Read part one: “Improved husbandry of marine invertebrates using an innovative filtration technology – Part 1: DyMiCo ” by Tim Wijgerde, M.Sc.
As the marine ecosystems of our planet slowly dwindle, the demand for marine life from the aquarium industry puts evermore pressure on wild populations. With climate change wreaking havoc on coral reefs, and ocean acidification looming on the horizon, the importance of sustainable aquaculture is increasing rapidly. Fortunately, there are growing efforts to culture a myriad of marine and freshwater species. Some species, however, are lagging behind despite high demand from the aquarium industry. As detailed in part one, so-called filter and suspension feeding organisms that rely heavily on live plankton do not fare well in closed systems. These include many species of sponges, corals and echinoderms, which are interesting candidates for sustainable aquaculture. Filtration systems that are designed to maintain plankton populations and high water quality simultaneously may be an important step to increase the number of species that can be aquacultured.
In part one I introduced DyMiCo (Dynamic Mineral Control) as an alternative to systems using foam fractionators or common biofilters, to allow the buildup of a plankton population. Several institutions have been using DyMiCo over the last decade, including Rotterdam Zoo and Wageningen University (The Netherlands), Antwerp Zoo (Belgium), and NAUSICAA (France). The systems at these institutions have been running for up to eight years straight, with flourishing marine life. In early 2011, two DyMiCo systems were set up as part of an experimental coral nursery project in Utrecht, The Netherlands (see www.ecocoral.eu). These systems each have a volume of 12 m3 or 3,158 USG and hold many invertebrates including approximately 3,000 scleractinian corals in total. One of these systems was stocked with several invertebrates whose husbandry has proven highly difficult, in order to assess their response to DyMiCo. These included sponges (Trikentrion flabelliforme), gorgonian octocorals (e.g. Guaiagorgia sp.), hydrozoans (Distichopora spp.) and Comatulid crinoids. Below I will give an overview of the observations on both systems, in terms of water quality, plankton abundance and the welfare of the aforementioned invertebrates.
Water quality
Various water parameters were measured once a week. Inorganic nutrients, i.e. ammonium, nitrate and phosphate, were measured with a spectrophotometer. ICP-AES and ICP-MS, short for inductively coupled plasma atomic emission spectroscopy/mass spectrometry, were used to determine a wide range of chemical elements in the water. Most water parameters were quite stable throughout the year. This was most likely the result of low stocking densities.

Inorganic nutrients were measured with a spectrophotometer (left). Alkalinity was measured with an automated titrator (right).
Ammonium, nitrate and phosphate were low throughout the year, even though no water changes were performed. Calcium, magnesium and alkalinity were very high due to the DyMiCo reactor, which as described in part one functions as a calcium reactor. Several trace elements (i.e. metals) were present in concentrations which exceeded those of seawater (Spotte 1992), which was likely due to the chemical composition of the artificial salt used. These included potassium, barium, cadmium, cobalt, chromium, copper, iron, lanthanum, lead, antimony and tin. Although the aquarium industry heavily promotes the dosage of various trace elements to home aquaria, it seems that without the proper analytical methods, the home aquarist may overdose a wide range of potentially toxic elements. Strontium, boron, aluminium, manganese, arsenic, lithium, molybdenum, nickel and selenium were lower in concentration when compared to seawater, and some of these elements were below the detection limit of the mass spectrometer. Despite the imbalances measured, life in the systems flourished. This may have to do with the thriving plankton population, which is known to contain high amounts of trace elements next to organic carbon, nitrogen and phosphorus, as bacteria, algae and crustaceans accumulate elements from the seawater (Martin and Knauer 1973). This may also explain the concentration decrease of certain elements. It is promising to observe that without water changes, many water parameters such as calcium, magnesium, alkalinity, nitrate and phosphate remain stable around levels considered acceptable to marine organisms.
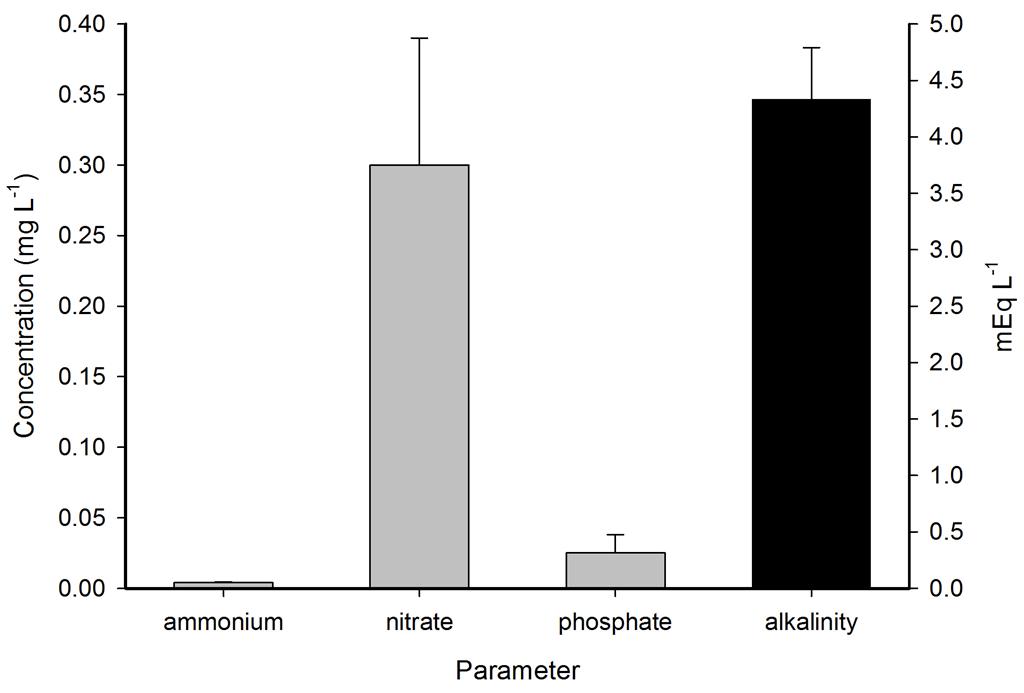
Representative measurements of ammonium, nitrate and phosphate in mg L-1 and alkalinity in mEq L-1 in the DyMiCo systems. Error bars show standard deviations (N=2).
The pH in both systems also remained fairly stable at 8.1±0.1, even though no foam fractionator or air stones were used. This was most likely the result of low stocking densities, a large water surface area and adequate surface flow, the latter two promoting gas exchange. Temperature was maintained around 26 degrees Celsius (79 degrees Fahrenheit) by heating or ventilating the room, depending on environmental conditions. Salinity was highly stable at 35 g L-1, resulting from an automated top-off system connected to a RO/DI unit.
