For so many aquarists, one of the most satisfying activities is observing trophic interactions amongst the animals in their keep-that is, watching them eat. Some of these interactions are quite conspicuous (feeding live foods to carnivorous fishes or adding hermit crabs to eradicate nuisance algae, for example). Others, while considerably important to the ecological fitness of the aquarium system, are not so obvious. These processes are for the most part carried out by microscopic organisms and are thus typically unrecognized by the keeper’s naked eye. As such, microbial activity (or a lack thereof) may only be evident indirectly by odors or discoloration of aquarium water, biofouling of the rock and tank panels or (ideally) the analysis of water chemistry using precision testing equipment.
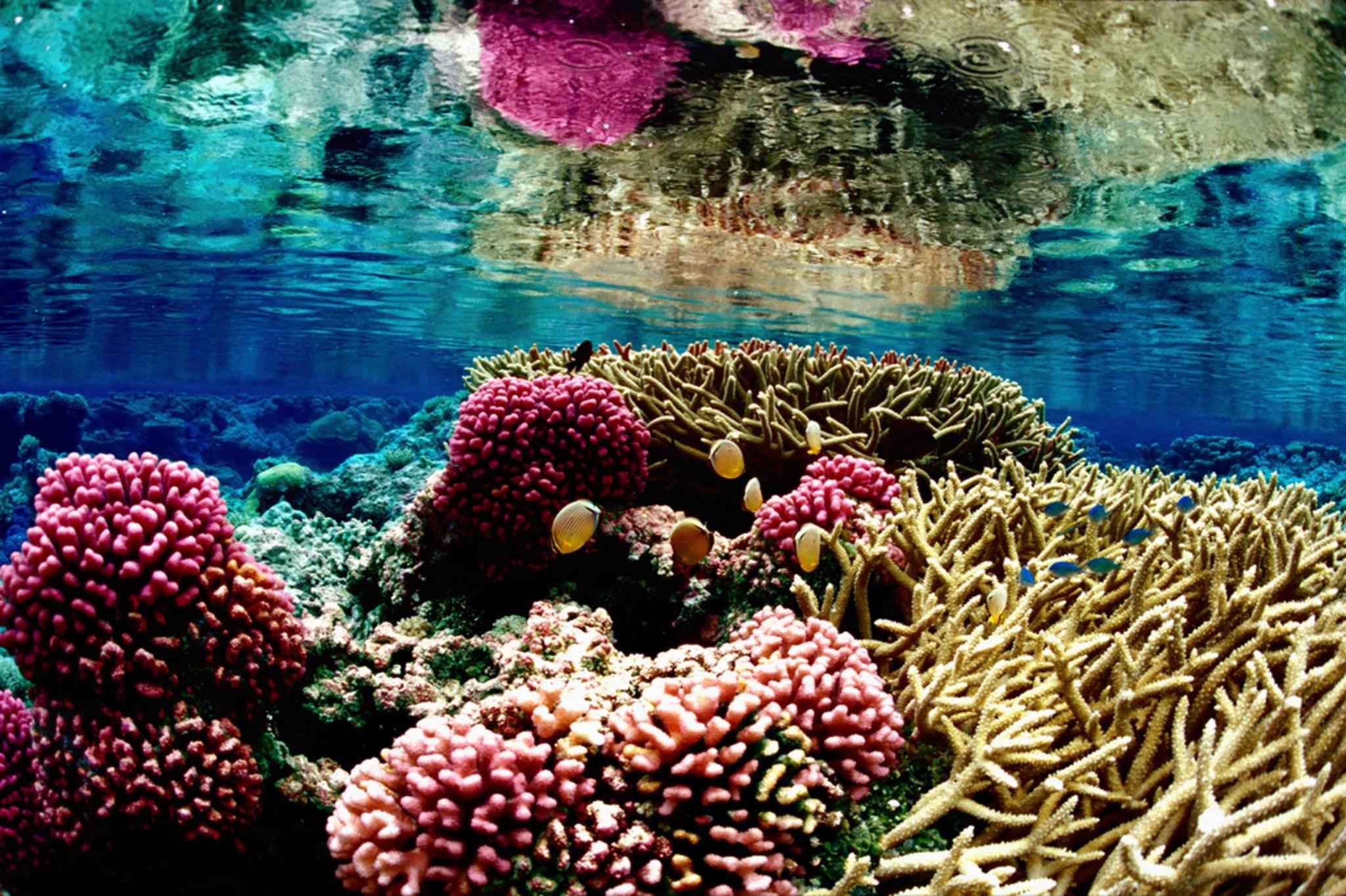
In the nutrient-poor environment of a typical coral reef, it is zooxanthellae rather than phytoplankton that are responsible for most primary productivity. Photo by Jim E Maragos, U.S. Fish and Wildlife Service.
