- Lamp D = Lamp A in horizontal polished aluminum fixture, 9.5″ from sensor, no shield
- Lamp E = 175-watt 4,000K metal halide in horizontal polished aluminum fixture, 9.5″, no shield
- Lamp F = 150-watt DE metal halide with UV shield, 9.5″ from the sensor
- Lamps G = 2-96-watt power compact fluorescent lamps, 9.5″ from sensor, no shield
Not shown is the lamp that produced 11,500 μWcm2 UV-A and 566 μWcm2 UV-B!
Are the Results Applicable to Other Lamps?
The element mercury (Hg) produces specific line spectra and is found in metal halide, mercury vapor and fluorescent lamps (including Power Compacts). These spectral spikes are a reliable signature of Hg, and are seen at these UVR wavelengths: 248, 280, 297, 313, 334 and 365 nm. There are many variables involved (such as glass envelope quality, amount of mercury, etc.) but, generally, all ‘aquarium’ lamps emit UVR at 365 nm. Some – not all – emit measurable, and potentially damaging, amounts of UV-B radiation as well.
The Area Affected By UVR Is Small, Isn’t It?
Not necessarily. Figure 8 demonstrates an example of high – but not unrealistic – ‘footprint’ of UV-B falling upon a surface. The lamp was a 400-watt 10,000K in a pendent luminaire suspended 9″ above the surface. The ‘hot spot’ of UVR equaling, or exceeding that used in this PAM/photoinhibition experiment is roughly 12″ in diameter.
Notice that the lamp geometry (probably the ‘dimple’ in the end of the lamp) combined with the characteristics of the luminaire create an irregular (and generally unpredictable) UVR distribution pattern.
But the Aquarium Water Removes UVR by Absorbing It – Right?
Not very effectively – we’re dealing with high output lamps and shallow basins. Figure 9 shows UVR transmission in ‘clear’ aquarium water (APHA Color = 0 @ 8.11 pH). This was a commercially available artificial seawater mixed with water treated by the reverse osmosis process, and allowed to ‘age’ for a day so ‘cloudiness’ would disappear. Transmitted at a water depth of 15.5″ are 34% and 29% UV-A and UV-B, respectively.
But ‘Real’ Aquarium Water Contains Some Organic Compounds That Absorb UV
True enough. Figure 10 shows UVR transmission in aquarium water that is extremely yellow, and represents an aquarium without foam fractionation, GAC or routine water changes (the water is really yellow: APHA Color = 10 @ 8.11 pH. See Kirk, 1983). Transmitted at a water depth of 15.5″ are 29% and 22% UV-A and UV-B, respectively.
As a footnote, these experiments were conducted without water surface agitation. Another experiment showed that the lensing effect of small waves (that is, ‘flicker’ or ‘glitter lines’) focused the UVR into concentrated pulses. The response of the sensor was not fast enough to accurately gage the pulse intensity, although ‘pulsed’ UVR was measured as much as 12% higher than ‘still surface’ UVR. In his 1976 work, Jerlov reports ‘disturbing’ pulses of long-wave radiation due to focusing by passing waves – this phenomenon probably happens in aquaria as well.
The Effects of UVR in This Experiment Were Short-term. So What?
Corals have a limited number of responses to UVR. They can cope with UVR and expend energy for repair, they can adapt by developing (or obtaining through diet) MAAs, or they can avoid UVR.
Hobbyists, as masters of these unique ecosystems we call reef aquaria, can choose how their corals are treated. Some corals will be just fine in coping with relatively high UVR doses (which has led to the myth that UVR is harmless). Others will offer silent testament to the effects of UVR, and may grow relatively slowly or perhaps not at all. We do not know the long term effects of artificially-generated UVR on coral health. Some could die as a result of prolonged exposure.
Gleason (1993) shows the ability of Porites astreoides to adapt to varying degrees of UVR. Even after an adaptation period of ~3 months, some Porites and their zooxanthellae failed to adapt to the higher UV environment and demonstrated less growth than ‘adapted’ corals.
Why Was the Coral Screened from UVR Before the Experiment?
The idea was to suddenly subject a coral to high intensity UVR, such as would be encountered when a coral is transferred from low to high UV environments.
Doesn’t UVR Promote Coloration in Corals?
There is a lot of work to be done in this area, and some interesting coloration shifts have been noted in corals exposed to UVR. But it has not been determined if the coloration is due to UVR or simply an slight increase in PAR (especially violet and blue wavelengths) due to the lamp’s UV shield being removed.
