Currently, large numbers of fish, corals and other invertebrates are exported annually for the American, European and Asian markets. It is paramount that this trade is sustainable, for coral reefs as well as for local tropical communities. This poses a significant challenge, as coral reefs are under great pressure from pollution, overfishing, coastal development, global warming and ocean acidification.
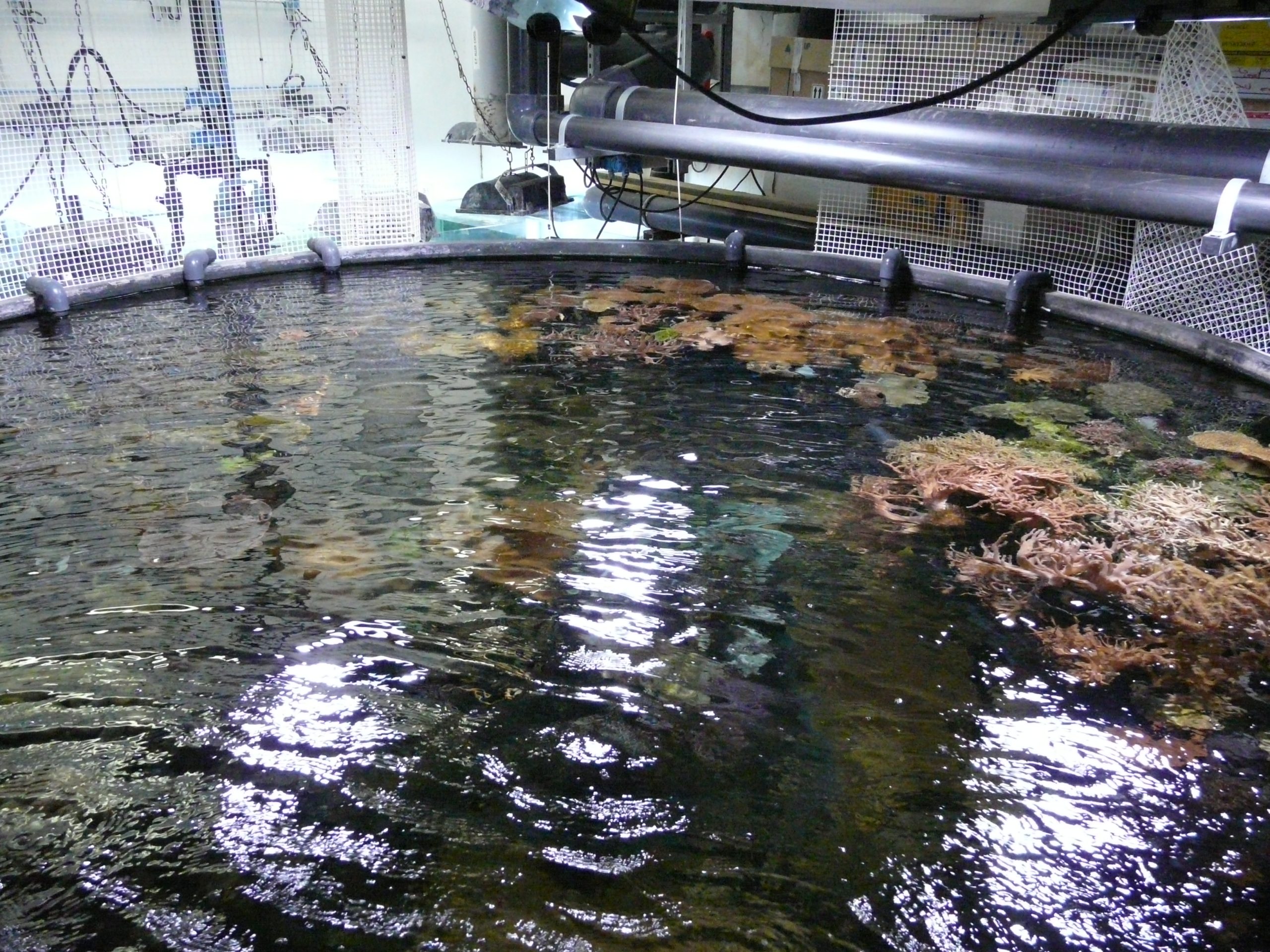
An increasing number of zoos and public aquaria is growing corals behind the scenes (photograph: Tim Wijgerde, NAUSICAA, Boulogne-sur-Mer, France).
A sustainable trade involves coral mariculture by local communities, but also aquaculture in destination countries. Culturing corals in captivity could become increasingly important in the future, not only to meet market demand, but also to conserve corals that may go extinct in the wild. A good example of a conservation initiative is Amphibian Ark (amphibianark.org), a project which aims to prevent the extinction of endangered amphibians by breeding them in laboratories.
The culture of countless marine species will have to be upscaled significantly over the following years, due to increased demand. Quotas of wild-caught specimens, be it corals or fish, will possibly be reduced in the future. This change towards a more sustainable trade is already ongoing, and aquarium hobbyists play an important role in this process. Species such as clownfish and sea horses are bred regularly by specialists, who devote a great deal of time to their hobby. Many corals are being fragmented and traded on internet fora and events, and this has led to commonly available species in the trade. A serious limitation of coral propagation however, be it mariculture or aquaculture, is that it is mostly done via asexual reproduction. Below, I will explain why this could become a future problem.
Asexual reproduction
A marine aquarium which runs optimally quickly becomes a “victim” of its own success. Some corals grow out to impressive proportions, after which the colonies are subject to enthusiastic pruning. Fragmenting and selling so-called ramets or “frags” is an effective way to recuperate some of the investments made, and also allows for expansion of a captive coral population.
In the wild, corals are also fragmented constantly; animals and storms continuously break off fragile colonies. Corals also release parts of their colonies via a process called autotomy (from the Greek auto, or self, and tome, or severing). This can occur during periods of stress, and it is observed frequently in aquaria. Autotomy can be a last resort for a colony to survive, in the form of a released fragment which often completely regenerates. It is also a very useful way for a healthy colony to spread its genes.
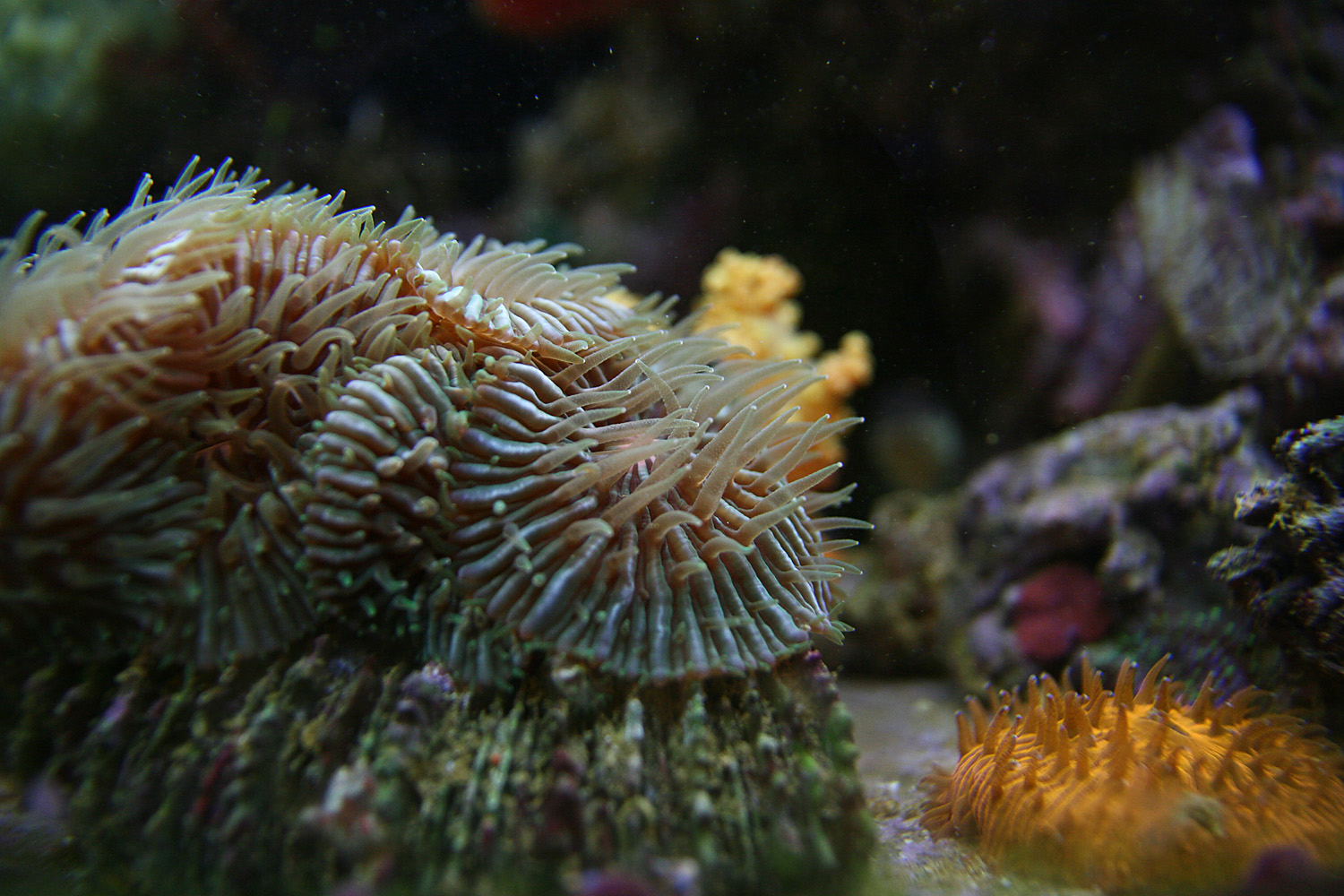
Fungia or mushroom corals display an unusual form of asexual reproduction; the formation of numerous daughter polyps from a dying parent polyp known as anthocauli. This strategy serves as a last resort, and ensures the survival of the individual (photograph: Jorick Hameter).
Biologically speaking, coral fragmentation is a form of vegetative, or asexual reproduction. Corals use many different strategies of asexual reproduction, including intratentacular budding, extratentacular budding, “dripping”, polyp bailout and the formation of anthocauli displayed by members of the Fungiidae family.
