This series is a much needed update to articles originally prepared in 1991, and published in Freshwater and Marine Aquariums (FAMA) in 1993 and 1994. At that time, the necessity of feeding by marine invertebrates was a hotly debated issue. Although research scientists had established that symbiotic algae (Symbiodinium spp.) could the supply most if not all of the daily carbon requirements of the invertebrate host, this was construed by many to mean that feeding wasn’t necessary, hence the necessity supplemental feeding of corals in captivity was much in doubt by many.
Feeding captive animals stirs a primal response within us, and we realize this at an early age. I fondly recall visits to the Atlanta zoo when I was a child and how I wanted to buy a small package of fish to feed the seals held in captivity there. A careful aim and throw delivered a fish into the tank and I imagined I squealed with delight when the torpedo-like seal gobbled up his lunch. Sharing of food gives us a positive feeling and provides health to the recipient. This feeling follows us into later years. Dating couples might follow an ancient ritual where food is a gift from one to the other. In older days (and unfortunately, many places in the world today) sharing of food might be the difference of life and death. In any case, the ritual of food sharing establishes bonds and our feelings might progress from caring to loving. And this trait follows us for the rest of our years. So, it is only natural that we take care to provide sustenance to our pets. We might fret over what to feed and how much. When trips call us away for an extended period of time, we locate a reliable caregiver for our fishes and invertebrates. We coach this person on how to provide for animals within the glass box.
Every decade since the 1960’s has seen advances made in the husbandry of marine invertebrates in closed system aquaria. All-glass tanks and undergravel filters revolutionized the hobby in the mid-60’s. Judging from advertisements in Straughan’s Salt Water Aquarium and Siegel’s Marine Aquarist in the 1970’s there was an interest in protein skimmers, ozone generators, advanced water quality testing kits, and so on. Robert P.L. Straughan also included a short chapter on captive corals in his 1964 book The Salt Water Aquarium in the Home, yet practical reef keeping in the home would remain decades away. A resurgence in popularity of advanced filtration (trickle filters) and home application of industrial lighting products in the 1980’s (largely due to George Smit’s series in Freshwater and Marine Aquariums) made the ‘mini-reef’ trendy in North America. Many animals once thought impossible to maintain in captivity were thriving, and reports of soft- and stony-corals reproducing in captivity were heard, although infrequently.
Some corals were now considered ‘easy’ to keep, including the Elegance coral (Catalaphyllia), Leather coral (Sarcophyton) and Star Polyps (Clavularia), but some were not (including some Goniopora species). Granted, some Goniopora specimens would survive for a few months, and perhaps as long as a year or so before dying. Noted European authors, such as Peter Wilkens, despaired about this coral’s short life expectancy. The trend would usually follow this course – the Goniopora would appear healthy for a few weeks, but then begin to shrink in size and failing to expand. Holes would appear in its tissue with death following quickly.
Times changed and companies researched the nutritional needs of corals and provided foods said to meet the full nutritional requirements of corals. These foods’ packages spout claims such as ‘high protein content’, ‘bio-available amino acids’ and ‘provides omega-3 and -6 fatty acids.’ What do these claims mean, and do they have any basis in fact?
These articles will explore these issues. But feeding corals with proper foods is only a start. Many things act in synergy, and we must consider other factors such as proper lighting and water motion. Temperature is critical for reasons many hobbyists might not suspect. Water chemistry is important since it provides many macro- and micro-elements for general overall health and building of skeletal structures. In nature water motion delivers both dissolved and particulate foods, light energy drives photosynthesis in zooxanthellae where nutrition is produced for both the symbiont and coral host. Hence, proper feeding is dependent upon practically every parameter within a hobbyist’s control. A broken link in the chain spells danger and potential disaster.
The information in these articles must be presented in an architectural manner. We should start at a molecular level, and this will provide the foundation on which other factors build. Only in this manner can be hope to understand (if only incompletely) not only what our corals require but how we can provide it. We can then feed our captive invertebrates and our primal need to provide nutriment is satisfied. Our animals benefit and they thrive, grow, and reproduce. In a way, this bond between the aquarist and captive animal is a symbiosis where ancient needs are satisfied.
With this spiel complete, we’ll being our examination at an elemental level – a foundation – and we’ll proceed from there.
What Is the Coral Animal Made Of?
In our arrogance, we consider corals to be primitive animals, yet they are amazing complex.
Corals could be described as, by a term I believe was used in some Star Trek movie, carbon-based units. They are organic beings possessing varied biosynthetic pathways, with many important in nutritional aspects.
Like all living organisms, corals are composed of atoms of carbon, oxygen, and hydrogen. Nature adds nitrogen to the mix, along with phosphorus, sulfur, and others. To carry our biochemistry class a bit further, corals are composed of proteins, lipids including fats, oils, waxes, along with carbohydrates, and minerals in a measured manner. See Figure 1.