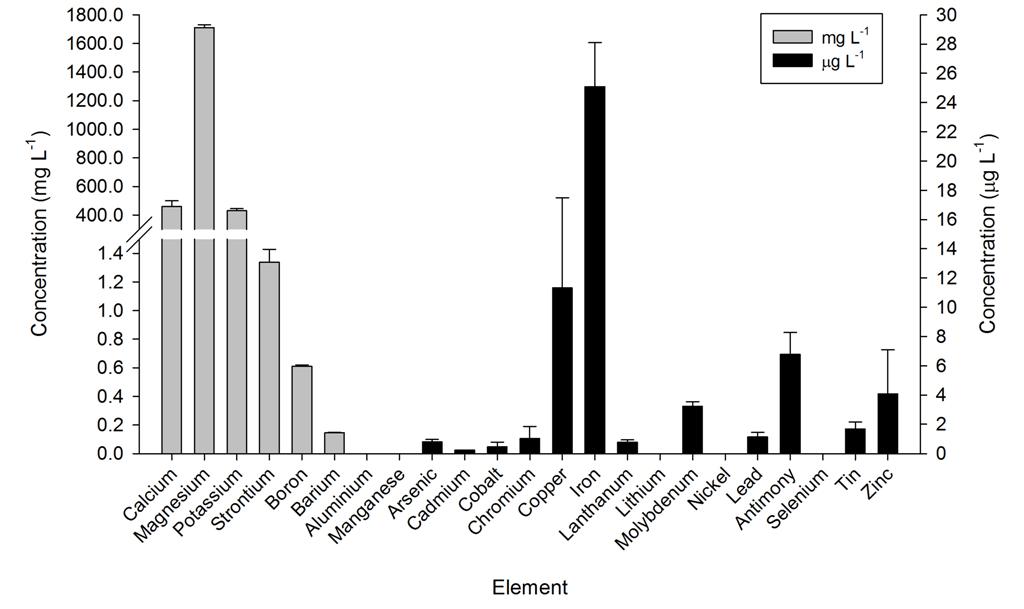
Overview of several elements measured in the DyMiCo systems, in mg L-1 (grey bars) and g L-1 (black bars). Error bars show standard deviations (N=2).
Plankton abundance
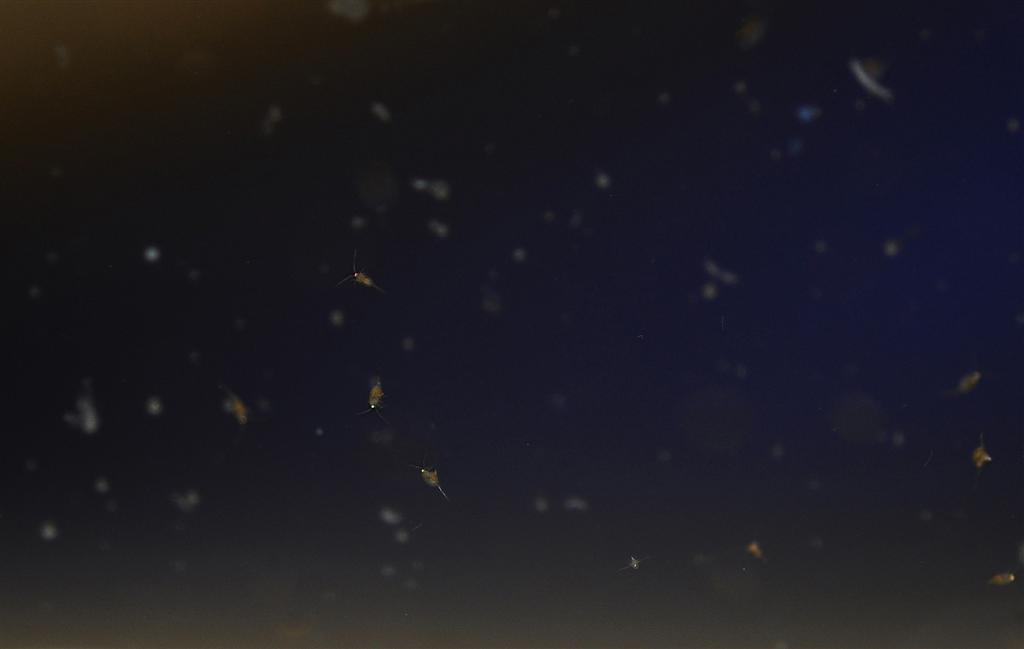
Copepods, here swarming in a DyMiCo system, form the bulk of natural zooplankton. At night, these zooplankters migrate to the water column and serve as a food source for marine invertebrates.
To spur the initial buildup of a plankton population, several commercial products were dosed three times per week. Although DyMiCo produces its own plankton, which is seeded by the introduction of live rock and corals, it is important to aid the system when grazing pressure is high. In other words, as the ratio between live animals and system water is increased to an unnaturally high level, the demand for food particles invariably follows suit. Products that were used included Phyto Feast live, containing five different types of live phytoplankton, Oyster Feast, a homogenate of oyster ovarian tissue and eggs, live adult Tigriopus californicus copepods and various frozen and dried feeds (Table 1).
After several months, copepods and their nauplii swarmed throughout the system, especially at night. Small unidentified shrimps were also present. During several weeks, one system was dominated by ephyra larvae of an identified species, possibly a hydrozoan. This vibrant population of zooplankton indicated that phyto- and or bacterioplankton were available in sufficient quantities, as copepods and other crustaceans grew and reproduced.