Although fragmentation is a useful way to propagate corals, a major disadvantage is that it does not results in genetic diversity. A coral fragment is an exact genetic copy of its parent colony, and is thus considered a clone. Clones behave exactly the same under equal conditions, which means they are equally strong, but also equally weak as their parent colonies. Imagine a coral colony sensitive to salinity fluctuations being fragmented into many small colonies. When these fragments are aquacultured, this latent weakness will present itself sooner or later. A population of 1,000 Acropora formosa clones can be completely eradicated by a faulty water top-off system. Another example is the well-known pink/purple Stylophora pistillata, which has been fragmented and traded for many years in Europe. This clonal line is known to be hardy, and tolerates a wide range of salinities, light intensities and nutrient levels. However, I personally have lost a few kilograms of this clone within a weak, possibly due to a bacterial infection. A colleague of mine had the same problem with this genotype, and lost about half his population a few months later. Only strong UV filtration of the water seemed to stop the spreading of the disease. These problems occur when latent weaknesses suddenly become apparent.
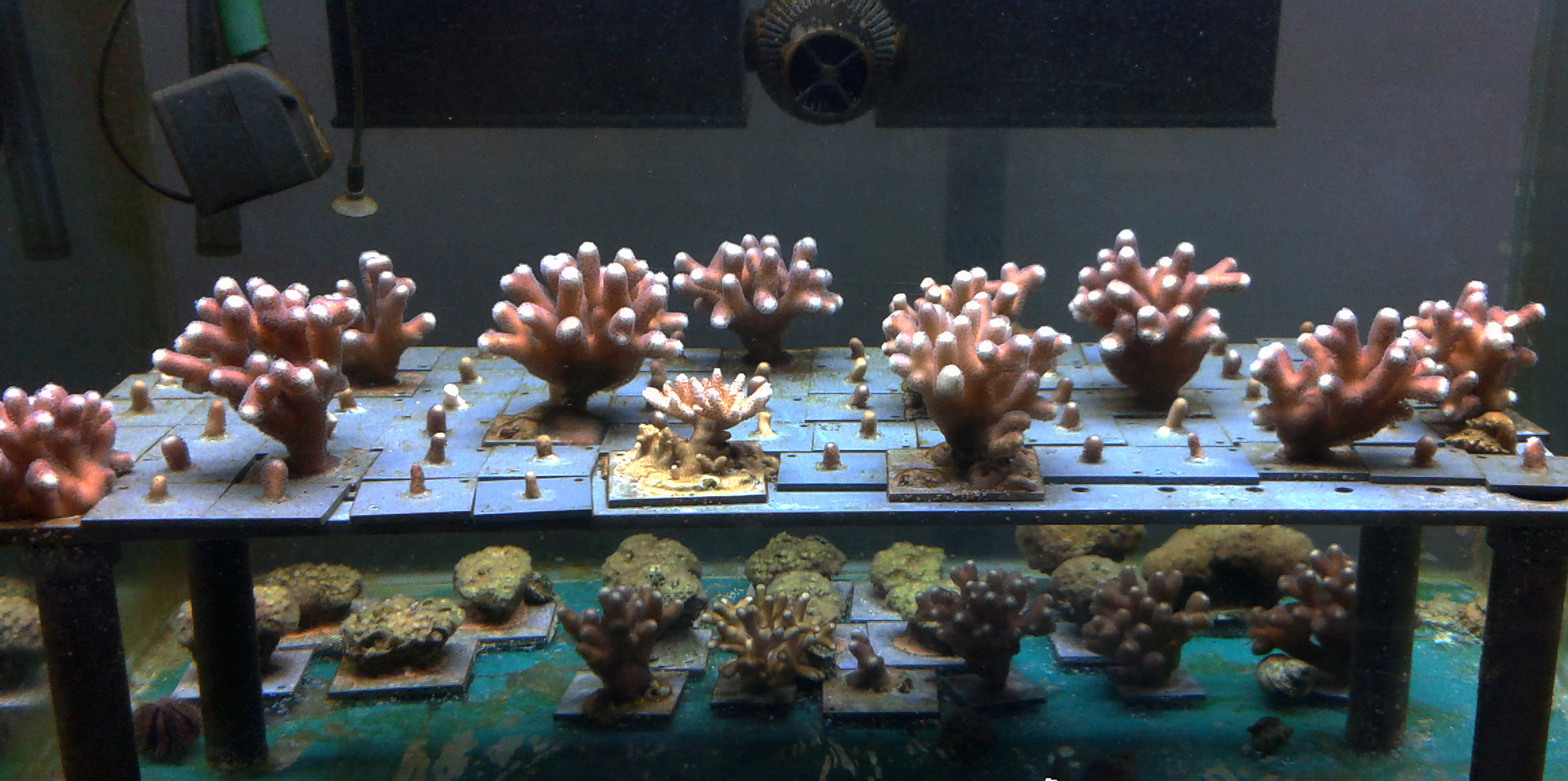
These pink Stylophora pistillata clones are hardy, but do have weaknesses. Without genetic diversity, this small coral population may be completely eradicated by a sudden disturbance (photograph: Tim Wijgerde, Wageningen University, Wageningen, The Netherlands).
“A coral fragment is an exact genetic copy of its parent colony, and is thus considered a clone. Clones behave exactly the same under equal conditions.”
If the coral trade becomes highly dependent on aquacultured corals in the future, due to changes in legislation, it is paramount that genetic diversity within each species is maintained. This could prevent major losses of coral species within the trade, for example due to bacterial or parasitic infections. A genetically diverse coral population, with therefore different resistive qualities, will have a higher chance of survival in the long run. This is because after each disturbance, it is likely that some individuals will survive as they have an innate resistance to it. There is only one natural way of generating genetic diversity, and that is through sexual reproduction.
Sexual reproduction
Sexual reproduction involves the fusing of gametes, or ova (eggs) and sperm, which together generate a new individual. Eggs and sperm are produced via a process known as meiosis, during which the DNA in chromosomes is “blended”, resulting in new combinations of genes. Each gene is a small section of DNA, which encodes a specific RNA molecule and/or protein with essential functions inside or outside a living cell. Genes and their encoded RNA/proteins have a major impact on the growth rate, colouration, size or any other attribute of an organism. The overall genetic composition of an individual therefore determines its characteristics. Every time an ovum and sperm fuse, they generate a new combination of genes, thereby creating a completely unique individual. On a large scale, this process results in a genetically diverse population, with individuals able to respond to a wide array of disturbances. The starting population should of course be sufficiently large, to prevent too much “recycling”, or inbreeding, of the same genes. Corals produce gametes as well, and when starting out with several hundreds of colonies, a gene pool may be maintained which is reasonably diverse. Collecting “fresh” specimens from the wild every now and then may serve to enrich such a gene pool.
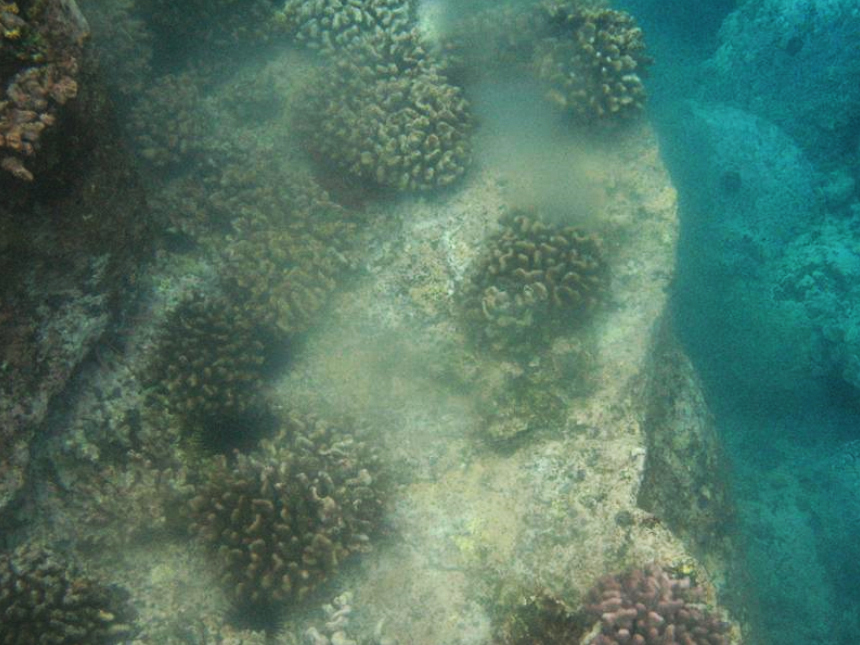
Sexual reproduction involves the fertilization of eggs with sperm. Corals have adopted several strategies for successful reproduction, such as releasing gametes in concert on an annual basis. Here, Pocillopora meandrina colonies are releasing eggs and sperm in Hawaii (photograph: Denise Ulrich, courtesy of Dana Riddle).
Of course, sexual reproduction sometimes yields weak individuals, when genetic defects or weaknesses are passed on. This is especially true if weaknesses are transferred by both parent colonies to one individual, such as sensitivity to bleaching and salinity fluctuations. In nature, these individuals may not last very long, which may be equally true in the aquarium. The survival of favorable genes which yield better-adapted individuals is often called “survival of the fittest”, a sentence which was first coined by Herbert Spencer (1820-1903) in his book Principles of Biology (1864). Similarly, Charles Darwin, father of the evolution theory, called the selection of stronger individuals over weaker ones “natural selection”. In the end, sexual reproduction will yield a diverse population in which the stronger, or better-adapted individuals will survive. In terms of coral aquaculture, we might lose a portion of our offspring colonies, which is acceptable and even paramount to obtaining strong populations. Maintaining genetically diverse captive populations seems the most ideal scenario for the future of coral aquaculture. This holds especially true if such colonies are to be reintroduced to the wild, as part of reef restoration programmes.
Next to maintaining genetic diversity, ageing may be a second major incentive to commence large-scale sexual coral reproduction. It is known that many organisms age, which may be defined as a decreased functional state in genetic makeup, physiology, morphology and reproduction. There is some evidence which supports the idea that cnidarians age, including corals. On the other hand, they have been found to produce telomerase, an enzyme which inhibits ageing by repairing chromosome ends. This enzyme is also active in tumour cells, which makes these cells immortal. Corals also have effective DNA repair mechanisms, which would also reduce ageing caused by DNA damage. Although it is not yet clear if and which cnidarians truly age, sexual reproduction would “reset the clock” for each coral generation, preventing ageing from having negative effects.
Reproductive strategies
Corals have developed an array of strategies for effective reproduction. In addition to the diverse methods of asexual reproduction, different means of sexual reproduction exist. These can be categorized based on coral sexuality, and the way gametes are released and fertilized.
Gonochorism
A number of coral families harbors species which are gonochoric, which means males and females exist as separate individuals. This phenomenon is displayed by many animals, including mammals, birds, reptiles, amphibians and fish. The Dendrophylliidae family contains several genera which utilize this reproductive strategy, such as Turbinaria, Tubastrea, Leptopsammia, Heteropsammia, Rhizopsammia, possibly Duncanopsammia, Dendrophyllia and Balanophyllia. These gonochoric animals mainly brood their eggs, which exceptions such as the genus Turbinaria, containing species which release gametes into the water (see broadcasting).