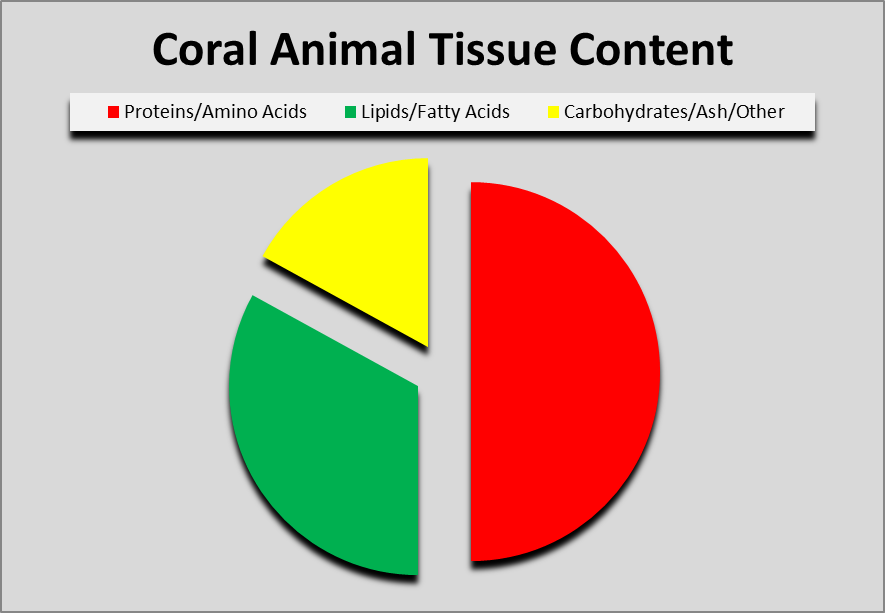
Figure 1. Proteins account for about ½ of a coral’s dry weight, with fats following at about 1/3. Carbohydrates, non-volatile minerals (ash), and undescribed components make up the rest.
As a general rule, proteins make up for 50%, of coral tissue dry weight. Proteins are made of amino acids consisting of carbon, hydrogen, nitrogen with perhaps a little sulfur thrown in. Some amino acids are synthesized by corals, while others are not, and these are said to be ‘essential’ amino acids. Essential amino acids will be examined in this series.
Lipids acting a energy reserves, or structural compounds, are about a third (but sometimes more) of coral tissue dy weight. Fats are made of fatty acids, made of carbon, hydrogen, and oxygen. Like amino acids, the coral animal can make some fatty acids while others – essential fatty acids – must be obtained from symbiotic zooxanthellae or through feeding. Fatty acids and lipids will be the subject of a future article.
Carbohydrates – Hydrates of carbons, such as sugars and alcohols, are a major portion of coral tissue. These must be received from zooxanthellae, or through feeding.
Ash – Non-volatile inorganic compounds, composed of minerals, salts, etc.
We know well that many coral animals are able to build skeletons made chiefly of calcium, magnesium, strontium, and other metals. These materials are absorbed from the water column. Water motion plays an important part in their delivery. Skeleton production (skeletogenesis) and content will be the subject of an installment in this series.
Autotrophy versus Heterotrophy
Coral nutrition becomes a rather cloudy issue when we consider the relationship between symbiotic zooxanthellae and the coral animal. The zooxanthellae (Symbiodinium spp.) are, when conditions are proper, autotrophic meaning they can produce all the nutritional substances required for them to live. The coral animal on the other hand is heterotrophic meaning it has to obtain nutriment through feeding. Symbiodinium provide some nutrition to the coral animal through a process called translocation, and the plot thickens. Figure 2 shows the importance of photosynthesis and its translocated products.

Figure 2. Photosynthesis in zooxanthellae and sharing of the products produced through the photosynthetic process provides important nutrition to the coral animal. From Sorokin, 1981.
It is generally agreed that zooxanthellae translocate enough organic carbon (sugars, carbohydrates, etc.) to the coral host and this was the basis of debate among hobbyists many years ago about the necessity of feeding corals in captivity. Organic carbon alone will not sustain the coral animal for an extended period any more than a carbohydrate diet (such as sugar water or, even better, chocolate) would sustain a human being.
The translocation of carbohydrates from zooxanthellae to the coral animal is not a bad thing however. Carbohydrates can be used to produce lipids and proteins. The questions then become those of which lipids and proteins can be produced by the coral animal (regardless of the source of the precursors), which must be obtained through feeding, and, of course, the quantity of the substances.
With this said, it would seem the issue boils down to what and how much to feed. If the coral does not contain symbiotic zooxanthellae, these issues become critical. Unless efforts are made to manually feed the coral (such as non-zooxanthellae ‘sun corals’ – Tubastrea species), consideration about proper water flow to deliver the food sources is an issue. If the coral contains zooxanthellae, then their ability to produce substances that are translocated (certain amino acids, lipids, and carbohydrates) is dependent upon proper lighting (intensity and spectrum) and, hence, photosynthesis (as well as proper water flow.)