Feed | Type | Amount (ml or g) | Frequency (feedings system-1 week-1) | Manufacturer |
---|---|---|---|---|
* feedings system-1 year-1 | ||||
Phyto Feast Live | live | 100 ml | 3 | Reed Mariculture, USA |
Oyster Feast | refrigerated | 100 ml | 3 | Reed Mariculture, USA |
Tigriopus californicus | live | 500 ml | 3* | Reed Mariculture, USA |
Rotifers | frozen | 21 g | 3 | Ruto B.V., The Netherlands |
Copepods | frozen | 21 g | 3 | Ruto B.V., The Netherlands |
Porphyra sp. | dried | 1.4 g | 2 | Granfood B.V., The Netherlands |
The invertebrates
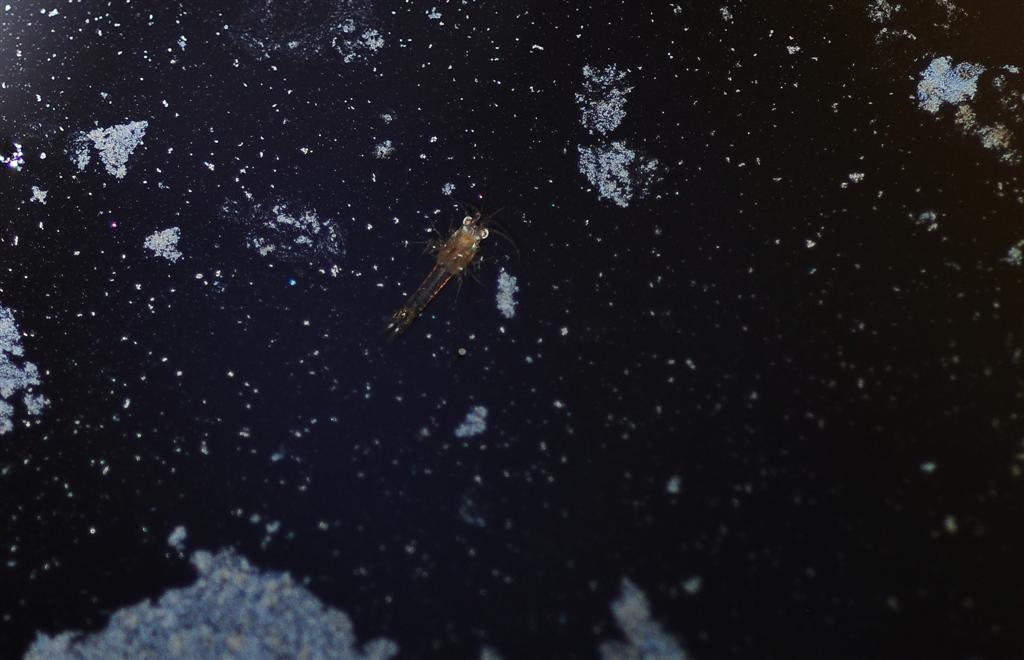
One of the many small, unidentified shrimp roaming in the dark corners of the system. Such macrozooplankton (2-20 mm) constitutes an excellent food source for many species including fishes.
High water quality and a vibrant plankton population resulted in thriving invertebrates. The species discussed below usually wither and die in the gin-clear waters of heavily filtered systems. Although these animals have only been growing in this system for a year, the results have been tantalizing.
Sponges
Although different species of sponges have been growing in the system, there is one that is truly fascinating. This sponge is referred to by the scientific name Trikentrion flabelliforme, and was introduced deliberately. T. flabelliforme is a sponge that is becoming increasingly popular in the international trade. Known under the dubious trivial name Spider Sponge, this species is fascinating in the sense that it has formed a symbiosis with a hexacoral from the genus Parazoanthus (order Zoanthidea). T. flabelliforme belongs to the largest class within the phylum Porifera known as the Demospongiae, and is found in the Arafura Sea, a shallow body of water sandwiched between Australia and New Guinea. It is a typical filter feeder as it uses its choanocytes or collar cells to filter particles and dissolved substances from the seawater. Its coral symbiont is very likely Parazoanthus swiftii, as this species is the only coral known to associate with sponges from the order Poecilosclerida, to which T. flabelliforme belongs (Swain and Wulff 2007). P. swiftii is a suspension feeder as its polyps capture food particles from the water. Individual polyps are connected by common tissue known as coenenchyme or coenosarc, in the form of horizontal bridges or stolons.
The tissue of Parazoanthus is connected to the skin or pinacoderm of its host sponge, with tissue integration varying between different combinations of sponge and coral species. The physical integration between T. flabelliforme and P. swiftii seems to be superficial, in contrast to Epizoanthus spp. which may penetrate deeper into sponge tissue. The latter morphology, according to researchers, suggests a mutualistic symbiosis from which both partners benefit. The exact nature of the symbiosis between T. flabelliforme and P. swiftii is not yet clear. Parasitism seems a likely option, where the symbiotic coral benefits at the expense of its host sponge. For example, the coral may impair the sponge’s ability to pump water through its system, which is vital to sponge nutrition, waste removal and gas exchange. Commensalism is also possible, where the coral benefits while having a neutral effect on the sponge.
Although many hobbyists have tried to maintain this sponge, its survival record in closed aquarium systems has been poor. This is most likely the result of malnutrition, as this sponge and its symbiont heavily rely on food particles and dissolved organic carbon. Sponges are known to filter the smallest food particles from the water, including viruses, bacteria and phytoplankton, which are all below 10 microns (µm) in diameter. For reference: a single Artemia nauplius, which already seems small to the naked eye, measures roughly 440 µm in length. Another major part of the sponge diet is composed of dissolved organic carbon or DOC (De Goeij et al. 2009). Picoplankton (0.2-2 µm) and nanoplankton (2-20 µm) may not be available in closed systems in sufficient quantities, possibly the result of foam fractionating and overstocking of invertebrates. The coral symbiont requires zooplankton or detritus in sufficient quantities, and may feed on rotifers (e.g. Brachionus plicatilis), copepods (e.g. Tigriopus sp.), Brine shrimp (Artemia salina) and their nauplii.
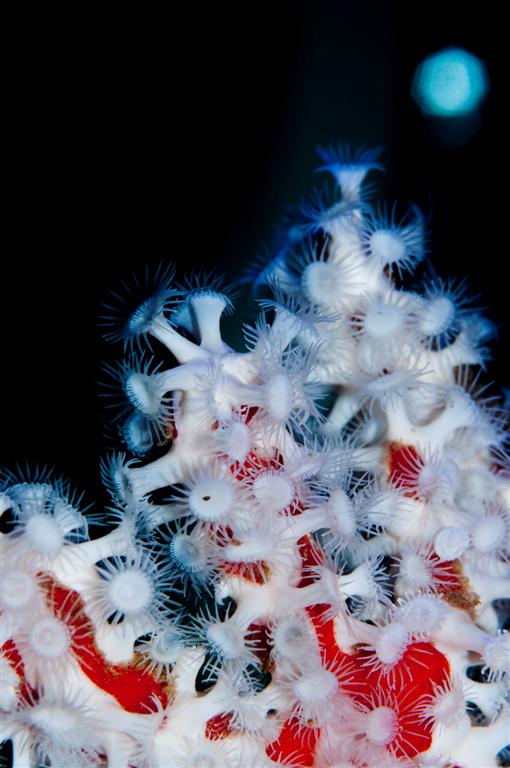
This sponge (Trikentrion flabelliforme) hosting a symbiotic coral (Parazoanthus sp.) seems to thrive under strong water flow and high prey availability.
T. flabelliforme has been growing in the system for a year now, and shows signs of health. Its symbiotic Parazoanthus polyps are often well expanded, especially after a batch of plankton is introduced. Although I have not been able to accurately measure growth, by e.g. determining specific growth rate, the two colonies I have observed seemed to gain volume.