Hermaphroditism
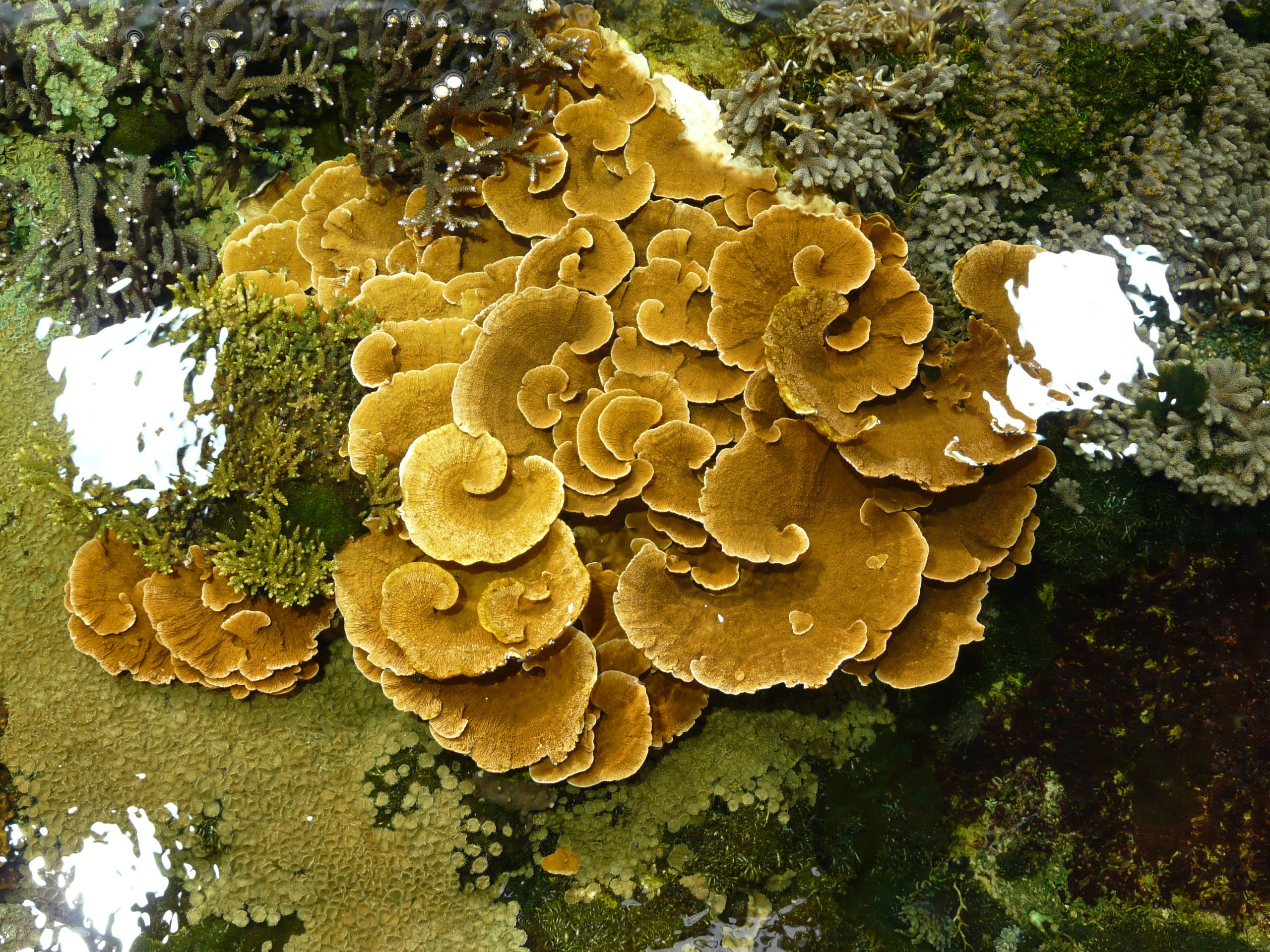
Montipora spp., such as this colony in an artificial lagoon, are hermaphroditic broadcast spawners (photograph: Tim Wijgerde, NAUSICAA, Boulogne-sur-Mer, France).
The majority of stony coral species, such as Acropora spp., is hermaphroditic or bisexual. Polyps of such colonies display both active male and female gonads, called testes and ovaries, respectively. These species often release egg/sperm bundles during summer periods, after which the sperm cells detach from the bundles and fertilize the eggs (see broadcasting). Some species also display self-fertilization, or selfing. This feature seems to occur amongst some brooding corals. This may happen within the same polyp, or between polyps of the same colony.
In addition, several species display a unique form of sexuality which involves a transition from one sex to the other, called sequential hermaphroditism. This transition can occur from male to female, called protandry, or from female to male, called protogyny. This phenomenon is often found amongst marine fishes, including anemone fish (Amphiprion and Premnas spp.). The Fungiid corals Fungia repanda and Ctenactis echinata also demonstrate this ability, with the latter species being able to switch back and forth. At any given time, sequential hermaphrodites only have one active gonad type, whereas the other lies dormant and does not produce gametes. For example, a female mushroom coral may have active ovaries and produce oocytes, and have dormant testes at the same time which may become active the following year. Whereas corals may switch sex every season, fish such as pygmy angels can undergo sex change within eight weeks.
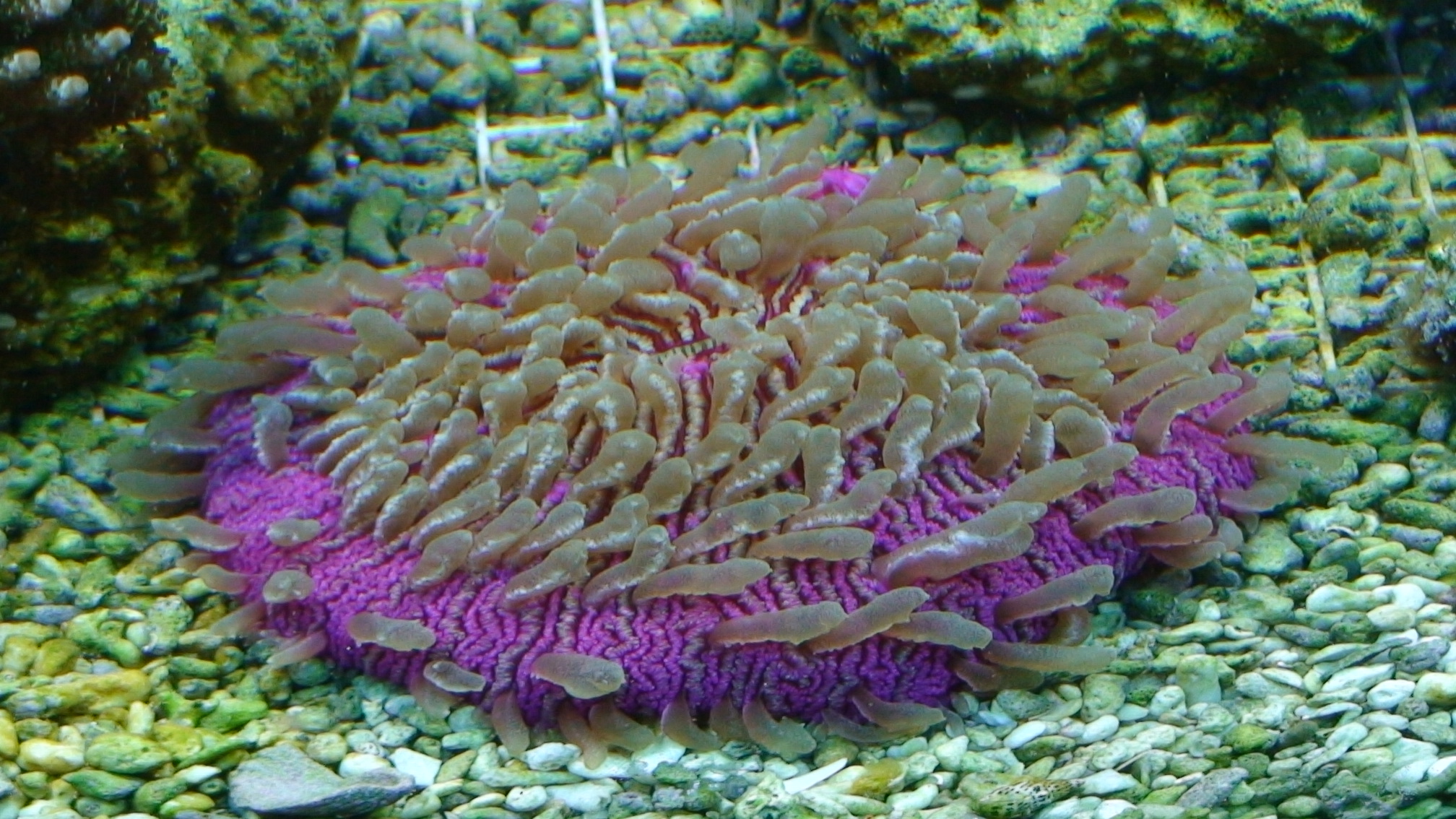
Mushroom corals predominantly exist as solitary polyps, and may grow up to 40 cm (16 inches) in diameter. Species from the Fungiidae family may change sex, with small or stressed individuals typically being male. Sex change is a powerful evolutionary adaptation, allowing corals to maintain high fecundity levels even during periods of stress (photograph: Tim Wijgerde, Rotterdam Zoo, Rotterdam, The Netherlands).
(Sequential) hermaphroditism may have evolved because it is an effective strategy to sexually reproduce, as any two individuals from the same species may be able to fertilize one another. This circumvents the problem of an incidental lack of either males or females in a given population. Second, sequential hermaphroditism allows an individual to produce sperm instead of eggs during times of stress, which is more economical as sperm require less energy to produce. Eggs have to be loaded with protein and fats, which is undesirable for any organism during times of physiological stress. It has been found that the coral Ctenactis echinata reproduces as a male when it is small or stressed by sedimentation or bleaching.
Parthenogenesis
Parthenogenesis (from the Greek parthenos, or virgin, and genesis, or birth) occurs when egg cells undergo cellular mitosis and start embryonic development without prior fertilization. This yields an individual which is similar to its parent, as no genetic contributions have been made by a male partner (it is not cloning, as genetic recombination still yields a genetically dissimilar animal). The advantage of this strategy is that female organisms are still able to reproduce in the absence of males. The major downside is that the genetic variation of a given population decreases as a result. This decreases the overall fitness of the population, which means it is less able to adapt to changing conditions. Hereditary defects may also become more apparent. Parthenogenesis seems to be quite uncommon amongst corals, and has been reported for Pocillopora damicornis and Porites sp.