Corals’ Symbiotic Dinoflagellates – Symbiodinium
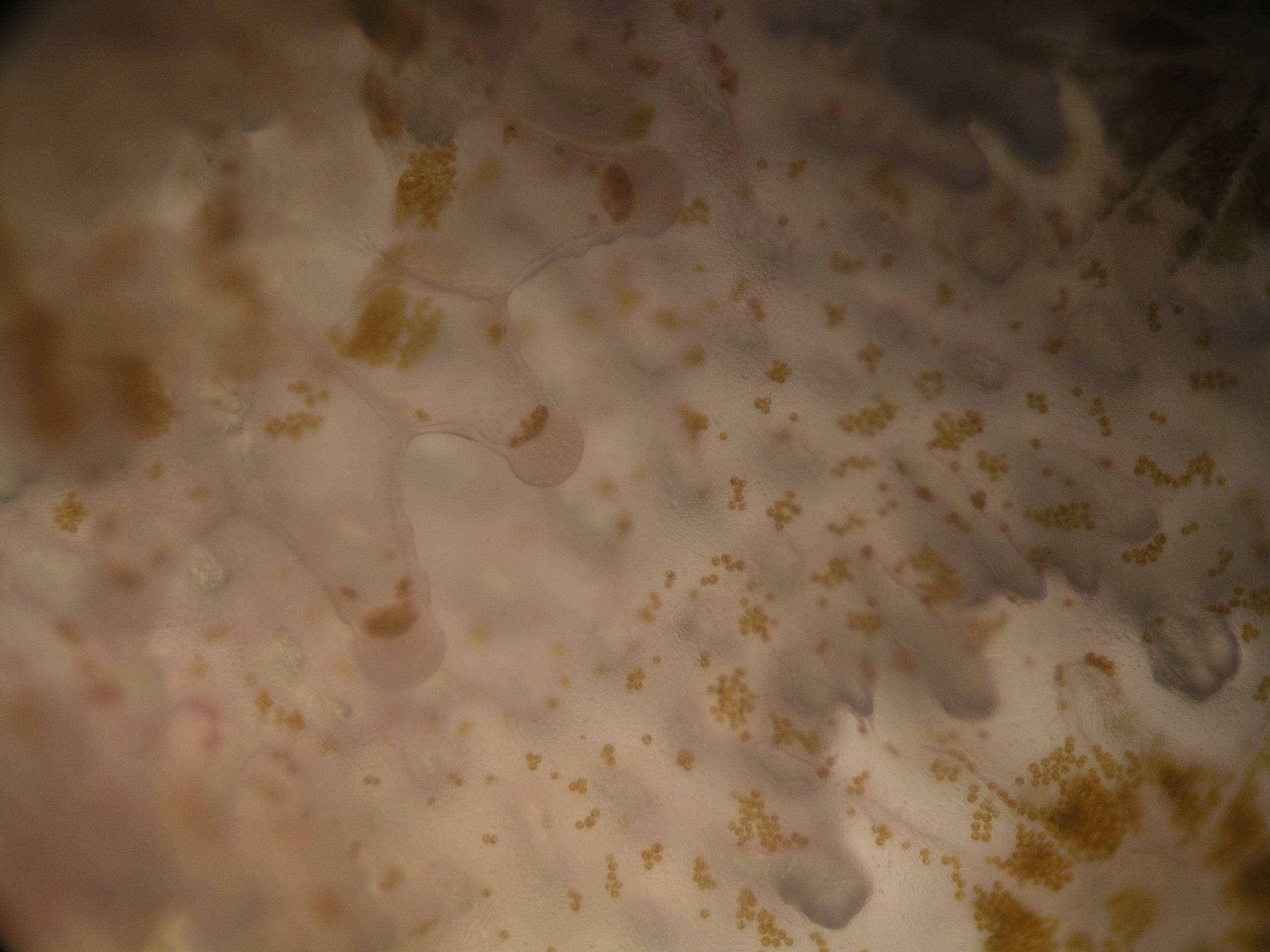
Figure 4. Hundreds of Symbiodinium (zooxanthellae) within the tissues of a Hawaiian Pocillopora damicornis stony coral. Photo by the author.
Corals and algae have co-evolved and possess a symbiotic relationship with the genus Symbiodinium. These dinoflagellates are rather demanding, and without their needs being met (especially when light intensity becomes very low), they can die, or possibly become parasitic. Hence, we, as hobbyists, must meet their requirements for light of proper intensity and spectrum and nutritional requirements. Yet proper lighting in and of itself is practically useless unless water motion meets minimal requirements.