Due to reorganization of the system, I have kept this sponge under several distinct microenvironments; strong (> 10 cm s-1) or weak (< 5 cm s-1) water flow rates, with moderate or low light intensity. It seems to prefer stronger water currents, although I cannot substantiate this. The presence of light, at least moderate irradiances (< 100 µm m-2 s-1), do not seem to harm the sponge or its symbiont. However, fouling organisms such as algae and cyanobacteria may take hold over the colony when it is weakened or damaged. Therefore, shading this sponge from direct light may be favorable.
Hydrocorals
Next to sponges, hydrocorals have been growing in the system over the last year. These invertebrates are not too popular in the ornamental trade, which may have to do with their difficult husbandry. Hydrocorals are not true corals, which is why they do not belong to the Anthozoa class. They are placed in a completely different class within the phylum Cnidaria, namely the Hydrozoa. There are two families within the Hydrozoa that contain coral-like species; the Milleporidae or Fire corals, and the Stylasteridae or Lace corals. Hydrocorals are often mistaken for scleractinian corals because they have a hard, calcareous skeleton, but they are only similar in appearance. Unlike scleractinian corals, they have differentiated polyps with different biological functions. This phenomenon is also found amongst many octocorals. The gastrozooids or feeding polyps are dedicated to prey capture and nutrient acquisition, and the dactylozooids or defensive polyps use their powerful cnidocytes to defend the colony. The way these different polyps are organized spatially in a colony depends on species. A common arrangement is one gastrozooid that is surrounded by five to fifteen dactylozooids, the latter being much longer and thinner. Lace corals are shades of purple, pink or red and much more colorful than fire corals, which are typically yellow-colored.
The difficulty surrounding the husbandry of these corals is, similar to the other animals discussed here, most likely stems from their feeding habits. Although members of the Milleporidae host symbiotic dinoflagellates to support their metabolism, Stylasterids such as Distichopora spp. do not. This causes them to quickly deteriorate in the average aquarium, their bare corallum quickly changing into a shade of green or brown due to algal fouling. I have had some fortunate experiences with several purple Distichopora specimens, of which I have not been able to determine their exact species. These specimens were introduced in good condition, and have remained healthy ever since their introduction in the system about a year ago. Their whitish, calcifying tips and continuous polyp expansion are indicators of health. Dactylozooids can be seen macroscopically. I also introduced a larger, blue specimen that was already deteriorating. Unfortunately, this colony did not recover and slowly died over the course of several months.
Gorgonian octocorals
Gorgonians (subclass Octocorallia, order Alcyonacea) have become popular ornamentals over the last few years, with the advent of specialized aquarium displays. Their interesting morphologies and colors attract the attention of many aquarists and hobbyists. At this moment, gorgonians are classified into three suborders within the Alcyonacea order; the Calcaxonia, Scleraxonia, and Holaxonia. Although they are related to soft corals, and to a lesser degree scleractinian corals, their body plan is quite different. The inner core of their skeleton houses a proteinaceous rod composed of gorgonin and collagen fibers that provide the colony with stability (Fabricius and Alderslade 2001). Members of the Calcaxonia have rods that are reinforced with aragonite, a form of calcium carbonate or limestone that makes up the skeleton of scleractinian corals. The Scleraxonia suborder contains species that produce sclerites-tiny needles made from calcite, another form of calcium carbonate-that provide strength. The Holaxonia are characterized by a hollow inner core.
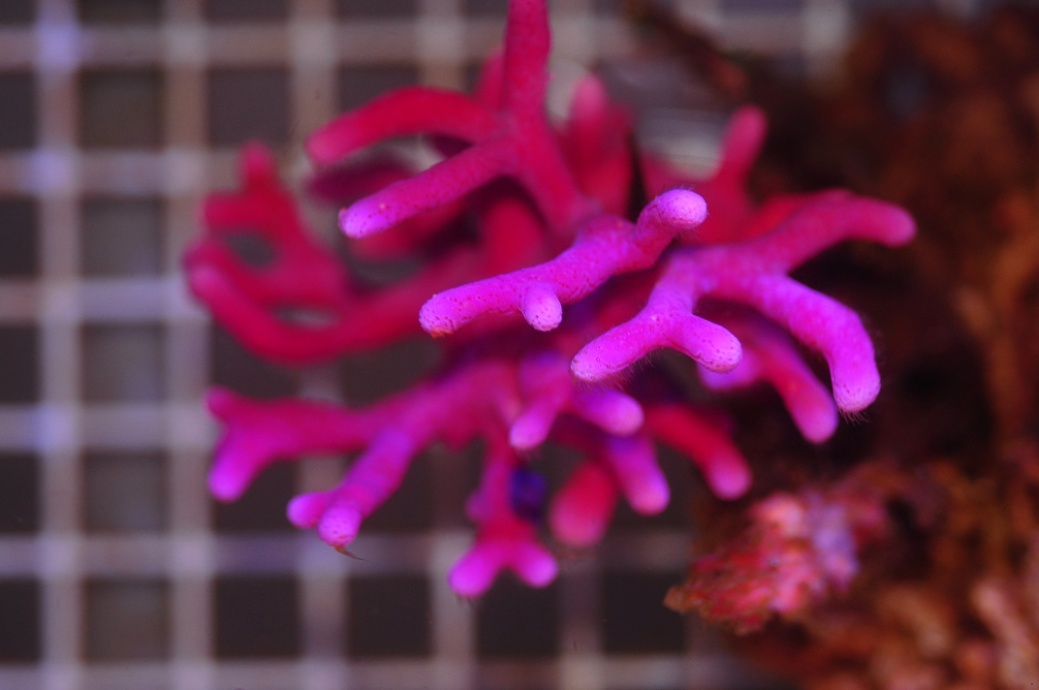
This Distichopora sp. has been growing in the DyMiCo system for a year. Although its growth rate seems low, calcifying tips and polyp extension are signs of health. Photographs by Jeroen Beuk (top) and Tim Wijgerde (below).
Many aquarists have been successful at maintaining gorgonians from the Caribbean, including Plexaura and Plexaurella spp. (Holaxonia). This success is most likely due to the presence of zooxanthellae, which is reflected in their brown coloration. Other, more colorful azooxanthellate gorgonians, however, have been proven far more difficult to maintain for prolonged time. Examples are species from the genera Menella, Swiftia and Guaiagorgia (Holaxonia), and Diodogorgia (Scleraxonia).