A good majority of marine aquarium keepers (advanced or otherwise) are well aware of some of the autotrophic processes that take place in their tanks. Autotrophy can be best described as the utilization of environmental energy (as from sunlight or through the oxidation of inorganic substances) to synthesize organic molecules from inorganic molecules. In short, autotrophs (also called primary producers) create biomass from nonbiological sources of energy and material. This diverse group includes organisms such as the photosynthetic dinoflagellates that live endosymbiotically within hermatypic corals (i.e. zooxanthellae) as well as the chemosynthetic bacteria (e.g. Nitrosomonas and Nitrobacter) that populate so-called biological filter media.
To be sure, this keen attention to autotrophy is duly warranted, as it is as critical to the health of our reef tanks as it is essential for all life on Earth. Even so, autotrophs form but one of two major components in the complex marine food web that aquarists strive so diligently to replicate. The other, complimentary part of the equation is claimed by the heterotrophs.
Heterotrophy can be described as the acquisition of material for growth and energy through the consumption of other organisms and/or their organic bioproducts. That is, heterotrophs (also called secondary producers or consumers) must eat to survive. This group is perhaps just about as diverse than the autotrophs. It includes relatively large organisms like you, me and the fishes. But it also includes a great variety of microscopic organisms. This is where the term “eat” becomes rather broad; these tiniest and simplest of lifeforms must obtain their sustenance either through phagocytosis (the envelopment of solid particles by a cell body) or through the absorption of dissolved organic substances from the surrounding waters. In so doing, they “finish off” organic matter that has been leached, excreted or partially broken down via degradation and decomposition.
Carbon recycling in reef environments
Coral reefs are discrete ecosystems with their own biological communities. These communities do, of course, include both producers and consumers. However, coral reef environments are somewhat remarkable in their unusually high degree ecological efficiency. Indeed, they rival tropical rain forests in terms of productivity. While extremely productive, coral reefs are necessarily situated in shallow, clear, nutrient-poor waters. This seeming paradox is a consequence of very tight nutrient cycling, which is itself a consequence of the close association between reef-building corals and their symbionts. But corals-even those “photosynthetic” varieties-do eat, and therefore require the continuous input of foods from the water column. While some of this food consists of particulate organic matter (POM) such as marine snow, the bulk of it generally consists of plankton.
Though they might seem relatively empty and devoid of life, the open water environments above and adjacent to coral reefs can be regarded as marine pastures. Examined under a microscope, even the clearest open waters are teeming with life. These miniscule, free-living organisms, the plankton, are in many cases a significant food source for nearshore habitats (such as coral reefs). The washout of nutrients and dissolved organic carbon (DOC) from these coastal environments, which owes to exchanges fueled primarily by tidal action, is conversely a notable source of nourishment for the planktonic organisms. Still, the input of organic carbon obtained from drifting oceanic phytoplankton accounts for little of a reef’s gross production-something like 0.3%-13%. In actual fact, most of the plankton that corals consume originate on the reef itself.