Corals provide zooxanthellae protection in the form of housing, and their waste products can act as fertilizers. In return, zooxanthellae produce and provide (translocate) amino acids, lipids, carbohydrates, and possibly vitamins. See Figures 5 and 6.
Feeding Zooxanthellae
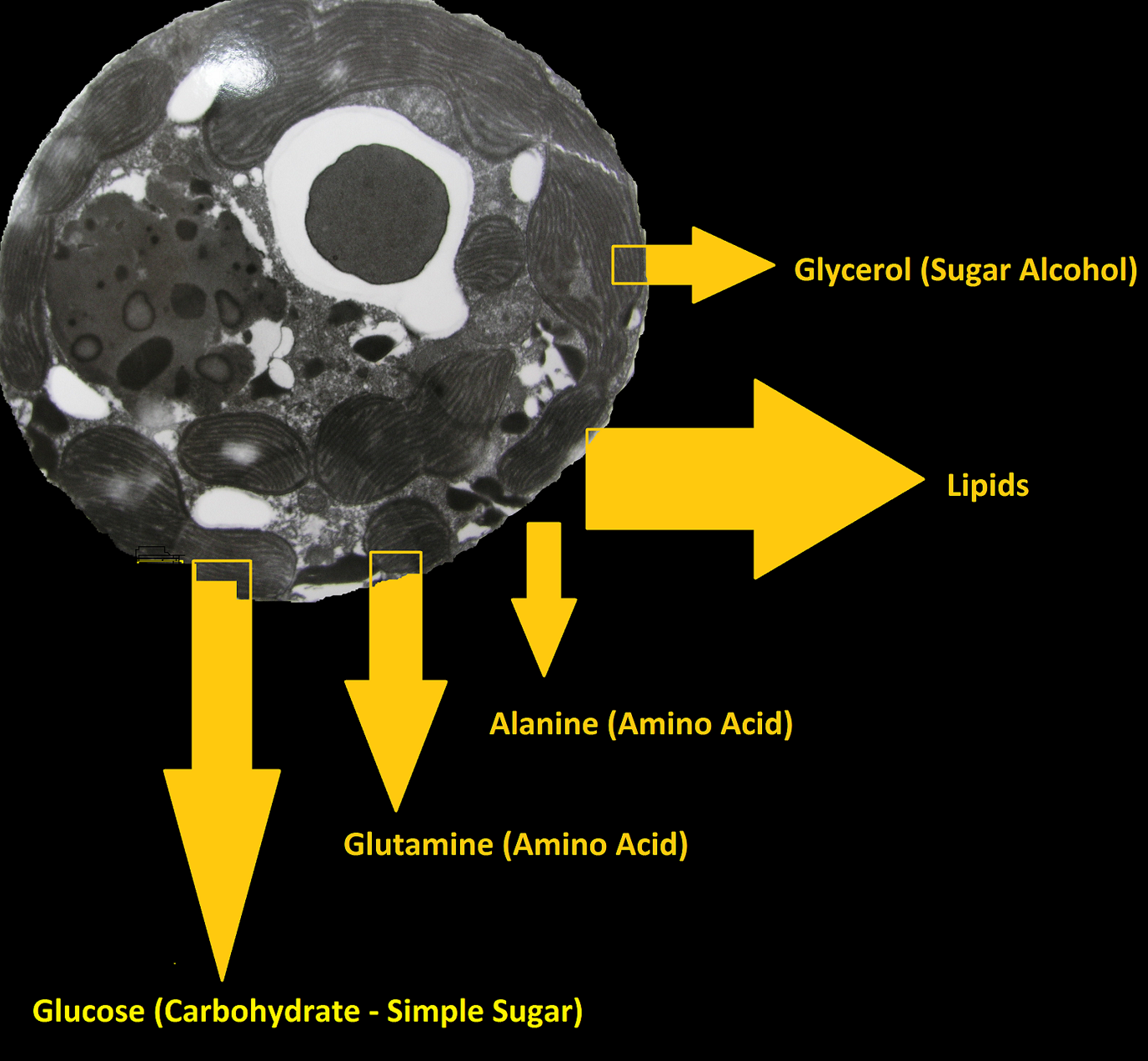
Figure 5. Zooxanthellae produce many organic compounds from substances such as ammonia and bicarbonate. Some of these compounds are shared (translocated) with the coral animal.
Zooxanthellae must be properly cared for in order that they provide nutriment to their host invertebrate. They must be properly fed and correct levels of illumination must be provided.
As with any plant and algae, Symbiodinium spp. have nutritional requirements, and they are not vastly different from terrestrial plants.
A glance at any bag of fertilizer intended for house plants or lawns will reveal the macronutrient levels of the contents. This is known as the NPK number, where N stands for nitrogen, P for phosphorus, and K for potassium. These are the major macronutrients required for plant growth. Other macronutrients include calcium, magnesium and sulfur. Micronutrients for proper zooxanthellae health include copper, iron, manganese, molybdenum, zinc, and nickel, with a possibility that other elements (such as boron and cobalt) are also required.