Gorgonian octocorals feed on a diverse array of particles, including zooplankton (e.g. copepods, rotifers), phytoplankton (e.g. diatom and dinoflagellate algae), protists (various microorganisms), protozoa (e.g. flagellates, ciliates) and detritus (particulate organic matter or POM). These particles range from approximately 1 to 2,000 μm in size, which underscores the importance of pico-, nano-, micro- and mesoplankton in the diet of azooxanthellate corals. Feeding preferences may greatly vary between species, where polyp size (diameter) may not be a good indicator of feeding preference, although it will determine maximum prey size a given species can ingest. Azooxanthellate gorgonians require intensive feeding with a wide variety of particles to meet their respiratory demands, including bacteria, protozoa, phyto- and zooplankton. Rotifers (Brachionus plicatilis), Artemia nauplii and small-sized copepods may be accepted by these corals, as well as various algae (diatoms and dinoflagellates).
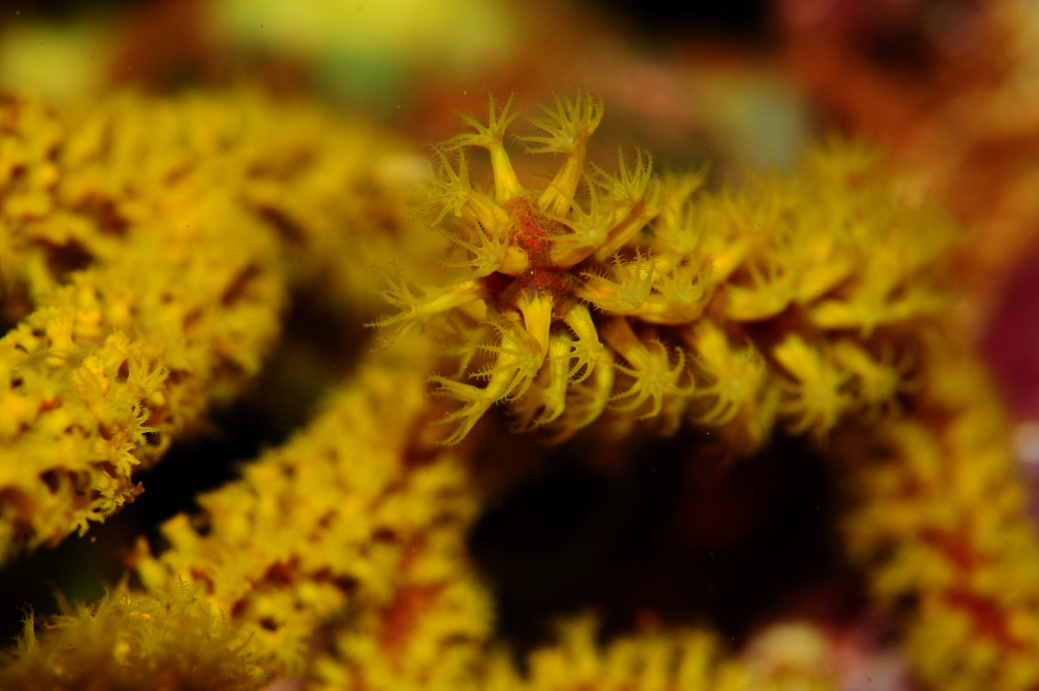
An Indo-Pacific Octocoral of the Menella genus (suborder Holaxonia, family Plexauridae). Each polyp is equipped with eight tentacles, from which fine structures called pinnula extend. These increase polyp surface area and filter efficiency.
In the wild, predation on zooplankton by corals is often quite limited due to low zooplankton abundance (Palardy et al. 2006; Sebens et al. 1996, 1998). However, experiments have shown that feeding rates can be enhanced by providing high concentrations of zooplankton in the aquarium, with an approximate linear relationship between plankton concentration and feeding rate (Clayton and Lasker 1982; Lasker 1982; Lewis 1992; Ferrier-Pagès et al. 1998, 2003; Houlbrèque et al. 2004; Wijgerde et al. 2011). Although zooplankton concentrations on reefs are quite low, prey is available constantly. In addition, crepuscular zooplankton migration leads to elevated zooplankton concentrations at night, which can increase fivefold (Yahel et al. 2005). From this it becomes clear that small amounts of food items should be available throughout the day and night. This may be a major part of the successes obtained with DyMiCo.
Several gorgonian species, including Guaiagorgia sp., were introduced in one of the DyMiCo systems. All colonies exhibited regular polyp expansion and clearly responded to feedings. Growth was discernible although growth rates were not determined. At this time, it seems these azooxanthellate corals can be succesfully maintained in aquaria equipped with DyMiCo.

An unidentified specimen, possibly from the Scleraxonia suborder, with fine, densely packed branches and small polyps. Gorgonians from this group have well-developed sclerites that can be seen as white grains imbedded in the soft tissue of the corals. These provide the colony with strength so that it can withstand drag forces induced by water currents.
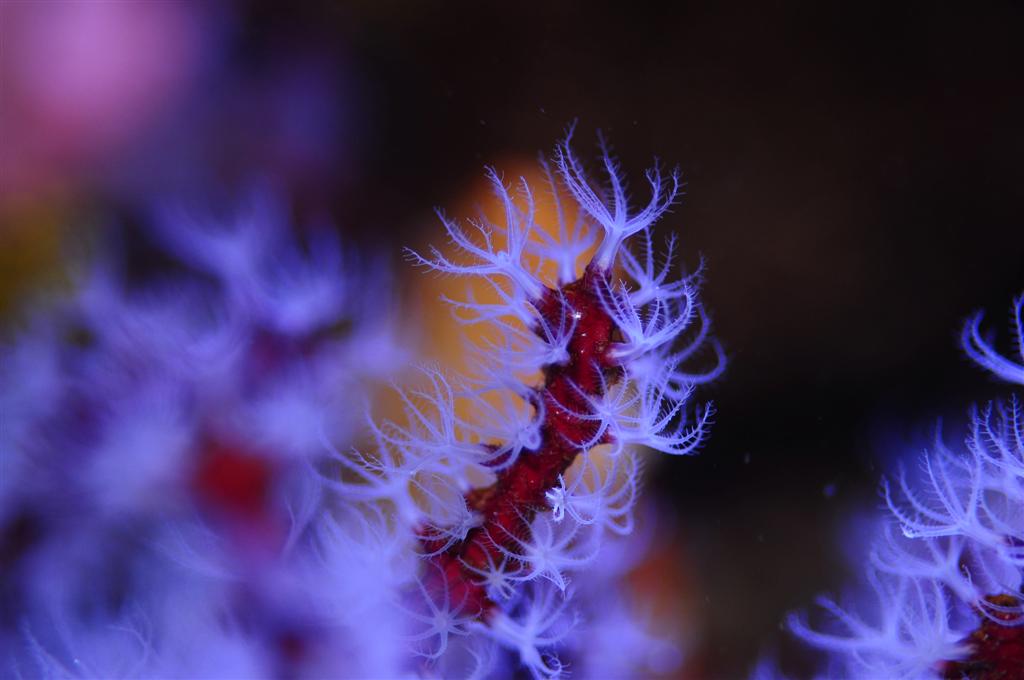
Gorgonians such as these Diodogorgia nodulifera (suborder Scleraxonia, family Anthothelidae) specimens are octocorals that require adequate water motion and constant feeding with zooplankton to meet their metabolic demands.