Broadcast spawning
Many coral species expel their gametes into the water column annually, during a process called broadcast spawning. This reproductive strategy is common amongst species from the Faviidae, Euphyllidae and Acroporiidae families. Members from the Faviidae and Acroporiidae families, such as Favia, Favites, Acropora and Montipora spp. are usually hermaphroditic, and release egg/sperm bundles into the water through the oral pores of their polyps. Members of the Euphyliidae are predominantly gonochoric, such as Euphyllia ancora and E. divisa. E. glabrescens, the Torch Coral, is an exception and has been found to be a hermaphroditic brooder.
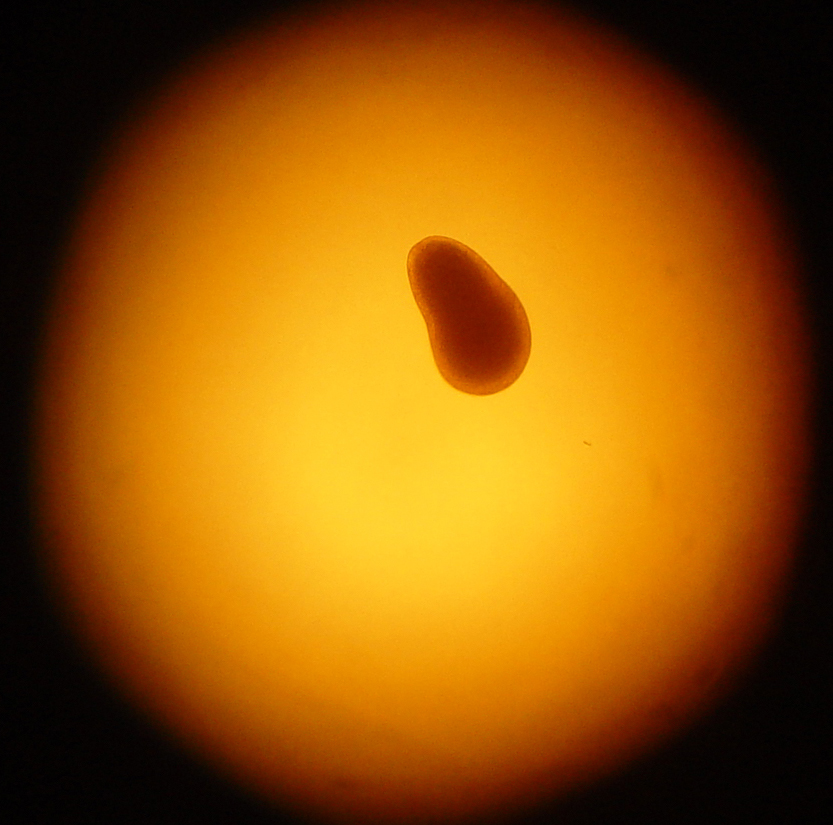
A four day-old Trachyphyllia geoffroyi planula larva. Coral larvae swim by beating cilia; these are countless tiny hairs which protrude from epidermal cells. Their swimming speed is quite low; about 2 mm (0.08 inches) per second! Their limited locomotory behaviour allows the larvae to scour the available substrate for a suitable location for settlement. The oral pore always develops at the posterior end, or backside of the larvae, which is the smaller top portion in the picture (photograph: Rachel Jones, London Zoo, London, UK).
Broadcasting species usually produce impressive amounts of gametes, of which only a small fraction yields offspring. The fecundity of these corals is therefore very high under ideal circumstances. Their offspring, in the shape of planula larvae, are an important part of the plankton present in the ocean and are consumed by many organisms such as crustaceans and whales. Eventually, a small portion (possibly less than 1%) settles onto a reef which may be hundreds of kilometers away from its location of origin.
“Many coral species expel their gametes into the water column annually, a process called broadcast spawning. This reproductive strategy is common amongst species from the Faviidae, Euphyllidae and Acroporiidae families.”
After external fertilization of eggs in the water column, which often display positive buoyancy, planula larvae develop after 24 to 48 hours. These are usually significantly smaller (75 – 500 micrometer) compared to larvae which are released by brooding species. For this reason, these larvae have developed ways of feeding themselves during this delicate stage.
Larvae which develop from average-sized eggs (around 0.5 mm) mainly feed on egg yolk, and are called lecithotrophic. Smaller larvae, which develop from smaller eggs depend on external nutritive sources and photosynthesis. In that case, the larvae are considered planktotrophic.
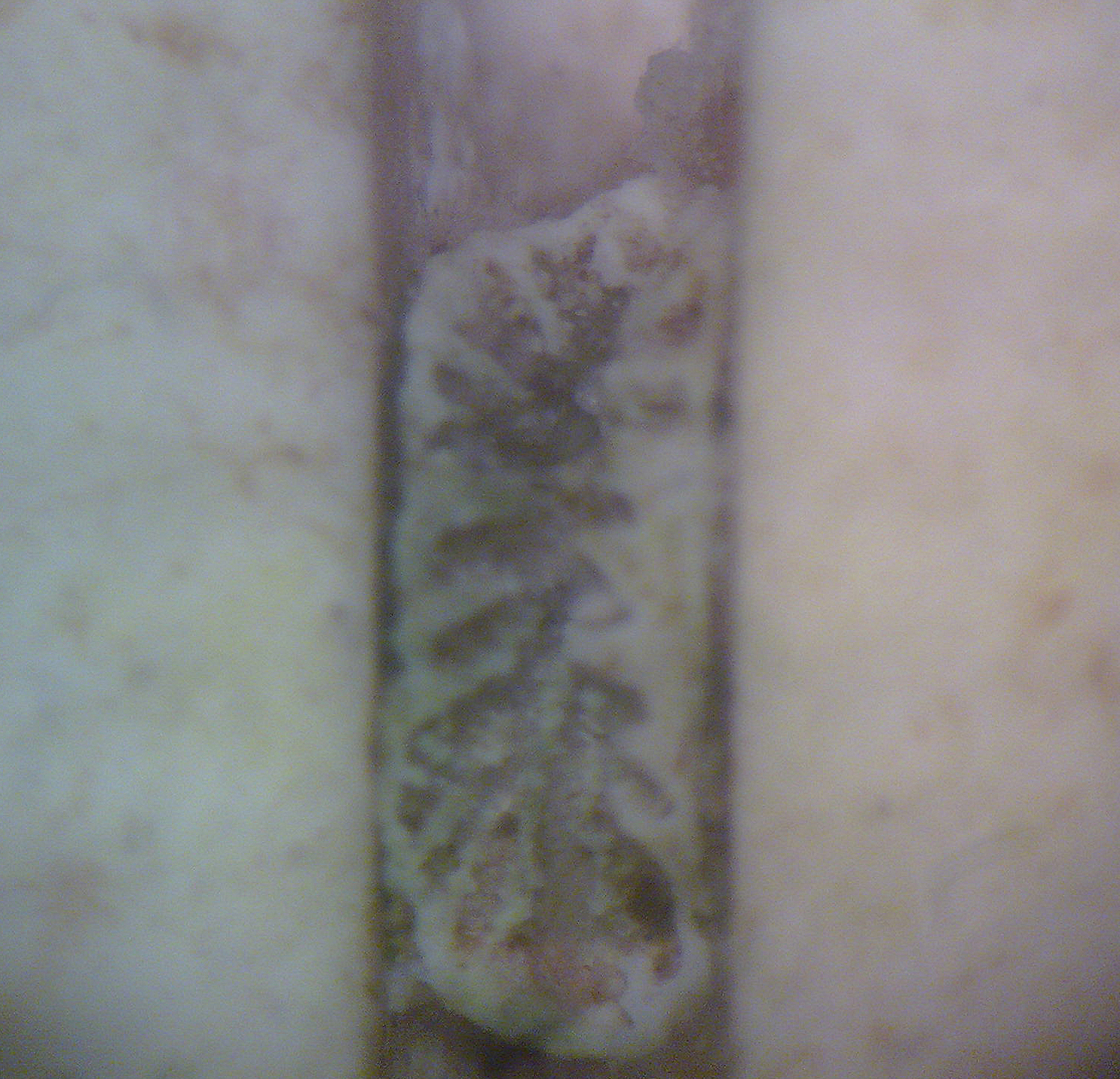
“Planula larvae of broadcasting species may spend from several days to several weeks as plankton. This has major consequences for the distribution of species and the recovery of damaged reefs.”
Coral larvae swim around actively by means of cilia; these are tiny hairs which protrude from the epidermal cells of the larvae. Some species produce larvae which develop oral pores during their planktonic stage, such as several Fungia and Acropora spp. This allows these larvae to ingest plankton and detritus from the water, which provides them with energy. Zooxanthellae, which are symbiotic algae from the genus Symbiodinium, are also ingested in this way. Other species produce larvae which do not develop a mouth until after metamorphosis into a primary polyp, which occurs immediately after larval settlement. An example is the threatened Elkhorn coral from the Caribbean, or Acropora palmata.
A young Trachyphyllia geoffroyi polyp which died several weeks after settlement. The larvae settled within a groove of a ceramic tile, which is a preferable location for many species. Reproduction of this species has been unsuccessful until now, and larvae of this species possibly require infection with zooxanthellae during this stage (photograph: Rachel Jones, London Zoo).
Planula larvae of broadcasting species may spend from several days to several weeks as plankton. This has major consequences for the distribution of species and the recovery of damaged reefs, which may be reseeded as a result of planula dispersion. This long so-called larval competency period allows for large distribution of many coral species.
Brooding
Brooding species expel sperm cells just like broadcasting species, however fertilization of ova occurs internally. Male or hermaphrodite colonies release sperm cells, of which a small proportion finds its way to female or other hermaphrodite specimens of the same species, after which fertilization takes place. Next, the eggs develop into relatively large larvae which are released through the oral pores at sizes between 500 – 2,000 m (0.5 – 2 mm). In contrast to broadcasters, brooding species produce little eggs and large, well-developed larvae. This strategy is thus based on the principle of “quality instead of quantity”. The large larvae are often negatively buoyant, and usually settle within 1 or 2 days. This poses great advantages for the aquaculture of such species, as offspring has far less chances of being skimmed off or being removed by protein skimmers. Examples of brooding species are Pocillopora damicornis, Favia fragum and Tubastraea coccinea.