Bear in mind that these macro- and micronutrients are required by the host animal as well.
Macronutrients
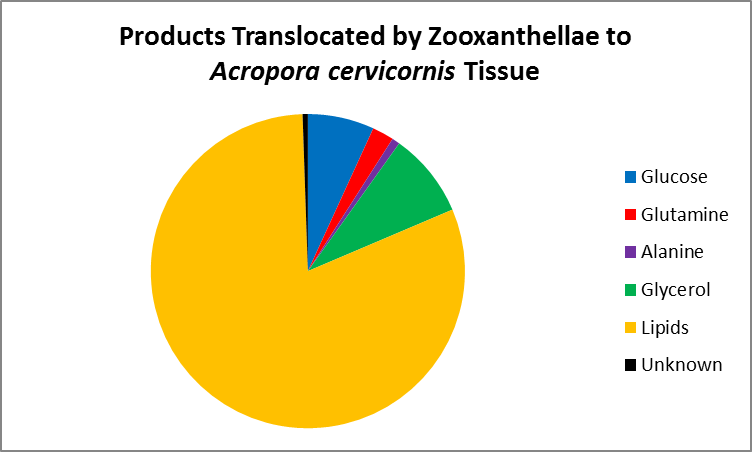
Figure 6. This chart expounds on the information presented in Figure 5. Lipids account for most of the products shared, followed by glycerol and glucose (sugar alcohol and simple sugar, respectively), the amino acids glutamine and alanine, and lastly, unknown subtances. From Pearse and Muscatine, (1971).
For the purposes of this article, we’ll consider those macronutrients for zooxanthellae health, growth, and reproduction as nitrogen, potassium, and phosphorus. I’ve included carbon as well.
Carbon
In terrestrial plants, the source of carbon for photosynthesis is carbon dioxide gas. In marine aquatic environments, little carbon dioxide is usually available, so another inorganic carbon source is used – carbonate (in the form of alkalinity.) Zooxanthellae can also utilize what little carbon dioxide is respired by the coral host.
Photopigments found in zooxanthellae contain relatively large amounts of carbon. An example is Peridinin, a major accessory pigment found in dinoflagellates:
Peridinin: (C39H50O7)
In addition to zooxanthellae growth and reproduction, carbon is also used in the synthesis of proteins, carbohydrates, and lipids that may be transferred (translocated) to the invertebrate host.
Nitrogen
Nitrogen, in the form of ammonium (NH4+) is the most important source of inorganic nitrogen for primary producers (plants, algae, zooxanthellae; Swanson and Hoegh-Guldberg, 1998.) At pH values seen in the ocean and well-maintained aquaria, ammonium (as opposed to ammonia – NH3+) is the prevalent form of inorganic nitrogen. See Figure 7.
Proteins, in general, contain 16% nitrogen (Brown, 1931.) The nitrogen content of the major zooxanthellae photopigment (chlorophyll a: C55H72N4O5Mg) is about 6% nitrogen by weight. Coral fluorescent proteins (and non-fluorescent chromoproteins) contain substantial amounts of nitrogen. See Figure 8.
Other Sources of Ammonium
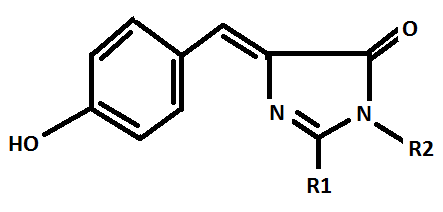
Figure 8. The chromophore of the Green Fluorescent Protein (GFP), which is thought to be anescetral to all other coral fluorescent proteins. These proteins are produced by the invertebrate host, not zooxanthellae. Nitrogen is an important component. From Chalfie and Kain, 2006.
Corals and anemones often form symbiotic relationships with other animals. The anemone Condylactis gigantea has a relationship with the anemone shrimp (Periclimenes yucatanicus.) Spotte (1996) showed that waste ammonium from these crabs resulted in higher zooxanthellae densities within the host anemones. Similar results could be expected in relationships between the stony coral Porites lobata and the Petroglyph Shrimp (Alpheus deuteropus), Pocillopora coral species and crabs of the genus Domecia, Trapezia crabs and members of stony coral families Acroporidae and Pocilloporidae, and so on.
Phosphorus
Phosphorus is a double-edged sword in an aquarium – it is essential for photosynthesis (and other life processes), yet too much can encourage unwanted algal growth and poison (slow) the calcification process.
In areas of the country where sources of potable water are aggressive (usually due to low water hardness), phosphorus may be intentionally added to drinking water in order to control the amounts of lead and/or copper than can be dissolved from home plumbing. Hence, it is a good idea to pre-treat such water sources through use of reverse osmosis and/or deionization devices.