Gleason (1993) suggests green Porites specimens are dominate (over brown- colored corals of the same species) in shallow water however he believed that the green coloration plays no photoprotective role – an observation expounded upon by Mazel et al., 2003. See this website for further comments about UVR, and a couple of photos of colorful stony corals grown under practically non- existent UVR: www.masla.com/reef/uvlighting.html.
Can I Check My Lamps for UVR?
Since checking corals for presence of natural sunscreens is impractical for most, the next best thing is checking your lamps for UVR. Fortunately, there is an inexpensive alternative to radiometers and UV meters. It is a ‘UV card’ – a plastic rectangle the size of a credit card which has a strip of UV- sensitive material. This strip qualifies the presence of UV radiation and these are distributed to guests by some of the upper-end resorts here in Hawaii. They are also available through various suppliers. I got mine through Edmund Scientific for $4.95 plus shipping. See www.scientificsonline.com, and search for item #3120200. (See product photo in Figure 11.) Though not really a scientific instrument, it sure comes in handy for on-the-spot checks of UVR without all the fuss of calibrating a NIST-traceable radiometer.
It was curious about the sensitivity of the material on this card, and investigated a $5 item with $5,000 worth of equipment. It’s true – the strip on the card is sensitive to only UVR.
Hold the card at the same distance from your lamp as it is from the water surface.
UVR is probably not a concern with most fluorescent lamps (unless they are specifically made to generate UVR). Based on experience I would check any metal halide lamp, any mercury vapor lamp, and Power Compact or VHO fluorescent lamps exceeding combined wattages of ~400. I would suggest shielding your lamps if the indicator strip suggests a ‘Moderate’ amount of UV.
Store this card in a protective envelope in a dry, dark place when done.
OK – My Lamps Are Generating UVR. How Do I Get Rid of It?
There are many choices for UV filters and, even better, are widely available and relatively inexpensive. Your local hardware store probably has sheet acrylic material (sold as ‘safety’ glazing). Any glazing marked as ‘UV Protective’ will work. Common brand names are Lexan, Lexan Solar and Lucite. Thickness isn’t critical (1/8″ or 3/32″ is fine), but orientation is – some acrylics are coated with UVR-absorbing compounds only on one side (the directions will tell you which). Avoid hardware store window glass – if the glass is green when viewed edge-wise, don’t use it (3/16″ ‘green’ window glazing transmits 75% UV-A and 3% UV-B). If in doubt, use the ‘UV card’ mentioned above to check the material in full sunlight.
What Are the Trade-offs of UVR Shielding?
Expect the acrylic material to attenuate photosynthetic active radiation (PAR) by 6 to 14% (depending upon brand of acrylic). As a general rule, acrylic tends to absorb most energy < 390 nm, but also attenuates violet and blue wavelengths slightly more than other visible wavelengths. This weakening is not apparent to the human eye (mine anyway) and only a meter can gage the weakening effect. Maintenance is a bit higher, since salt spray will have to be cleaned off the shield (but is much easier than scraping dried salts off a metal halide lamp).
Closing Comments
One may wonder under what set of circumstances corals could be damaged by UVR. Remember that MAA concentrations, if present at all, are variable and related to environmental conditions, including water velocity (Jokiel et al., 1995), UVR intensity (UV-A and UV-B; Baker 1995) and/or water depth (Dunlap et al., 1986).
These are a few reasons to shield your lamps and/or check for UVR:
- Any number of environmental factors is greatly altered once a coral is collected and held in captivity before you purchase it. Therefore, MAA concentrations could change – likely drop – dramatically.
- Some corals may never acquire appropriate MAAs and/or adapt to a high UVR environment.
- Captive-grown corals could be grown in UVR-free conditions (ask your supplier if you insist on using ‘naked’ lamps!).
- Since light and UVR production decrease with lamp age, one could speculate that coral MAA concentrations decrease as well.
- It is apparent from product testing that acrylic UVR shields lose effectiveness over a period of time, and should be replaced periodically. Until we have a better understanding of the effects of environmental conditions on UVR-absorbing substances, it’s probably not a bad idea to check your shielded lamps monthly.
- As Figure 6 shows, using the same lamp in a more efficient reflector could greatly increase the UVR dose.
- The outer glass envelope could fail and expose the captive animals to significant increases of UVR (including dangerous UV-C wavelengths).
- Lowering the fixture (luminaire) only a few inches could easily double the amount of UVR.
- And, of course, one might simply use another type or brand of lamp.
- Vivid fluorescent/reflective coral coloration, in many cases, is possible without exposure to UVR.