As are nutrients such as nitrate and phosphate, DOC is primarily recycled within the confines of the reef proper, and so is its heterotrophic plankton. These planktonic organisms (e.g. bacteria, archaea, protozoa and miniscule animals such as copepods) consequently play a major part in reef productivity and carbon cycling. They form vital connections within the reef food web through the recycling and remineralization of organic matter at nearly all trophic levels. The fundamental implication of this cycle is that organic carbon moves to higher trophic levels through uptake by organisms at lower trophic levels. More specifically, bacteria and some phytoplankton (e.g. facultative heterotrophs such as certain diatoms and dinoflagellates) consume dissolved organic carbon. These simple organisms are consumed by protozoa. Larger plankters such as copepods consume phytoplankton and protozoa and then are in turn preyed on much larger benthos such as corals. The excretions, secretions and carcasses of these benthic organisms ultimately return organic matter to the pelagic food web.
High loads of heterotrophic bacteria and protozoans mineralize much of the DOC and particulate organic carbon (POC) into (1) nutrients such as ammonium and (2) dissolved inorganic carbon (DIOC) such as carbon dioxide, both of which promote the growth of phytoplankton and benthic algae. Significantly, a large proportion of DOC and POC is redirected to the lowest trophic levels. This is largely due to infection of bacteria by viruses; infection leads to cell rupture, resulting in the spilling of DOC and POC, which is again taken up by bacteria in an endless cycle referred to as the microbial loop. As much as 60% of the energy in a marine ecosystem can be invested in this pathway.
Up the trophic ladder
Mortality of bacterioplankton from protozoan predation is roughly equivalent to that from viral infection. However, the ecological effect of protozoan grazing is markedly different. Rather than being relayed into the microbial loop, bacterial biomass is passed on to higher trophic levels. This is especially important in nutrient-poor environments, where major metazoan plankters (e.g. copepods) seem to preferentially prey on protozoa (e.g. ciliates). Benthic, filter-feeding reef animals (e.g. sponges) might prey directly on bacterioplankton. Other filter-feeders (e.g. sea pens) can obtain nutrition from bacteria in a more indirect manner, as they can capture and consume bacteria that attach to sinking POM. Detritivores similarly benefit from this source as it contributes to the organic sediment pool. Even herbivores can utilize this food source; as much as 30% of the sinking POM that deposits and enriches algae surfaces (thereby improving its palatability and nutritional value) is made up of microbial biomass.
Being as the waters above a coral reef are so unproductive, the chief supply of organic carbon on a coral reef (at least that which is produced locally) is from benthic primary producers. Major producers in these environments are zooxanthellae and, to a lesser extent, fleshy and crustose algae. Primary production budgets on the reef surface are very much regulated by intricate interactions between these autotrophs and certain heterotrophic microbes.
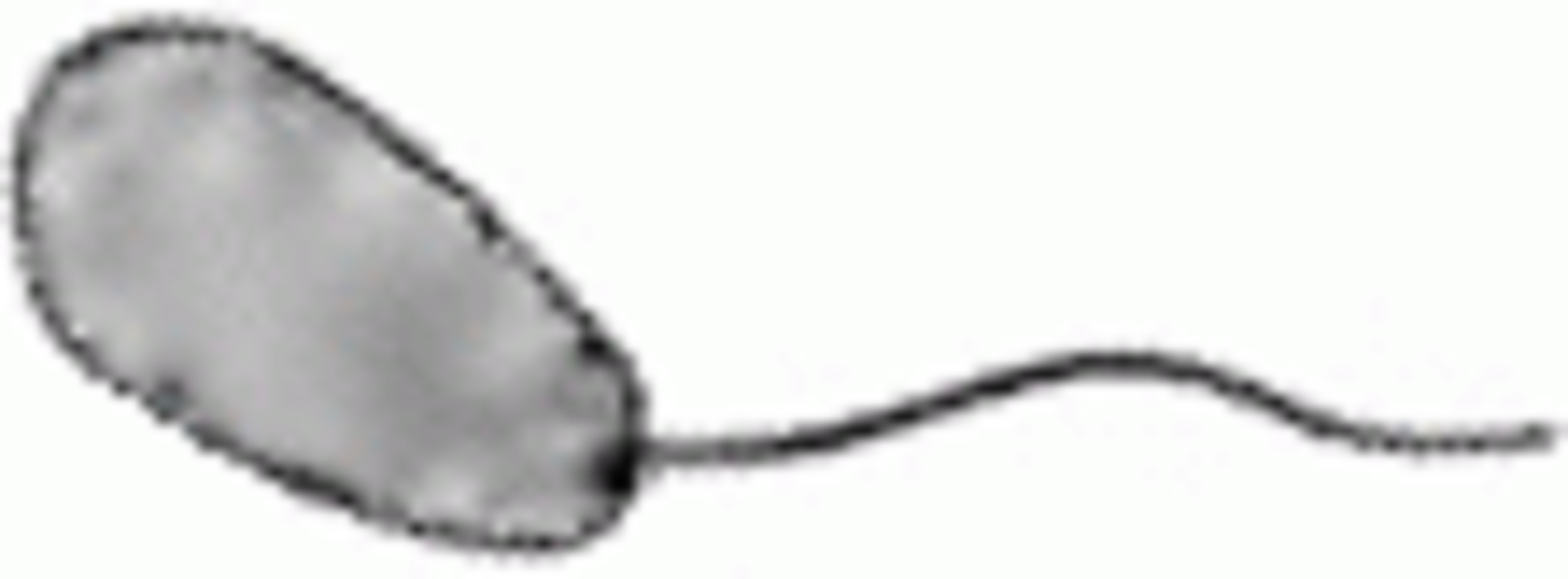
Some motile microbes are chemotactic, capable of detecting and swimming toward rich sources of DOM. Photo by Dennis Myts.
Around 40-80% of a hermatypic coral’s nourishment is derived from organic matter supplied by its photosynthetic symbiont. Around 10-50% of this carbon is released through the discharge of mucus. Some of the POM that is released into the open waters serves as a water-born particle trap delivering food and energy to filter feeders and detritivores (tube worms, nematodes, etc.). The easily-broken down DOM is either incorporated into particulates that may be exploited by both benthic and pelagic consumers or simply enters the microbial loop. Thus, coral exudates strongly promote the growth of heterotrophic bacteria that dwell in the water column. Reef-associated bacteria consequently proliferate at a rate that is approximately 50 times higher than that of those inhabiting the open ocean.
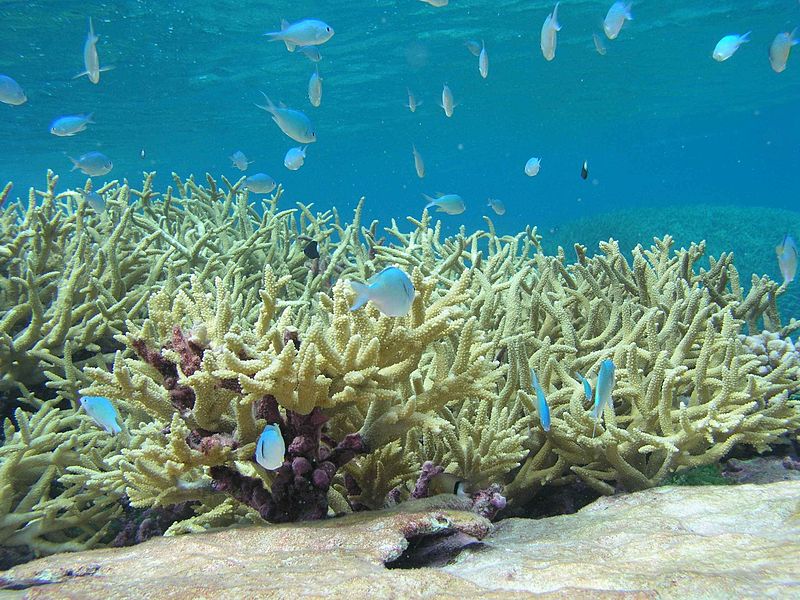
Organic carbon moves to the uppermost levels of the trophic ladder as planktivorous fishes (such as these Chromis sp.) are preyed on by larger piscivores. Photo by Amanda Meyer, U.S. Fish and Wildlife Service.