Phosphorus is abundant in animal foods and wastes so phosphorus tends to accumulate in an aquarium unless measures are taken to control it.
Potassium
Potassium is usually found in seawater at a concentration of ~400 mg/l (or ppm, if you prefer.) It is difficult to imagine a potassium shortage would ever occur in a well-maintained aquarium (although I haven’t seen any long-term test results made available.)
Micronutrients
Micronutrients required by zooxanthellae are required in small amounts, although some might be in found in relatively high concentrations in seawater.
Calcium
The oxygen-evolving complex in Photosystem II depends upon a cofactor containing calcium. Calcium, as hobbyists should know, is found at a concentration of about 400 mg/l.
Sulfur
Sulfur (in the amino acid cysteine), along with iron, is important in the photophoshorylation process (where ADP becomes ATP.) Sulfur is found in seawater at a concentration of ~900 mg/l.
Magnesium
Chlorophyll c2 (C35H28N4O5Mg), an important accessory pigment in zooxanthellae is about 4% magnesium by weight. Chlorophyll a also contains magnesium. Magnesium is found in seawater at a concentration of ~1,300 mg/l.
Iron
Iron, along with sulfur (in the amino acid cysteine), is important in the photophosphorylation process (where ADP becomes ATP.)
Copper
Copper is important in maintaining the electron flow between Photosystem II and Photosystem I.
Manganese
The oxygen-evolving complex in Photosystem II depends upon a cofactor containing manganese.
Boron
Boron deficiencies in terrestrial plants have been shown to either directly interfere with chloroplast function, or indirectly repress enzyme activities (such as antioxidant enzymes.)
Chlorine (as Chloride)
Chlorine (as Cl–) helps regulate stomata actions in terrestrial plants. The oxygen-evolving complex in Photosystem II depends upon a chloride-containing cofactor. Chlorine (as chloride) should never be in shortage in a marine aquarium!
Superoxide Dismutase (SOD) and Peroxidase Enzymes &Metals
Superoxide Dismutase (SOD) enzymes are a group of specialized proteins that render superoxide radicals (O2–) inert. These radicals are produced during photosynthesis (these ‘free radicals’ also occur in the human body and are the reason we should ingest antioxidants.) These enzymes turn the superoxide radicals to ‘regular’ oxygen. Important SODs in zooxanthellae are:
- Iron SOD
- Manganese SOD
- Copper-Zinc SOD
- Nickel SOD
Oxygen radicals can also be hydrated to form O2 and hydrogen peroxide (H2O2), a strong oxidizer. Peroxidase enzymes that act upon hydrogen peroxide to ‘detoxify’ it contain iron.
Feeding with Light: Light, Photosynthesis, and Coral Nutrition
Photosynthesis is the link between inorganic and organic worlds and depends upon light energy (whether natural or artificial.) Further, the light must be of proper intensity (critical) and of proper quality (in most situations, less critical than intensity.)
Years ago, the train of thought followed the line that artificial lights could not produce enough light to achieve the levels seen in a natural environment. We now know that this is not true. Zooxanthellae have minimum and maximum lighting requirements. The minimum amount is known as the Compensation Point. At this point, zooxanthellae receive just enough light energy to produce enough oxygen and other substances (proteins, carbohydrates) to meet their requirements. In other words, they become self-sufficient. If the Compensation Point is not achieved, the symbiotic relationship stumbles and falls, and, in at least some cases, zooxanthellae might become parasitic, and could possibly harm the coral host. In other cases, insufficient light quantity (intensity) leads to the death of zooxanthellae. Fortunately, the Compensation Point for most zooxanthellae species or clades is quite low, often less than 100 µmol∙m²∙sec (or about 5,000 lux.)
When zooxanthellae photosynthesis is stimulated with enough light to surpass their Compensation Points, they produce enough beneficial compounds to share with their coral host.
So, it would seem that if a little light is good, a lot of light is better. And this is where two debates among hobbyists rages for quite some time (and are still issues for the uninformed hobbyist). These concepts are unfortunately still circulated among hobbyists: 1. It is impossible to exceed the amount of light found on natural coral reefs and 2: It would be beneficial to corals and their zooxanthellae even if we could achieve (and even exceed) that much light. The truth of these matters: It is possible to exceed the amount of light corals receive in nature and it can be harmful.
To explain this, we must examine what happens when zooxanthellae receive too much light, and we can look at a real-life example that many of us can relate to. Let’s say you have a house plant that has existed just fine in your living room for quite some time. It has thrived and grown and we could say that it is ‘happy.’ Since it is doing so well, you move it to a spot that receives a little more light with no problems – it still thrives. Its next home is on the porch and it continues to be OK. We have reached a point where the plant is receiving as much light as it can handle, and the rate of photosynthesis will not increase with increasing light intensity. This is called the Saturation Point. Its final home is in full sunlight, where it does not do well, in fact it dies after a few weeks. What has happened? Our good intentions have killed the plant because we did not understand what can happen when light intensity exceeds the Saturation Point.