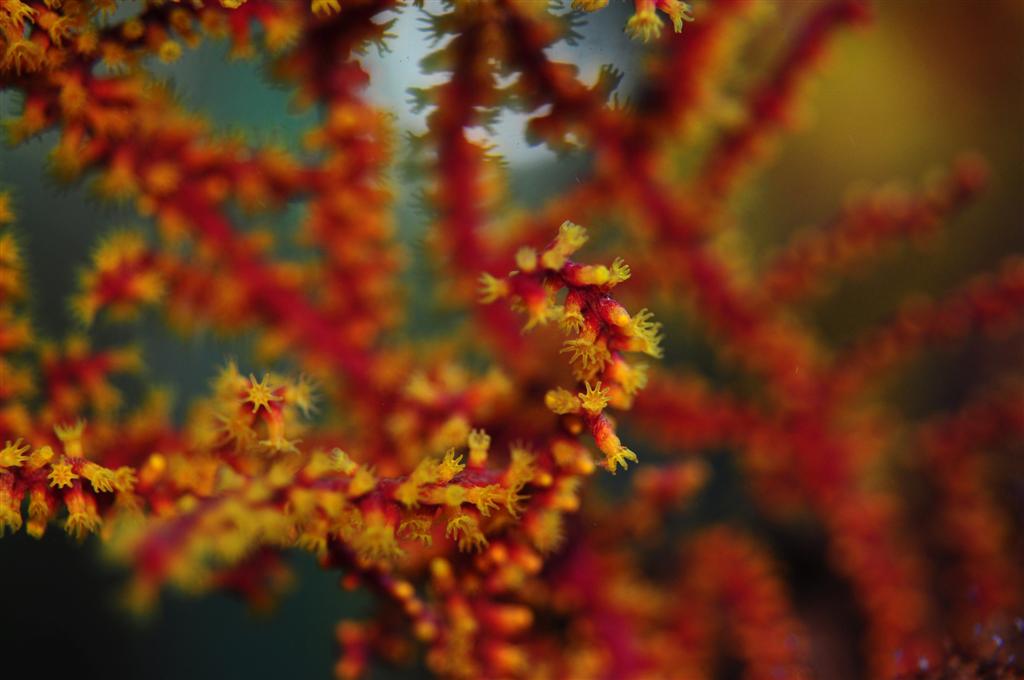
An Astrogorgia specimen (suborder, Holaxonia, family Plexauridae) with well-developed sclerites and conspicuous yellow polyps.
Crinoids
The successful husbandry of crinoids has been an aspiration for many aquarists, and so far the results have been dismal. To the best of my knowledge, there are no reports of crinoids surviving for prolonged time in closed systems, let alone reproducing. It is evident that in the average aquarium, these specialized animals do not encounter prey items in sufficient quantities to survive. In most cases, these animals slowly lose their arms until only the center part or calyx of the body remains. This, in turn, slowly disintegrates until only bony fragments called ossicles are left.
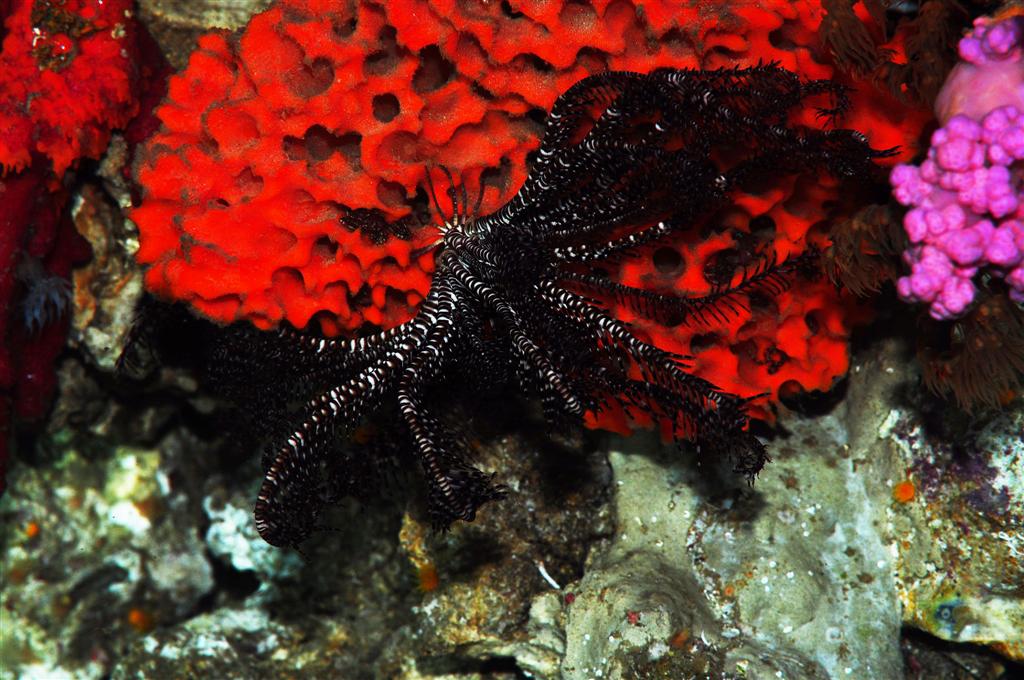
Crinoids actively filter food particles from the water, including zooplankton. Their survival rate in the aquarium is dismal at best.
Crinoids are true suspension feeders and grab small food particles from the water by using their podia or tube feet. The podia secrete mucus to which food items adhere, after which they are propelled to the so-called ambulacral groove. These food grooves run along the animal’s arms and are lined with cilia, tiny beating hair-like structures which transport mucus-imbedded food particles to the central mouth. Crinoids predominantly feed on nano- and microplankton, including unicellular organisms (e.g. foraminiferans), phytoplankton (e.g. diatoms) and zooplankton (e.g. rotifers, mollusk larvae and copepods) (Rutman and Fishelson 1969; Kitazawa et al. 2007).