In stark contrast to oceanic bacteria, the growth rates of reef bacteria are not strongly linked to phytoplankton productivity. This suggests that reef-associated heterotrophic bacteria are not highly dependent upon phytoplanktonic sources of carbon; they rather rely principally upon organic materials secreted by benthic organisms. Not only chemical makeup, but also microbial activity, regulate the specific allocation of this recycled dissolved carbon. So, heterotrophic bacteria have a considerably stronger influence on the trophic structure of a reef than that of open-ocean habitats (which is governed primarily by phytoplankton productivity).
Amazingly, bacteria make up 37-73% of the total biomass in the heterotrophic plankton over coral reefs, where they commonly occur in densities of a million cells per milliliter. In one important respect, reef bacteria serve as compensation for the relative sparseness of reef phytoplankton (and, by extension, phytoplanktivorous zooplankton). In marine food webs, over 95% of organic matter is composed of large, complex molecules such as proteins and lipids. Therefore, little DOM is available to higher organisms through direct uptake. Moreover, while these compounds are rich in carbon, they are typically lacking in nitrogen and phosphorus. Due to the release of exudates by benthic organisms, DOM production is high in reef environments. After enriching it through the parallel uptake of phosphorous and nitrogen, reef bacteria redirect this organic carbon source back into the food web where it may be passed on and on to higher trophic levels.
Implications for coral-microbe relations in captive systems
The density of organisms that reside at higher trophic levels-especially fishes-tends to be considerably higher in our reef aquaria than in natural reef environments. Frankly, reef aquarists love reef fish. And, to return to an important point, they love to feed them. As a matter of fact, they often overfeed them. This commonly leads to problems associated with excessive nitrate and phosphate concentrations. In other words, what should be an oligotrophic (i.e. nutrient-poor) system effectively becomes a eutrophic (i.e. nutrient-rich) system. This results (among other decidedly bad things) in the rapid proliferation of undesirable benthic algae. From what we observe directly, these algal films and turfs seem to simply outgrow and smother corals. But there is considerably more to it than that.
Heterotrophic bacteria feed on DOM from algae as well as that from corals. Releases from algae are different from those from corals. Firstly, algae release DOM at a rate that is as much as seven times higher than that of corals. Additionally, algal exudates differ from coral exudates in gross composition. Most significantly, DOM released from algae is rich in carbohydrates whereas DOM from corals is rich in proteins and lipids. These differences in food composition do not appreciably alter the microbial community; they do, however, alter the metabolic pathways utilized in microbial food consumption. It could be said that algal DOM is bacterial junk food.
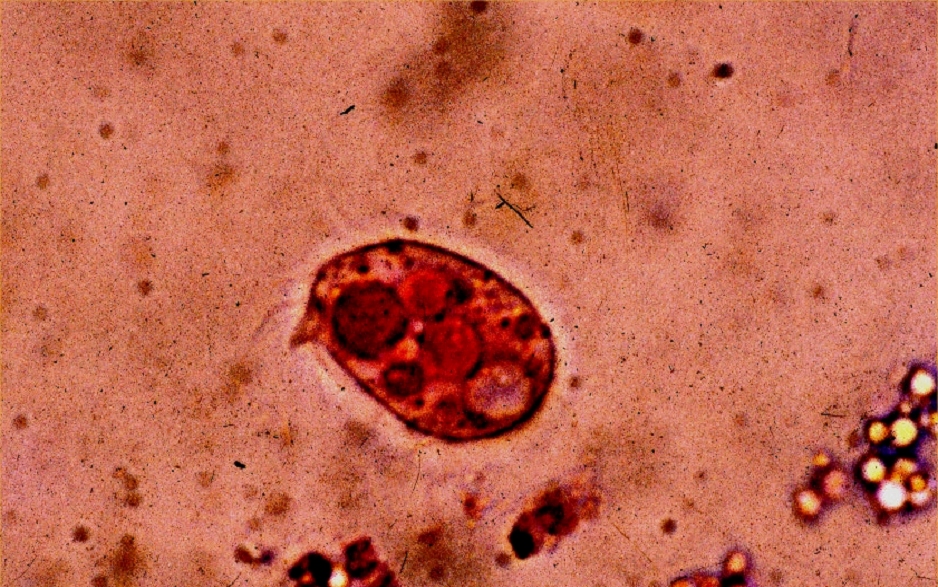
Where bacterial densities are high, some ciliates can consume as many as 50,000 cells in a single hour. Photo by Dr. Eugen Lehle.