Corals (or more correctly, their zooxanthellae) have similarities to our house plant example. See Figure 9 for more details.
The blue line represents the rate of photosynthesis in zooxanthellae. Numbers represent approximate light intensities in Photosynthetic Photon Flux Densities in microMol per square meter per second, denoted as µmol∙m²∙sec. At ‘0’ light intensity, no photosynthesis occurs. As light intensity increases, zooxanthellae are in a stage of caution until their Compensation Point is reached. At this point, they are self-sufficient in their product of oxygen, food, etc. It is possible that zooxanthellae could become parasitic in conditions of very low light.
The rate of photosynthesis (and production of oxygen, food, etc.) increases proportionately with light intensity until the Saturation Point is obtained (at about 500 µmol∙m²∙sec.) The upper portion of the green zone in this diagram is the ideal zone for photosynthesis. With increased light, zooxanthellae enter another cautionary zone. The rate of photosynthesis begins to drop as natural protection forces come into play (carotenoids found in the Xanthophyll Cycle begin to quench photosynthesis into order to protect the photosynthetic apparatus from being damaged from too much light. This is known as Dynamic Photoinhibition – rates of photosynthesis drop and offer a ‘pressure relief valve.’) Little or no damage to zooxanthellae occur in this zone. However, as light intensities increase, the Xanthophyll Cycle is taxed to its limit and no longer offers protection. In the red zone, rates of photosynthesis begin to fall as zooxanthellae are damaged by excessive light. This is known as Chronic Photoinhibition, and if allowed to continue could destroy zooxanthellaes’ ability to photosynthesize. In these cases, the damage is repaired daily and the zooxanthellae/coral survive but do not thrive. In extreme conditions, zooxanthellae become a hazard to corals’ health and are expelled in a process called bleaching.
Table 1 reports some important photosynthesis points for various marine invertebrates. Of particular interest, many SPS corals require little light intensity to reach their Saturation Point. A soft coral (Sinularia densa) required more light than some SPS corals. A Tridacna maxima specimen is a ‘light hog’ and Saturation could not be reached at 600 µmol∙m²∙sec (likely due to the thickness of the clam’s mantle and zooxanthellae ‘type’ or clade.)
Host | Compensation | Saturation | Photoinhibition | Depth |
---|---|---|---|---|
Acropora cervicornis | n/a | 281 | n/a | 17m |
Acropora cervicornis | n/a | 331 | n/a | 17m |
Acropora digitifera | 82 | 387 | n/a | 1m |
Acropora divaricata | 10 | 77 | n/a | 40m |
Acropora formosa | 170 | 340 | n/a | 1m |
Acropora gemmifera | 270 | 340 | n/a | 1m |
Acropora granulosa | 53 | 102 | n/a | 40m |
Acropora microphthalma | n/a | 300 | n/a | n/a |
Acropora millepora | n/a | 190 | n/a | <2m |
Acropora millepora | n/a | 230 | n/a | <2m |
Acropora nobilis | n/a | 310 | n/a | <2m |
Acropora nobilis | n/a | 180 | n/a | <2m |
Anthopleura elegantissima | 73 | n/a | n/a | n/a |
Montipora capitata | n/a | 135 | 250 | n/a |
Montipora tuberculosa | n/a | 180 | n/a | <2m |
Montipora tuberculosa | n/a | 300 | n/a | <2m |
Pavona varians | n/a | 110 | 350 | n/a |
Pocillopora damicornis | n/a | 225 | n/a | <2m |
Pocillopora eydouxi | n/a | 323 | n/a | n/a |
Porites cylindrica | n/a | 200 | n/a | n/a |
Porites lobata | n/a | 250 | 350 | n/a |
Porites lutea | n/a | 400 | 750 | 1.5m |
Sinularia densa | n/a | ~207 | n/a | n/a |
Stylophora pistillata | 40 | 200 | n/a | n/a |
Stylophora pistillata | n/a | 300 | 600 | n/a |
Tridacna maxima | n/a | >600 | >1,900 | n/a |
Water Motion
Any article on water motion in hobbyist literature discusses its importance in food delivery, removal of wastes and sediments, and so on. We’ll briefly discuss an important concept in the water motion dependent delivery of dissolved macro- and micronutrients to marine invertebrates and hence their zooxanthellae.
The Momentum Boundary Layer
The Momentum Boundary Layer (MBL) is a layer of relatively stagnant water that surrounds all benthic objects. Its thickness is inversely proportional to water velocity that is, its thickness decreases as water velocity increases and vice versa. The water velocity within the MBL is, by definition, 0 to 99% of that in the water column. In natural situations, the thickness of the MBL is usually on the scale of millimeters.