Transmission of zooxanthellae
Many coral species have formed a mutualistic relationship with dinoflagellates from the genus Symbiodinium, which provide their host with important nutrients. These dinoflagellates are colloquially known as zooxanthellae. When reproducing, many coral species transfer their zooxanthellae to their offspring via ova. This is very common amongst brooding species, in contrast to broadcasters, which often produce aposymbiotic eggs (i.e. devoid of zooxanthellae). In nature, exceptions to any rule exist, and this holds true for corals as well. Many species from the genera Montipora are broadcast spawners which transfer zooxanthellae through released eggs. This also goes for Pocillopora meandrina, P. eydouxi and P. verrucosa.
Transfer of zooxanthellae to offspring through ova is called vertical transmission, as this occurs from one generation to the next. This process is typical amongst brooding corals, and has been found for about 15% of all photosynthetic coral species. When aposymbiotic larvae take up zooxanthellae from the water, this is called horizontal transmission; each generation of offspring therefore has to take up new dinoflagellates. Both strategies have their (dis)advantages. Vertical transmission of zooxanthellae may result in higher survival rates of coral larvae, as they receive vital nutrients from photosynthesis. This only goes for situations where water temperatures do not exceed ~30°C (~86°F), as higher temperatures cause many zooxanthellae to disintegrate and release toxic oxygen radicals into the larval tissue. This leads to larvae mortality, and this phenomenon may be the reason why many species do not transfer zooxanthellae to their offspring, circumventing the useful but sometimes precarious symbiosis at early coral life. Another downside of vertical transmission is low flexibility, as the larvae do not get to obtain other zooxanthellae “species”, or clades, which may be better adapted to high temperatures. This is especially true if larvae end up at new locations where water temperatures are higher, which may render them more sensitive to bleaching episodes. Horizontal transmission has the advantage of being more flexible, which could be highly beneficial if larvae drift to warmer areas. Here, they may take up clade D zooxanthellae, which can be thermally tolerant up to 32°C (90°F). Of course, the major downside here is that these larvae have to rely on other nutrient sources until they acquire zooxanthellae. By feeding on their egg yolk initially, and by consuming plankton and taking up dissolved nutrients they can survive without zooxanthellae.
“Transfer of zooxanthellae to offspring via eggs is known as vertical transmission. Uptake of zooxanthellae from the water column is referred to as horizontal transmission.”
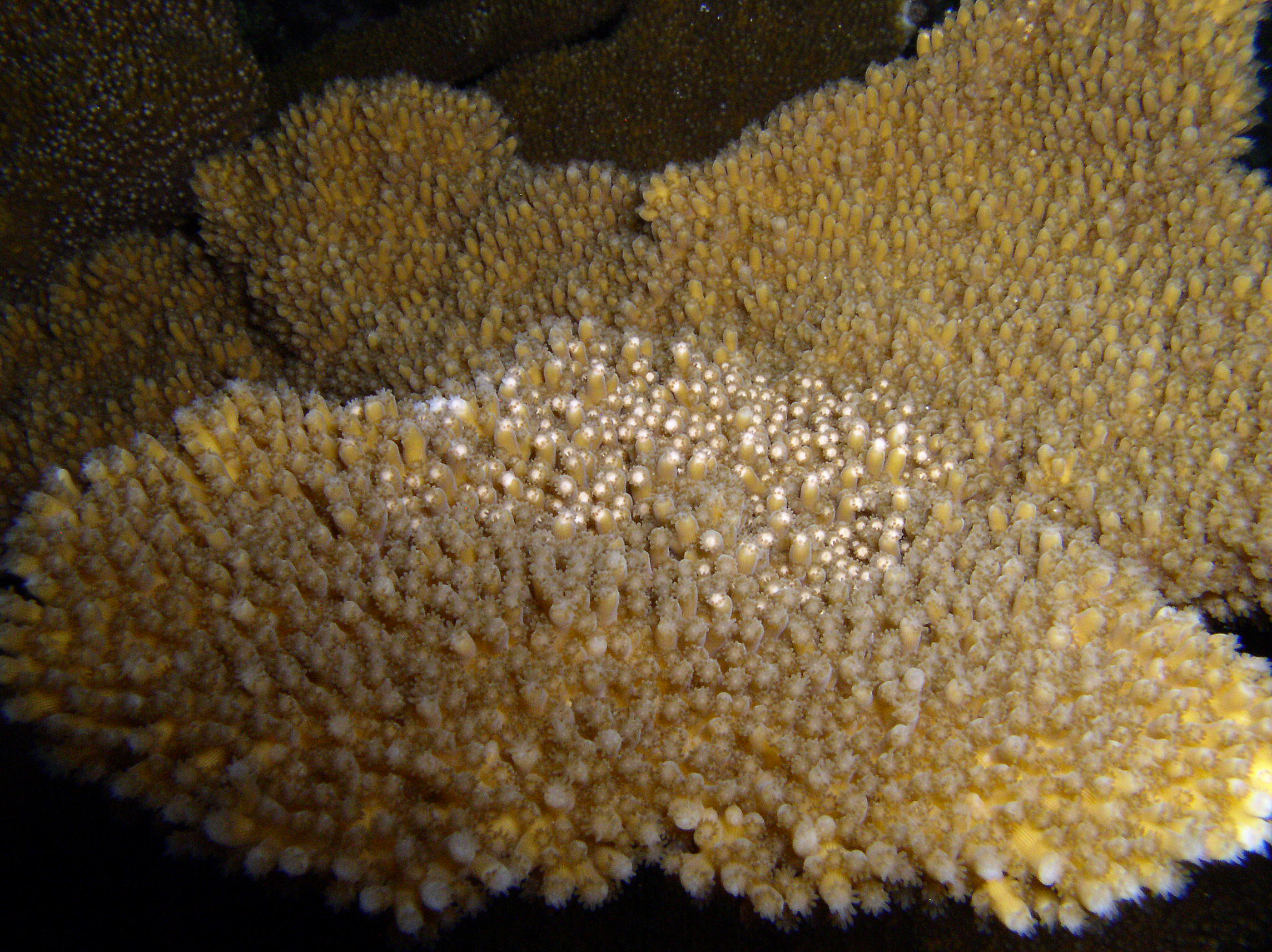
A large Acropora palmata colony during the August 2008 spawning in Puerto Rico. Released egg/sperm bundles can be clearly seen. These are collected by SECORE divers with plankton nets for further rearing and research. Currently, these phenomena are rarely observed in closed aquarium systems (photograph: Ramón Villaverde).
Reproduction in the aquarium
The reproductive biology of corals has major consequences for the success of sexual reproduction in captivity, as especially broadcasting species are highly difficult to reproduce in a closed aquarium. Below, I will discuss some of the challenges, possible solutions and future perspectives of coral reproduction in aquaculture. These challenges involve simulation of environmental cues which stimulate reproduction, providing sufficient nutrition and the use of specialized filtration systems.
Environmental cues for reproduction
Many organisms have adapted to the erratic seasons on the planet, which are especially contrasting at latitudes outside of the Tropics of Cancer and Capricorn. Temperature, photoperiod, irradiance, precipitation and water current are important abiotic factors which fluctuate dramatically over the course of a year. Making use of the optimal season for reproduction is key to the survival of many species.
Many reef animals are dependent on seasonal reproductive cues. When these environmental cues are absent, organisms such as corals will not spawn. This phenomenon has been found to apply to many coral species, especially broadcasting species. The main cues, or triggers for coral reproduction seem to be fluctuations in water temperature, sun- and moonlight, and possibly water movement. The involvement of hormones has also been proposed by scientists, although this remains to be determined.
“The reason why many corals reproduce during the summer months may be because concentrations of plankton are highest, increasing chances of offspring survival.”
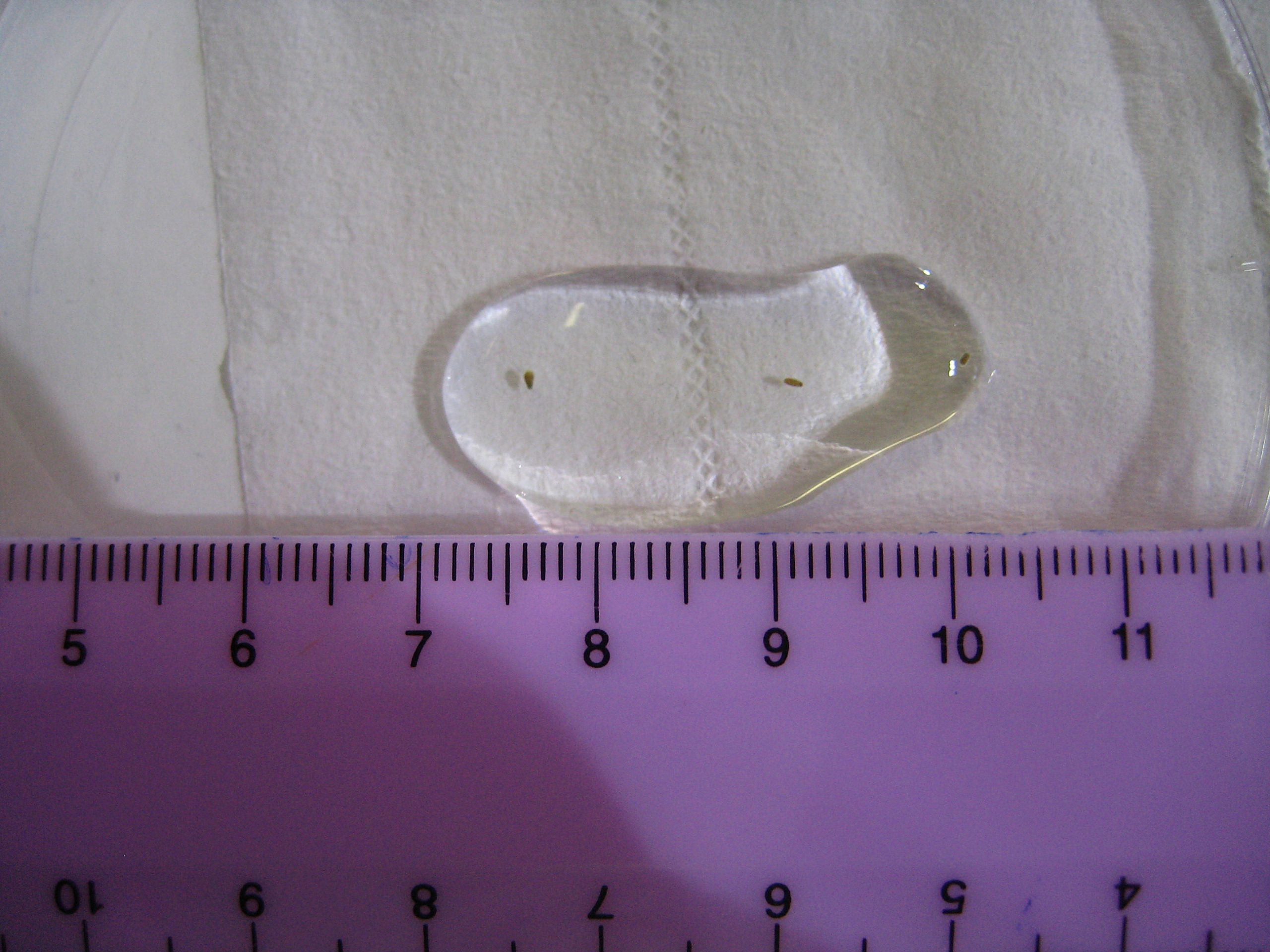
Swimming larvae of Favia fragum, a brooding species. These have been collected in the morning using a plankton filter mesh. The larvae have obtained zooxanthellae through the ovum from which they developed. The scale bar is in centimeters or 0.4 inches (photograph: Tim Wijgerde, Acquario di Genova, Genova, Italy).