Particularly in environments where sources of nutrition are scarce, many flagellated heterotrophic bacteria are capable of sensing and swimming toward food. They may congregate, rapidly proliferate and form extremely high densities over algal patches. Although these microbes do so much good for corals and coral reefs, too many of them can be a bad thing. Localized microbial feeding frenzies ensue where DOM is released from benthic algae. The resulting increase of bacterial respiration creates hypoxic zones just over the patch surface. The algae appreciate the extra carbon dioxide, whilst any nearby corals suffer for a lack of oxygen. As coral tissue dies and recedes, algae expand into the unclaimed territory.
Sometimes, in an effort to thwart nuisance algae growth, aquarists employ carbon dosing to encourage the growth of heterotrophic bacteria. This is done in the hope that the resulting bloom of bacterioplankton will scavenge excess nutrients and thereby starve out the algae. However, the resulting explosion of bacterial growth may greatly reduce dissolved oxygen concentrations and further stress the corals (as well as other animals). While carbon dosing is reported by some to have substantial benefits, overdosing or misuse can have serious negative consequences. Carbon dosing should therefore be used only as a means of modest, ongoing nutrient control. In cases of sharp nitrate or phosphate spikes, the best means of control are old-fashioned water changes. Where there are chronically high nutrient concentrations, a reduction of feeding and/or stocking might be in order.
What the aquarium keeper should be striving to establish is an ecologically appropriate balance. Use of ozone or ultraviolet sterilization can cause an overall decline in their density. Diversity of heterotrophic bacterioplankton can be lost through competitive exclusion. On the other hand, overfeeding can cause blooms thick enough to impart a discernable milky haze in the water. Other than through more subjective means of criteria (i.e. overall well-being and cleanliness of the system), aquarists can roughly estimate the density of their heterotrophic bacteria population using biological activity reaction tests such as the Heterotrophic Aerobic Bacteria (HAB) test made by Droycon Bioconcepts of Canada.
Given their importance on natural reefs, heterotrophic bacteria likely play a major role in the health and stability of reef aquaria. Yet, aquarists in general have only begun to recognize their value. The prevalence of bacterial food supplements and live “probiotic” and “digester” bacterial cultures in the aquarium industry certainly hints at a greater interest in this area. However, the greatest advances in captive microbial management will likely come (as so much advancement does) from thoughtful experimentation and communication between dedicated aquarium hobbyists.
References
- Silveira, Cynthia B., Giselle S. Cavalcanti, Juline M. Walter, Arthur W. Silva-Lima, Elizabeth A. Dinsdale, David G. Bourne, Cristiane C. Thompson and Fabiano L. Thompson. “Microbial processes driving coral reef organic carbon flow.” (PDF Download Available). Available from: https://www.researchgate.net/publication/316843950_Microbial_processes_driving_coral_reef_organic_carbon_flow [accessed Aug 30, 2017].
- Feldman, Ken S., Allison A. Place, Sanjay Joshi and Gary White. “Bacterial Counts in Reef Aquarium Water: Baseline Values and Modulation by Carbon Dosing, Protein Skimming, and Granular Activated Carbon Filtration.” Advanced Aquarist Online Magazine Volume X March, 2011.
- Levinton, Jeffrey S. Marine Biology, 2nd ed. New York, NY: Oxford University Press, 2001.
- Azam, Farooq, Tom Fenchel, JG Field, JS Gray, LA Meyer-Reil and F Thingstad. “The Ecological Role of Water-Column Microbes in the Sea“. Marine Ecology Progress Series. 10: 257-263. 1983.
0 Comments