- Introductory use of activated carbon, protein skimming (or upgrade) or drastic water change could increase the transmission of UVR (see Bingman, 1995).
As final thoughts: We tend to think of our aquaria as being ‘coral-centric’, since high intensity lighting is mainly for the corals’ and zooxanthellae sake. Perhaps we should appreciate these systems more for the artificial ecosystems they are, and think of the impact of UVR on inhabitants other than corals. Some of the fishes maintained in aquaria (such as damelfish species Dascyllus, Pomacentrus and Chromis ) have the ability to ‘see’ into the UV range (Shashir, 1995). It is thought that this special vision enables planktivorous fishes a competitive edge while feeding. It is also believed that fishes active in shallow, brightly lighted waters may pay the price of retina damage for this vision. Some fishes are known to possess protective MAAs in their eye lenses (Dunlap et al., 1989); one has to wonder if these pigments are lost and gained in response to environmental conditions, or how much a role diet plays, and how sudden exposure to unprotected eyes
(those not containing MAAs) could affect fishes’ vision. As mentioned, MAA production is known to occur only in plants and fishes could possibly need an MAA-enriched diet (algal-based) to maintain these pigments within their eyes.
Also of concern is the possible impact on other invertebrates. Lesser (1995) and Culotta (1994) relay the story of Max Bothwell, an ecologist involved in investigation of UVR effects on freshwater periphyton in Canada. He determined that UVR resulted in short-term decrease of periphyton biomass (and reduced rates of photosynthesis). Serendipitously, the experiment was allowed to continue, and Bothwell found that periphyton biomass increased. Why? Because UVR eventually eliminated an important periphyton predator, and this factor upset the entire ecology of the experimental vessels.
Does the same happen in our aquaria? One has to wonder of the effects of UVR on nocturnal snails if they’re unfortunate to be directly under the lamps at start-up. The fact is we’re just beginning to understand the effects of artificially produced UVR and effects of doses on our captive reefs. Still, we should view these results with some caution and avoid sweeping generalizations. However, if there is one coral species sensitive to the effects of artificially produced UVR, there are surely others. Until we have a much better idea of UVR and its effects – shield those lamps – you won’t be sorry.
Acknowledgements
Many thanks to Charlie Mazel of NightSea, Inc. (www.nightsea.com) for supplying the Spectronics light filter.
References
- Baker, A., 1995. Solar UV-A inhibition of planula larvae in the reef- building coral Pocillopora damicornis. In: Ultraviolet Radiation and Coral Reefs. D. Gulko and P.L. Jokiel, eds. HIMB Tech. Report #41: 149-163.
- Baker, A. and R. Rowan, 1997. Diversity of symbiotic dinoflagellates (zooxanthellae) in scleractinian corals of the Caribbean and Eastern Pacific. Proc. 8th Int. Coral Reef Symp., Panama. 2: 1301 – 1306.
- Banaszak, A., T. LaJeunesse and R. Trench, 2000. The synthesis of mycosporine-like amino acids (MAAs) by cultured, symbiotic dinoflagellates. J. Exp. Mar. Biol. Ecol., 249: 219-233.
- Bingman, C., 1995. The effect of activated carbon treatment on the transmission of visible and UV light through aquarium water. Part 1: Time- course of activated carbon treatment and biological effects. Aquarium Frontiers. 2, 3: 5-19.
- Chen, C., K. Lam, Y. Nakano and W-s. Tsai, 2003. A stable association of the stress-tolerant zooxanthellae, Symbiodinium clade D, with the low- temperature-tolerant coral Oulastrea crispata (Scleractinia: Faviidae) in subtropical non-reefal coral communities. Zool. Stud., 42(4): 540-550.
- Coles, S.L. and P.L. Jokiel, 1978. Synergistic effects of temperature, salinity and light on the hermatypic Montipora verrucosa. Mar. Biol., 48: 187-195.
- Cullota, E., 1994. UV-B effects: Bad for insect larvae means good for algae. Science, 265: 30.
- Deane, E. and R. O’Brien, 1981. Uptake of phosphate by symbiotic and free- living dinoflagellates. Arch. Microbiol. 128: 307-310.
- Dunlap, W., Chalker, B. and J. Oliver, 1986. Bathymetric adaptations of reef-building corals at Davies Reef, GBR, Australia. III. UV-B absorbing compounds. J. Exp. Mar. Biol. Ecol., 104: 239-248.
- Gleason, D.F. and G.M. Wellington, 1993. The intensities of ultraviolet radiation that induce bleaching of a Caribbean coral. Proc. 7th Int. Coral Reef Symp., Guam. 1: 71. (Abstract).