Temperature
The average temperature on coral reefs fluctuates annually between roughly 22°C (72°F) and 30°C (86°F), with summer maxima sometimes peaking around 35°C (95°F). This forms a striking contrast with home aquaria, which are usually kept at a constant temperature with aquarium heaters and thermostats. In these aquaria it is basically always “spring”, and therefore a major reproductive trigger is absent. For several species it has been found that gametogenesis, the production of eggs and sperm, accelerates during spring. During the summer, eggs will have matured and become poised to be fertilized. This cycle is displayed by a staggering amount of plant and animal species. Temperature may well be the most critical cue for reproduction. It is of course vital not to let water temperatures exceed the 30°C (86°F) threshold, which would induce thermal bleaching and promote bacterial infections (e.g. Vibrio). The reason why many corals reproduce during the summer months may be because concentrations of plankton are highest during this time, which allows larvae and primary polyps to ingest ample nutrients. This could promote their survival.
Light
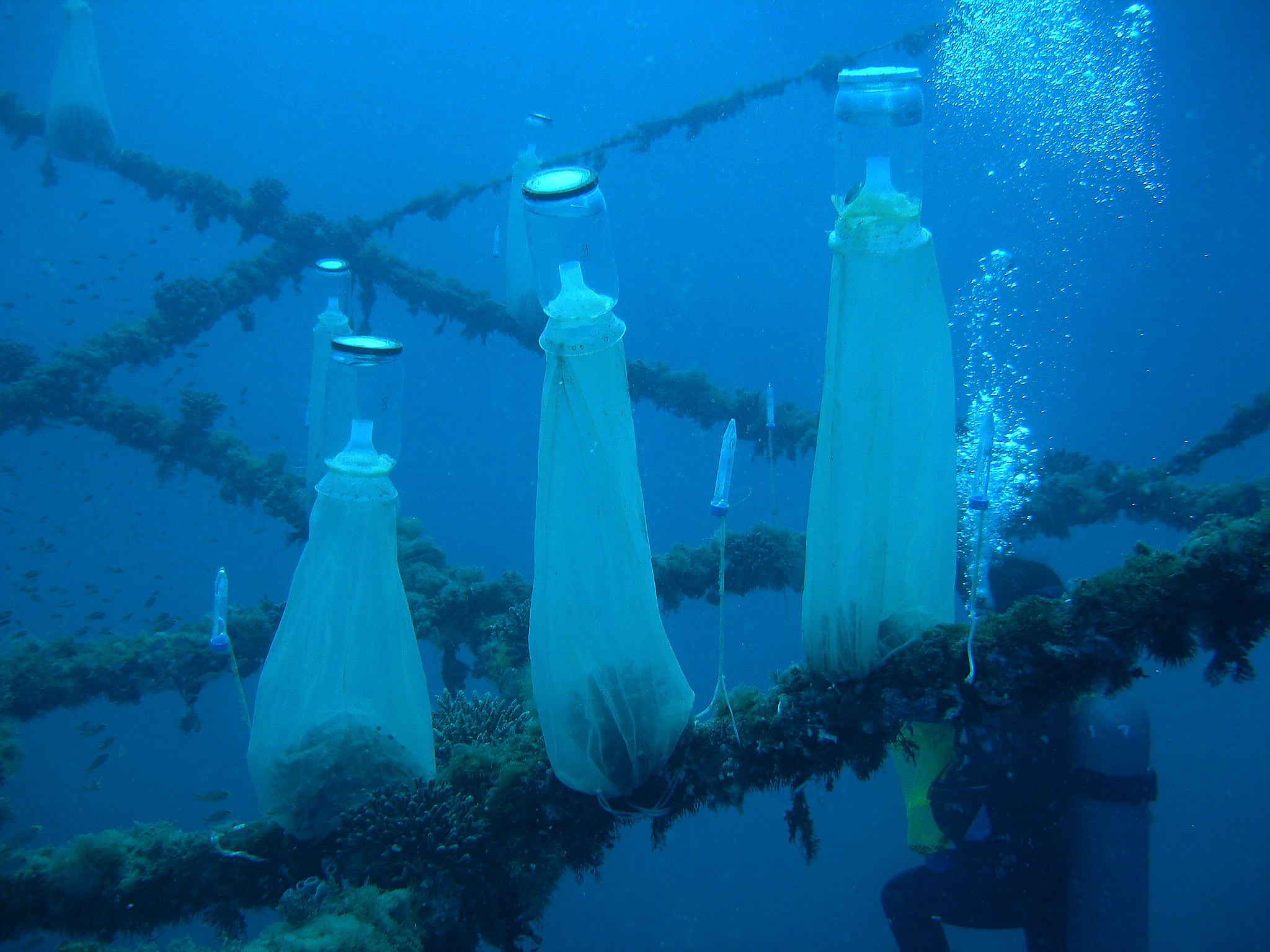
Scientists cover gravid or “pregnant” colonies of Stylophora pistillata with plankton nets in the Gulf of Aqaba, Red Sea. The following morning the collection beakers are harvested, which often contain released larvae (photograph: Dr. Keren-or Amar, Eilat, Israel).
Light is a factor which has been recognised by aquarists as an important cue for years, especially for fish. Light computers and dimmable fixtures nowadays are able to mimic natural photoperiods, and LED lights simulate moonlight. Unfortunately, moonlight is often applied at a constant irradiance level, which means the aquarium is always subjected to a “full moon”. This may not be sufficient to stimulate corals and fish to display their natural reproductive behavior (see water current). It is the fluctuation which makes all the difference, and this should vary between a PAR value of 0.01 mol/m2/s at full moon, and 0 mol/m2/s at new moon. Compare these levels with an average aquarium irradiance of 200-400 E/m2/s, and it becomes clear how dim this light should be. Without a light intensity meter (PAR meter), it is difficult to mimic these exact levels, however. Where temperature should fluctuate over a course of a year, moonlight should follow a cycle of four weeks. In practical terms, this means the moonlights should increase in irradiance level for two weeks, after which they should decrease in strength for another two weeks.
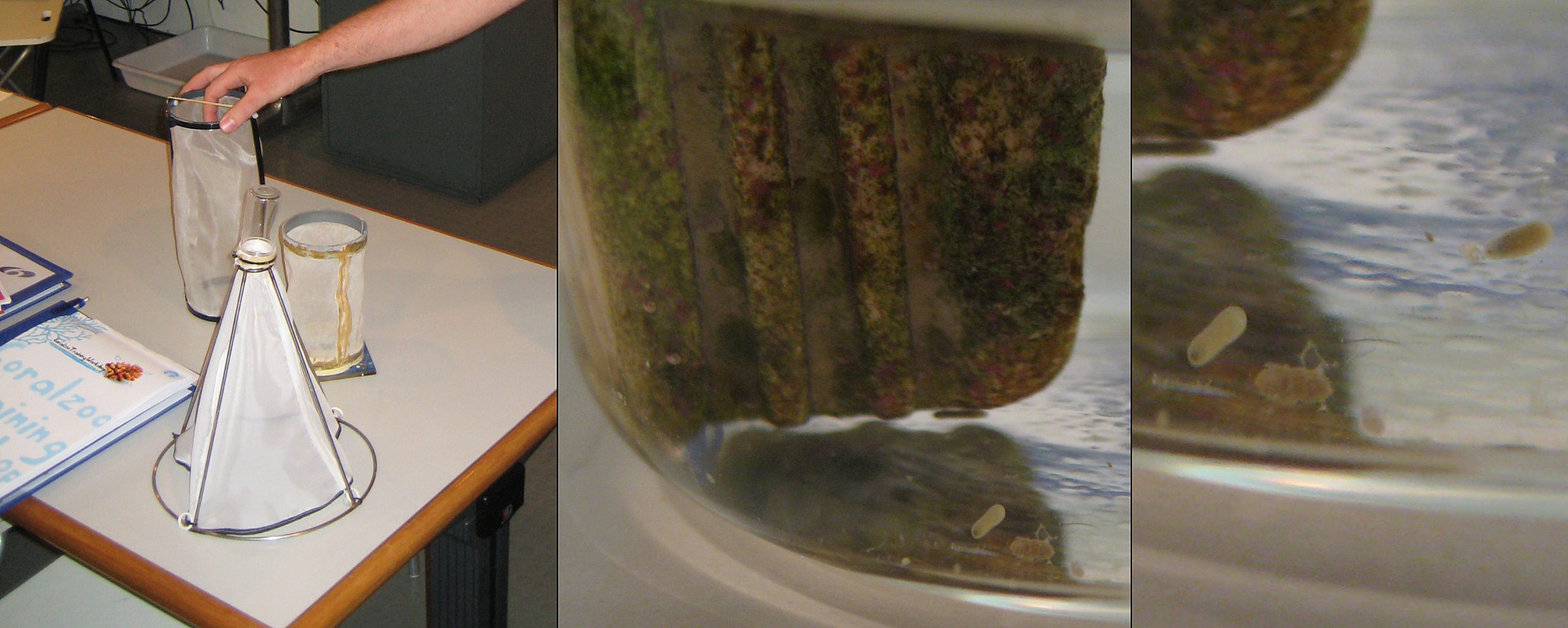
Larvae of brooding species such as Favia fragum can be collected easily by placing plankton nets over gravid colonies, which rise out of the water or use collection beakers (left). In the morning, larvae will have collected at the water surface or inside the beaker, after which they can be transferred to settling tanks with a pipette. These tanks may contain ceramic tiles on which a biofilm has already developed (middle). The larvae (middle and right) will start looking for suitable places to settle (photographs: Tim Wijgerde, Acquario di Genova, Genova, Italy).