Three unidentified crinoids (order Comatulida) were introduced in one of the systems, after which their behavior and survival were recorded over a one-year period. These echinoderms seemed to thrive and had their arms extended throughout the day. At this time, they remain in good condition.
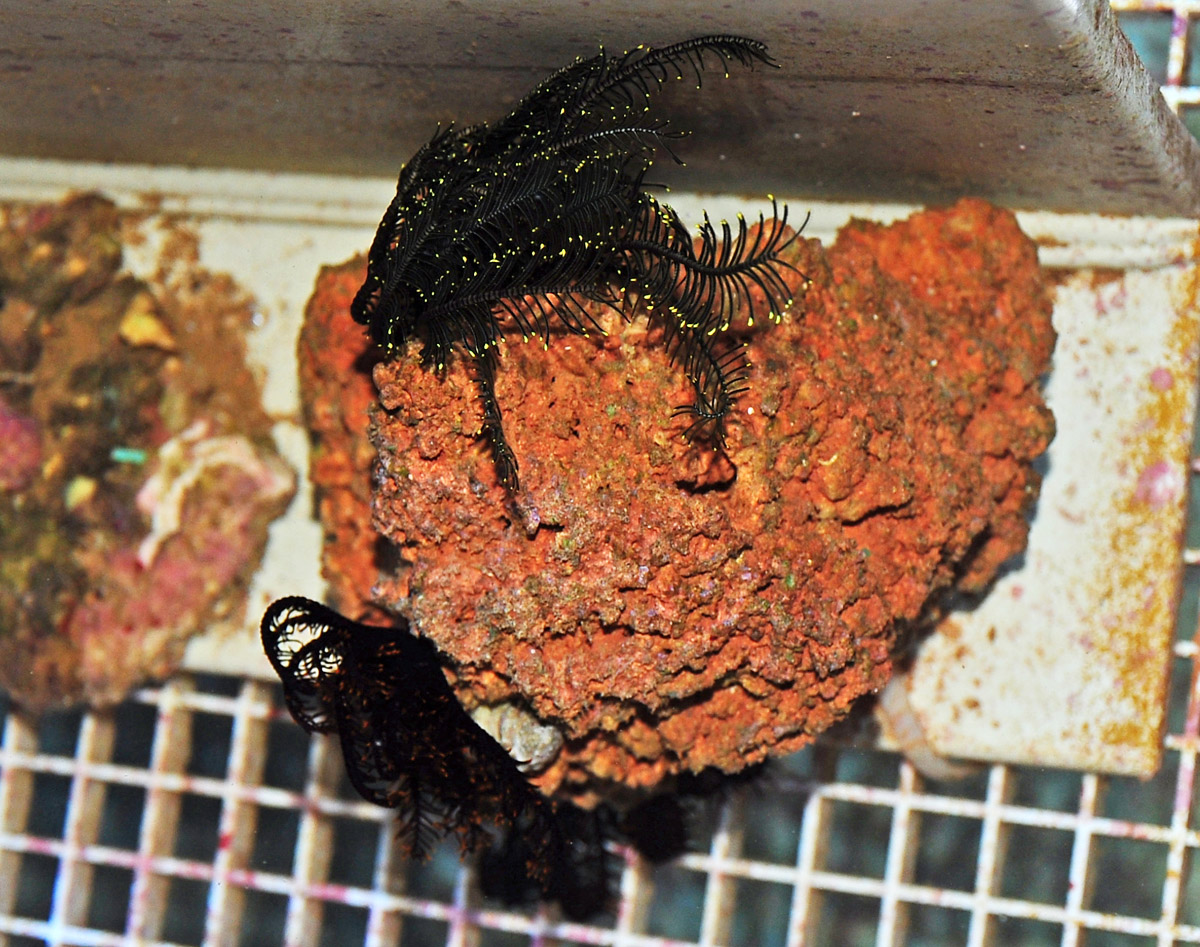
Crinoids seem to do well in DyMiCo-filtered aquaria. These specimens extend their arms after every plankton feeding.
Other observations
Next to the observations described above, there have been other interesting developments. Azooxanthellate octocorals, e.g. Dendronephthya and Scleronephthya spp., seem to be promising candidates for future testing in DyMiCo. A few months ago, an almost completely withered red-colored Scleronephthya sp. was introduced in one of the systems. It slowly recovered into several small colonies with clearly expanded polyps. Although these results are preliminary, these octocorals may thrive provided that sufficient feeding with (live) phytoplankton cultures is maintained, as they are predominantly herbivorous (Fabricius 2005).
Although many aquarists have witnessed coral spawning and release of larvae (planula) in closed systems, this is usually unpredictable and sparse. Several months after the introduction of several small-sized Pocillopora damicornis colonies, a well-known scleractinian coral, I was happy to witness countless offspring on the system walls. These new colonies, also referred to as recruits, arose from the settlement of larvae that were released by the corals after the lights went out. The larvae, after having chosen a suitable spot, metamorphose into a so-called primary polyp that start dividing and grow their own colony. Although I was not able to determine whether these new recruits originated through self-fertilization or polyp bailout, the survival of planula in large numbers is promising.
On the system walls, many unidentified sponges, polychaete tube worms, and other life started growing after several months, similar to what one might find in a dark filtration compartment. On the live rock that was introduced, different species of macro algae and Porites corals developed. Various unidentified species of nudibranchs were found as hitchhikers on the rock. Although these phenomena are common even in heavily skimmed Berlin systems, the explosion of life in these aquaria is remarkable.
Final comments
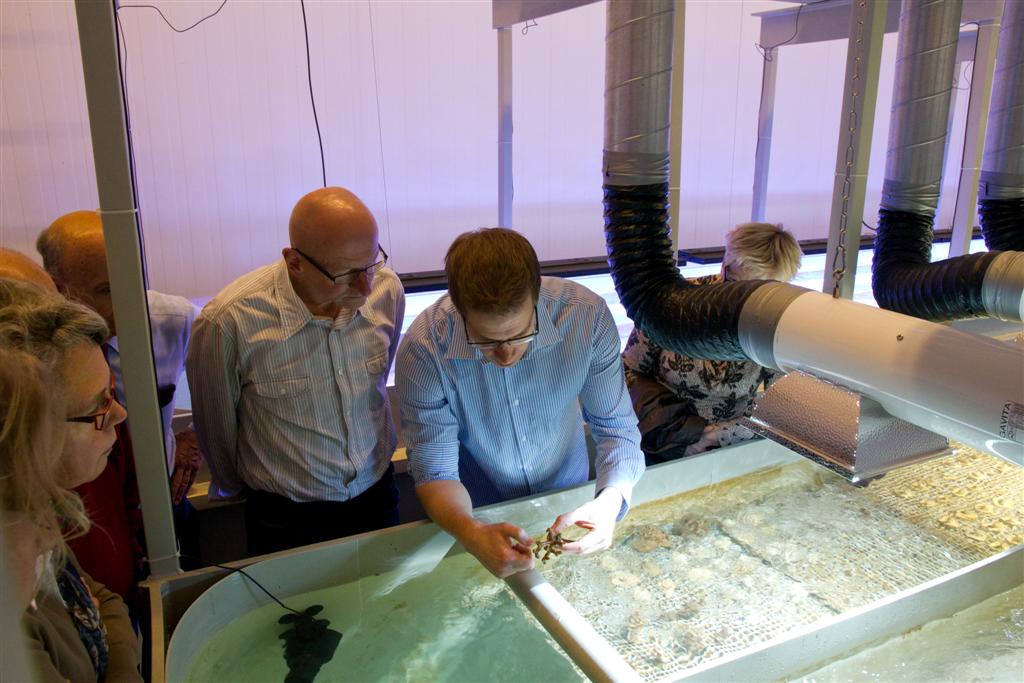
Exotic marine life captivates the minds of many – here, the author shows a piece of coral (Stylophora pistillata) to his family. Photograph by Jeroen Beuk.