- Gleason, D.F., 1993. Different effects of ultraviolet radiation on green and brown morphs of the Caribbean coral Porites astreoides. Limnol. Oceanogr., 38(7): 1452-1463.
- Grottoli-Everett, A.G. and L.B. Kuffner, 1995. Uneven bleaching within the colonies of the Hawaiian coral Montipora verrucosa. In: Ultraviolet Radiation and Coral Reefs. D. Gulko and P.L. Jokiel, eds. HIMB Tech. Report 41: 115-120.
- Hoover, J., 1998. Hawaii’s Sea Creatures: A Guide to Hawaii’s Marine Invertebrates. Mutual Publishing, Honolulu.
- Iglesias-Prieto, R. and R.K. Trench, 1997. Photoadaptation, photoacclimation and niche diversification in invertebrate-dinoflagellate symbioses. Proc. 8th Int. Coral Reef Symp., Panama. 2: 1319-1324.
- Jokiel, P.L., M.P. Lesser and M.E. Ondrusek, 1995. UV-absorbing compounds in the coral Pocillopora damicornis: interaction effects of light, water flow and UV radiation. In: Ultraviolet Radiation and Coral Reefs. D. Gulko and P.L. Jokiel, eds. HIMB Tech. Report #41: 87.
- Jerlov, N., 1976. Marine Optics. Elsevier Oceanography Series, Elsevier Sci. Publ. Co., New York. 231 pp.
- Kinzie, R.A., P.L. Jokiel and R. York, 1984. Effects of light of altered spectral composition on coral zooxanthellae associations and on zooxanthellae in vitro. Mar. Biol., 78:239-248.
- Kinzie, R.A. and T. Hunter, 1987. Effect of light quality on photosynthesis of the reef coral Montipora verrucosa. Mar. Biol., 94: 95-109.
- Kinzie, R.A., 1993. Effects of ambient levels of solar ultraviolet radiation on zooxanthellae and photosynthesis of the reef coral Montipora verrucosa. Mar. Biol., 116:319-327.
- Kirk, J.T.O., 1983. Light and Photosynthesis in Aquatic Ecosystems. Cambridge University Press, Cambridge. 401 pp.
- Krupp, D.A. and J. Blanck, 1995. Preliminary report on the occurrence of mycosporine-like amino acids in the eggs of the Hawaiian scleractinian corals Montipora verrucosa and Fungia scutaria. In: Ultraviolet Radiation and Coral Reefs. D. Gulko and P.L. Jokiel, eds. HIMB Tech. Report #41: 129-134.
- Kuffner, I.B., M.E. Ondrusek and M.P. Lesser, 1995. Distribution of mycosporine-like amino acids in the tissues of Hawaiian scleractinia: a depth profile. In: Ultraviolet Radiation and Coral Reefs. D. Gulko and P.L. Jokiel, eds. HIMB Tech. Report #41: 77-86.
- Rodriguez-Lanetty, M., H. Cha and J. Song, 2000. Genetic diversity of symbiotic dinoflagellates associated with anthozoans from Korean waters. Proc. 9th Int. Coral Reef Symp., Bali. 1: 163-166.
- Lesser, M., 1995. Oh no, not another workshop: A summary of previous UV workshops. In: Ultraviolet Radiation and Coral Reefs. D. Gulko and P.L. Jokiel, eds. HIMB Tech. Report #41: 13-14.
- Masuda, K., M. Goto, T. Maruyama and S. Miyachi, 1993. Adaptation of solitary corals and their zooxanthellae to low light and UV radiation. Mar. Biol., 117: 685-691.
- Mazel, C., M. Lesser, M. Gorbunov, T. Barry, J. Farrell, K. Wyman and P. Falkowski, 2003. Green fluorescent proteins in Caribbean corals. Limnol. Oceanogr., 48(1, part 2), 402-411.
- Pochon, X., J. Pawlowski, L. Zaninetti and R. Rowan, 2001. High genetic diversity and relative specificity among Symbiodinium-like endosymbiotic dinoflagellates in soritid foraminiferans. Mar. Biol., 139: 1069-1078.
- Schreiber, U., 1997. Chlorophyll Fluorescence and Photosynthetic Energy Conversion. Heinz Walz GmbH, Effleltrich. 73 pp.
- Shashir, N., 1995. UV vision by marine animals: Mainly questions. In: Ultraviolet Radiation and Coral Reefs. D. Gulko and P.L. Jokiel, eds. HIMB Tech. Report #41: 201-206.
0 Comments