Due to the daily rhythm of an aquarist, the aquarium is mostly viewed during the day or evening. At night, however, there is also much to be seen; corals expand their tentacles to capture particles, and release their gametes or larvae (save for exceptions such as Pocillopora meandrina in Hawaii). The reason why corals do this could be to reduce predation on their offspring, as less predators are active at night. This poses yet another difficulty for aquarists, as gametes and larvae are readily trapped by filtration systems. In addition, it is often desirable to rear offspring in separate aquaria. This problem may be solved by placing plankton nets or filtration mesh over suspected gravid colonies. This can be done in the aquarium as well as in situ, such as in the Gulf of Eilat where researchers place plankton nets over gravid Stylophora pistillata colonies. Collection beakers are harvested the following morning, which often contain released larvae of this brooding species. S. pistillata is known to release larvae mainly during springtime (from April to May), but other species such as the Caribbean Favia fragum may release larvae all year round.
“The release of gametes or larvae usually takes place during the night. Corals possibly do this to reduce predation on offspring.”
Another possibility is reversing the photocycle of the aquarium, allowing for gamete collection during the day. It may take a while before corals acclimatize to a new photocycle, however there is anecdotal evidence which suggests corals are able to depart from their natural rhythm. As an example, aquarists regularly see corals expanding their tentacles during the day as a response to food addition.
Light intensity and photoperiod also fluctuate over the course of a year, especially on reefs which are located outside of the tropics. With an advanced light computer, diurnal cycles, and changes in photoperiod and irradiance throughout the year can be simulated. Some data indicate that these factors may also affect coral spawning.
Water current
Closely linked to moonlight are the tides, which strongly influence water current velocity. In the aquarium, water current cycles should follow a two-week cycle, i.e. half the cycle length of the moon. This is because on the reef, water currents are strongest during both new and full moon. This means that flow controllers should ideally increase water flow rate over a one-week period, followed by another week of decreasing current. It is important that moonlight and water current are properly synchronized, in such a way that water flow rate peaks at full moon as well as new moon, and is lowest in between new and full moon. But why are moonlight and water current so important to corals, in terms of sexual reproduction?
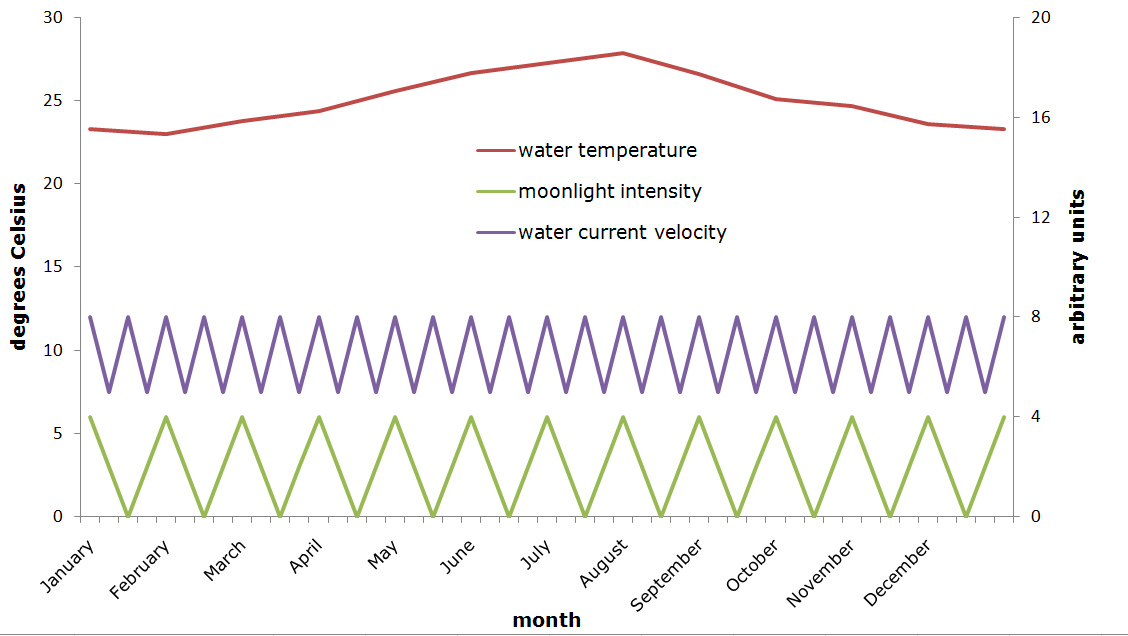
A suggestion for fluctuations in water temperature, moonlight intensity and water current velocity in the aquarium over a one-year period. Temperature is shown in degrees Celsius on the left y-axis, moonlight intensity and water current velocity are displayed in arbitrary units on the right y-axis. It is difficult to quantify these last two values without sophisticated equipment, however the essential point here is that strong fluctuations occur (graph: Tim Wijgerde).
For years, it has been known that many broadcasting species reproduce about a week after full moon, during the evening or night. This is possibly because in this period, water currents are lowest. A week after full moon, during the last quarter, the sun and moon partially cancel out each other’s force of gravity, thereby decreasing tidal forces. During this moment, known as neap tide, chances of egg fertilization by sperm may be highest as less dilution of gametes occurs. In 2007, scientists discovered that corals are able to detect blue light, which triggers the production of so-called cryptochromes in coral cells. These proteins are light sensitive, and are known to regulate Circadian rhythms (24 hour rhythms). Due to increased production of these proteins by coral tissue, changes in the reproductive state of coral polyps may occur, such as the onset of final gamete and larvae maturation, and subsequent release into the water.
When fluctuations in water temperature, (moon)light and water current are applied to home aquaria, the natural reproductive cycles of marine organisms may be maintained. This will, in a number of cases, lead to the reproduction of fishes, corals and other invertebrates.
Nutrition
Coral nutrition has received much attention by aquarists over the last few years; the view that only the fish require feeding, and that corals simply need strong lighting is losing ground. Scientific studies show that in order to grow well, corals need to obtain nutrients from plankton and dissolved compounds to complement photosynthesis. The same may be true for sexual reproduction, which could be hampered by a lack of external nutrient sources.
As described above, small or weakened Fungiid corals have been found to have active male gonads only, possibly because they have too little energy reserves to produce eggs. Local or aquarium populations suffering from male dominance cannot reproduce properly, as both gamete types are required simultaneously for fertilization. Parthenogenic and simultaneous hermaphroditic corals may also have difficulty with maintaining oogenesis (egg production) during times of physiological stress.
In the aquarium, plankton can be dosed on a daily basis to provide corals with sufficient food to maintain fertility. Artemia nauplii, rotifers (Brachionus plicatilis), copepods (e.g. Tigriopus californicus, Parvocalanus crassirostris, Acartia tonsa) and phytoplankton products (e.g. Phyto Feast) are suitable food sources for many corals.
Filtration
Next to providing reproductive cues and sufficient food, a third important factor is the type of filtration used. Aquarium filters can be a major hurdle for captive sexual reproduction, as they are usually not particularly plankton-friendly. The most well-known filtration device for marine aquaria is the protein skimmer, or foam fractionator. This device allows aquarists to maintain an aquarium with relative ease. By mixing seawater and air, organic molecules and fine particles are removed from the water. This reduces microbial breakdown of food and animal wastes, thereby inhibiting the accumulation of nitrate, phosphate and various organic compounds. The major disadvantage of this method is that plankton, which includes the gametes and larvae of many animals, is removed as well. Biofilters and sand filters also trap eggs and larvae. Aquarium pumps also have a destructive effect on planktonic life, although no real alternatives for the home market are currently available. This problem is so fundamental that active removal or separation of gametes and larvae is required for most species. This can be done with plankton nets or kreisels.
Plankton-friendly alternatives for protein skimmers and biofilters are available, which include denitrification systems. These systems use sand beds, in which bacteria break down various forms of inorganic nitrogen, via (de)nitrification. In the hypoxic lower zone of the sand bed, heterotrophic bacteria use nitrate (NO3–) as an oxidator instead of oxygen (O2) to oxidate carbon sources. Phosphates are converted into bacterial biomass, and precipitated as apatite when calcium carbonate is used as a substrate. Denitrification systems were more common in the 1980’s, before protein skimmers became popular. These systems are no longer widely used, as they can be unpredictable. A problem which can present itself after several years is congestion of the sand bed. This results in patches which are completely anoxic. Under these conditions, bacteria reduce sulfate (SO42-) instead of nitrate as this is energetically favorable. This leads to the production of toxic hydrogen sulfide gas (H2S), with sometimes dire consequences for aquarium life.
A system which is similar to the DSB has existed for many years, and was developed by Prof. Jean Jaubert. This system uses a plenum, which is a layer of stagnant water situated under a screen which supports the substrate. Ideally, the system is stocked with cirratulid worms, which consume detrital matter precipitating on top of the sand bed. After ingestion, they transfer fecal matter to the hypoxic layer. Here, bacteria can use the carbon present in the worm’s feces to reduce nitrate. Jaubert has obtained impressive results with his system at the Oceanographic Museum of Monaco.
Another type of denitrification system is DyMiCo, short for Dynamic Mineral Control. This is a computer-controlled filtration system, which makes use of several important innovations. With a pump, active communication between the water column and the sand bed is established. This allows for a more efficient breakdown of nitrate, as the system is no longer dependent on diffusion processes. A carbon source is also injected into the substrate, ensuring nitrate reduction is not carbon-limited. Finally, redox and pH probes in the sand bed allow for careful monitoring of the system. Redox values between -300 and -100 mV are important for high rates of denitrification, and this value can be manipulated by controlling the amount of injected carbon and flow through the sand bed. When the pH level of the sand bed is kept at 6.5 – 7 with carbon dioxide (CO2), it also functions as a powerful calcium reactor.
Whatever filtration methods are used, the essence is that these should be plankton-friendly if sexual coral reproduction of many species is to be successful without intervention, such as with plankton nets. It is clear that sexual coral reproduction is not an easy task, as it requires detailed knowledge, dedication and time.