A diffusion gradient within the MBL may occur when an organism extracts a substance from the water column. A deficiency occurs when the substance cannot diffuse quickly enough through the MBL. Hence, diffusion rates depend upon the substance’s concentration in the water column and the thickness of the MBL – a substance in high concentration (such as magnesium at ~1,300 mg/l) should diffuse in a satisfactorily manner through a thick boundary layer, while a substance in a low concentration (such as many micronutrients) require ‘good’ water motion which results in a minimal MBL.
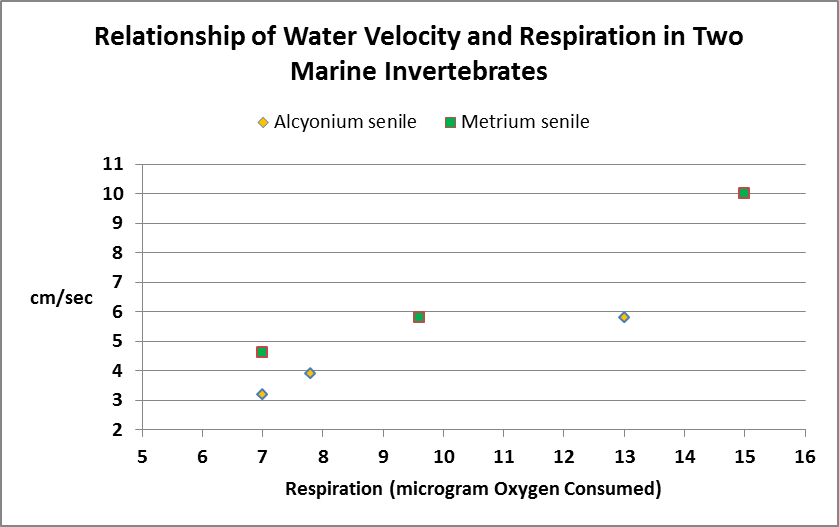
Figure 10. Respiration in these two marine invertebrates increases more or less in proportion to water velocity.
Researchers have determined that water motion affects marine invertebrate respiration.
Respiration has a number of definitions:
- Cellular respiration: Nutrients are converted to energy within a cell.
- Maintenance respiration: Amount of cellular respiration required for an organism to maintain itself in a constant state.
- Aquatic respiration: An aquatic organism extracts oxygen from the water.
In any case, Figure 10 shows the effects of water motion on aquatic invertebrate respiration.
In Closing
Coral animals are composed of proteins, lipids, carbohydrates, and inorganic ‘ash.’ A symbiotic relationship exists between the coral animal (the host) and zooxanthellae, and when conditions are proper (lighting, water motion, fertilization), important nutriment (proteins, carbohydrates, lipids) are translocated to the coral.
Water motion and lighting are critical elements in promoting photosynthesis in captive zooxanthellae.
Next time, we’ll begin a detailed investigation into the amino acid synthesis by zooxanthellae and the invertebrate host.
Comments? Questions? I’m best reached at: [email protected].
References
- Atkinson, M. and C. Bingman, 1997. Elemental composition of commercial seasalts. J. Aquaculture and Aquatic Sciences, VIII (2):39-43.
- Chalfie, M. and S. Kain, 2006. Green Fluorescent Protein. Properties, Application, and Protocols. John Wiley and Sons, Hoboken, New Jersey. 443 pp.
- Chailow, K., 1971. Ecological metabolism in the sea. Naukova dumka, Kiev.
- Jones, D., 1931. Factors for converting percentages of nitrogen in foods and feeds to percentages of protein. U.S. Dept. of Agriculture, Circular 183 (Revised February, 1941.)
- Patterson, M. and K. Sebens, 1989. Forced convection modulates gas exchange in cnidarians. Proc. Natl. Acad. Sci., 86: 8833-8836.
- Patterson, M. and K. Sebens, 1991. In-situ measurements of flow effects on primary production and dark respiration in coral reefs. Limnol. Oceanogr., 36(5): 936-948.
- Pearse, V. and L. Muscatine, 1972. Role of symbiotic algae (zooxanthellae) in coral reef calcification. Biol. Bull., 141:350-363.
- Sorokin, Y., 1973. On the feeding of some scleractinian corals with bacteria and dissolved organic matter. Limnol. Oceanogr., 18:380-385.
- Sorokin, Y., 1981. Aspects of the biomass, feeding and metabolism of common corals of the Great Barrier Reef, Australia. Proc. 4th Int. Coral Reef Symp., Manila. 2:27-31.
- Spotte, S., 1996. Supply of regenerated nitrogen to sea anemones by their symbiotic shrimp. J. Exp. Mar. Biol. Ecol., 198(1):27-36.
Always a pleasure to read Dana Riddles articles.
Excellent and invaluable information, a must read for every aquarist serious about keeping corals alive and healthy.