It is exciting to witness the health and growth of marine invertebrates which have proven to be notoriously difficult to maintain in aquaria. Their progress in DyMiCo systems will be monitored in the future, and hopefully these organisms will continue to grow and flourish. Perhaps, they may someday even reproduce sexually. Despite the complete lack of water changes, water quality of the DyMiCo systems remained high, adding to the environmentally friendly nature of DyMiCo. Although water analysis has indicated that supplementation of some trace elements including strontium may be required, most water parameters remained close to optimal values.
It is not yet clear whether this technology functions properly in home aquaria, but if it does, it could potentially change the future of the marine aquarium hobby. In that case, the spectrum of marine invertebrates that can be maintained at home would be drastically increased. I would like to end part two of this article by expressing the hope that within several years, we will have mastered the husbandry and reproduction of many (in)vertebrates that are relevant to us as aquarists, hobbyists, scientists and breeders. This will prevent us from taking endangered species from their native habitats, which will relieve some of the pressure on wild populations.
Acknowledgments
I would like to thank Peter Henkemans at EcoDeco and Michaël Laterveer at Bluelinked for their support during the writing of this two-part article.
Tim Wijgerde, M.Sc. is a Ph.D. candidate at Aquaculture and Fisheries, Department of Animal Sciences, Wageningen University. His research focuses on the heterotrophy of scleractinian corals. To find out more about the Dynamic Mineral Control technology, visit the official website at www.ecodeco.eu.
References
- Clayton WS, Lasker H (1982) Effects of light and dark treatments on feeding by the reef coral Pocillopora dam- icornis. J Exp Mar Biol Ecol 63:269-279
- De Goeij JM, De Kluijver A, Van Duyl FC, Vacelet J, Wijffels RH, De Goeij AFPM, Cleutjens JPM, Schutte B (2009) Cell kinetics of the marine sponge Halisarca caerulea reveal rapid cell turnover and shedding. Journal of Experimental Biology 212:3892-3900
- Fabricius K, Alderslade P (2001) Soft Corals and Sea Fans – A comprehensive guide to the the tropical shallow-water genera of the Central-West Pacific, the Indian Ocean and the Red Sea. Australian Institute of Marine Science, Townsville. 264 p
- Fabricius KE, Genin A, Benayahu Y (1995) Flow-dependent herbivory and growth in zooxanthellae-free soft corals. Limnology and Oceanography 40:1290-1301
- Ferrier-Pagès C, Allemand D, Gattuso JP, Jaubert J, Rassoulzadegan F (1998a) Microheterotrophy in the zooxanthellate coral Stylophora pistillata: Effects of light and ciliate density. Limnol Oceanogr 43:1639-1648
- Ferrier-Pagès C, Witting J, Tambutté E, Sebens KP (2003) Effect of natural zooplankton feeding on the tissue and skeletal growth of the scleractinian coral Stylophora pistillata. Coral Reefs 22:229-240
- Houlbrèque F, Tambutté E, Richard C, Ferrier-Pagès C (2004) Importance of a micro-diet for scleractinian corals. Mar Ecol Prog Ser 282:151-160
- Kitazawa K, Oji T, Sunamura M (2007) Food composition of crinoids (Crinoidea: Echinodermata) in relation to stalk length and fan density: their paleoecological implications. Marine Biology 152:959-968
- Lasker HR, Syron JA, Clayton WS (1982) The feeding response of Hydra viridis: effects of prey density on capture rates. Biol Bull 162:290-298
- Lewis JB (1992) Heterotrophy in corals: Zooplankton predation by the hydrocoral Millepora complanata. Mar Ecol Prog Ser 90:251-256
- Martin KH, Knauer GA (1973) The elemental composition of plankton. Geochimica et Cosmochimica Acta 37:1639-1653
- Palardy JE, Grottoli AG, Matthews KA (2006) Effect of naturally changing zooplankton concentrations on feeding rates of two coral species in the Eastern Pacific. J Exp Mar Biol Ecol 331:99-107
- Rutman J, Fishelson L (1969) Food composition and feeding behavior of shallow-water crinoids at Eilat (Red Sea). Marine Biology 3:46-57
- Sebens KP, Grace SP, Helmuth B, Maney EJ, Miles JS (1998) Water flow and prey capture by three scleractinian corals, Madracis mirabilis, Montastrea cavernosa and Porites porites, in a field enclosure. Mar Biol 131:347-360
- Sebens KP, Vandersall KS, Savina LA, Graham KR (1996) Zooplankton capture by two scleractinian corals, Madracis mirabilis and Montastrea cavernosa, in a field enclosure. Mar Biol 127:303-317
- Spotte S (1992) Captive seawater fishes: science and technology. J.Wiley & Sons Inc. 942 p
- Swain TD, Wulff JL (2007) Diversity and specificity of Caribbean sponge-zoanthid symbioses: a foundation for understanding the adaptive significance of symbioses and generating hypotheses about higher-order systematics Biological Journal of the Linnean Society 92:695-711
- Wijgerde T, Diantari R, Lewaru MW, Verreth JAJ, Osinga R (2011) Extracoelenteric zooplankton feeding is a key mechanism of nutrient acquisition for the scleractinian coral Galaxea fascicularis. The Journal of Experimental Biology 214:3351-3357
- Yahel R, Yahel G, Berman T, Jaffe JS, Genin A (2005) Diel pattern with abrupt crepuscular changes of zooplankton over a coral reef. Limnol Oceanogr 50:930-944
0 Comments