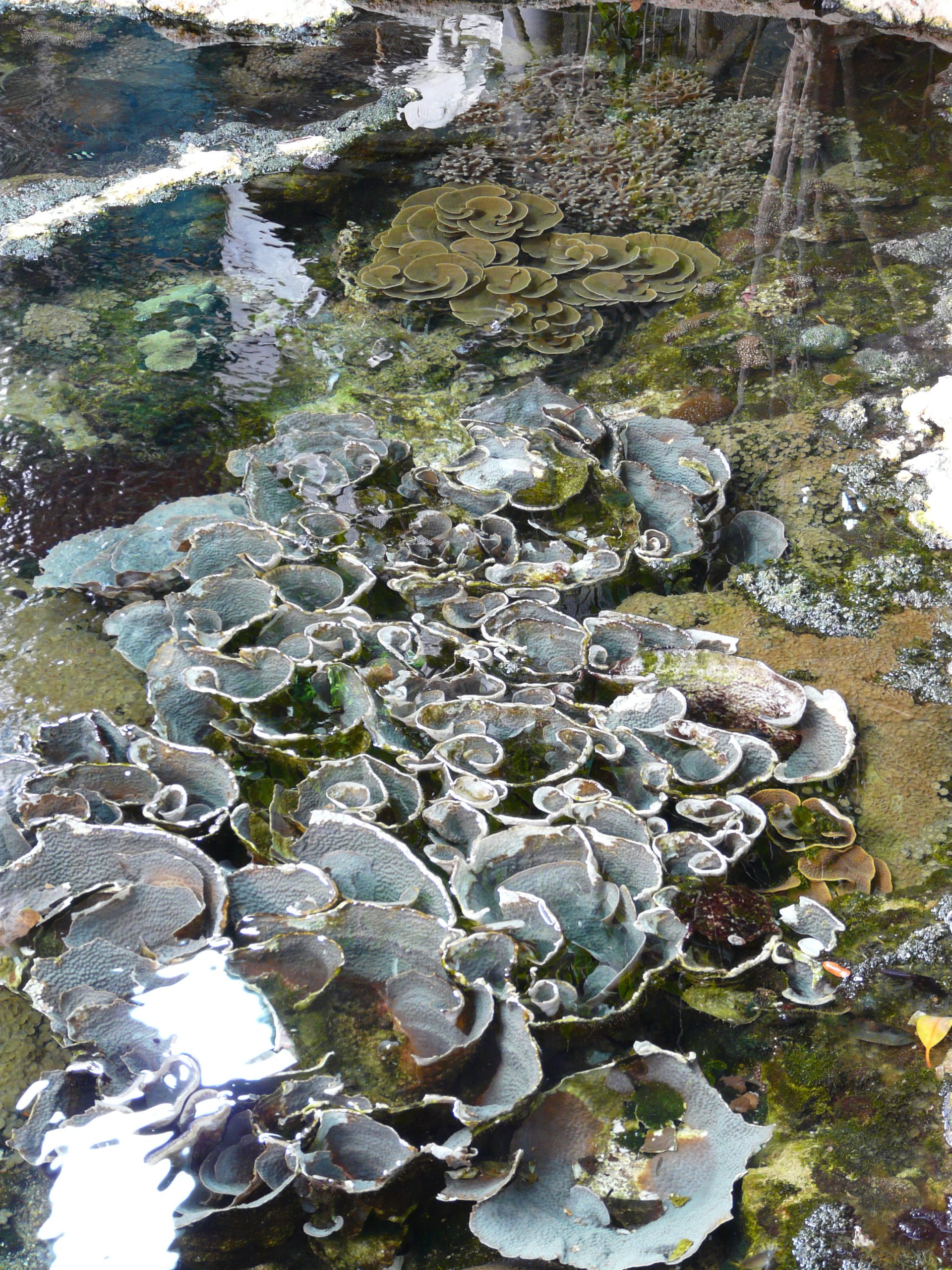
Echinopora lamellosa, Montipora sp. and Seriatopora caliendrum colonies which have reached impressive sizes, growing in an artificial lagoon. Many coral species only reproduce once they have attained specific minimum sizes (photograph: Tim Wijgerde, NAUSICAA, Boulogne-sur-Mer, France).
Colony size and fertility
In the aquarium trade, people often speak about “parent colonies”, and “frags”. The parent colony usually has become too large for the home aquarium, after which it is pruned. Often, these colonies are nowhere near their natural maximum sizes. For example, Acropora, Montipora and Euphyllia colonies can grow up to several meters in diameter. When colonies are maintained too small, they will not invest energy into reproduction. Only at a specific minimum size, they will start producing eggs and sperm. This principle applies to many plants and animals, which first invest energy into growth before reproducing. For example, an apple tree will first grow up to a specific size before initiating fruiting. In biology, these two phases are called the vegetative and generative phases, respectively.
This phenomenon may prevent small colonies from spawning in the aquarium, as they are juveniles that still invest most energy into growth. For Acropora formosa, for example, it has been found that branches of fertile colonies stop sexually reproducing when removed at a size of 5 cm (2 inch) in length. When 20 cm (8 inch) fragments are removed, they continue egg development. Such experiments suggest that breeding colonies should be allowed to grow to larger sizes, to allow them to maintain egg and sperm production.
Exceptions exist, such as the species Pocillopora damicornis, Favia fragum and Tubastrea coccinea. These species already reproduce at very small colony sizes. These are brooding corals, which in addition makes it less difficult to sexually reproduce them.
Additional obstacles
Mating pairs
Many gonochoric coral species exist, with separate male and female individuals. This applies to roughly 25% of all coral species, and this poses a challenge for aquarists. For example, species from the genera Turbinaria and Dendrophyllia (family Dendrophylliidae) will have to be maintained as both sexes. For broadcasters such as Turbinaria sp., gametes will also have to be released in concert.
Settling of larvae
If inducing coral spawning and gamete/larvae collection have been successful, the next challenge is to have the offspring attach, or settle, onto a substrate. If this occurs in the aquarium, which is common for brooding species, this process can be left to nature. In some cases however, it is desirable to collect gametes or larvae, which is especially true for broadcasters. The eggs can be fertilized by mixing them with sperm, with an incubation time of thirty minutes being sufficient for at least some Acropora species. Next, the eggs can be transferred to small aquaria which are gently aerated with air tubes.
Kreisel systems are also ideal, which allows for gentle stirring of larvae. Excess sperm cells will have to be removed within several hours after fertilization, as these will negatively affect water quality and oxygen levels. Depending on species and temperature, embryos will develop into larvae within one to four days. As soon as the swimming stage has been reached, the larvae will settle within several days to weeks. Brooding species release large larvae with short competency periods, allowing them to settle within a day. Larvae which develop from eggs externally often have long competency periods, allowing them to settle even after several weeks, in some cases. To survive for this long, these larvae develop mouths during their planktonic stage, allowing them to feed on particles and ingest zooxanthellae.
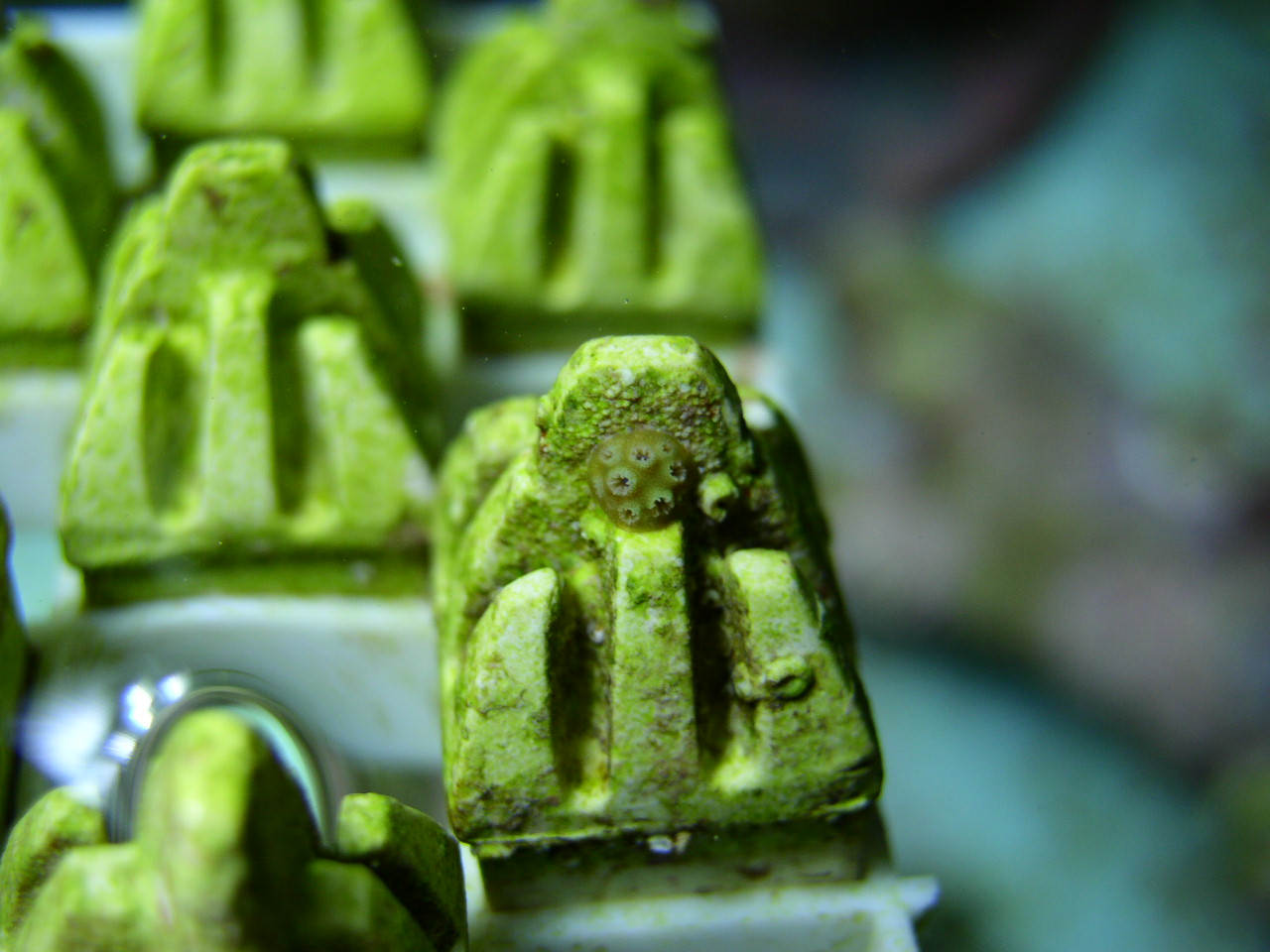
Ceramic tiles with grooves form an ideal substrate for coral larvae after preconditioning in an aquarium for several weeks. A biofilm of bacteria and algae may provide necessary cues for larvae to settle (photograph: A three month-old Acropora palmata colony, courtesy of Mitch Carl, Omaha’s Henry Doorly Zoo, Omaha, USA).
For larval settlement, suitable substrates are required, such as ceramic tiles. These are first conditioned in the aquarium, during which a biofilm of bacteria and calcareous algae develops over the tiles. Without this vital step, the larvae will not settle. The biofilm may provide necessary settling cues for the larvae, such as signal molecules released by bacteria or algae. Bacteria are known to be part of the coral holobiont, the complete assemblage of the coral and its symbiotic organisms including zooxanthellae and bacteria, and may provide the coral with immune defense against other harmful microorganisms. An example of such harmful organisms are bacteria from the Vibrio genus, which have been linked to coral diseases such as white band syndrome and yellow blotch.
Zooxanthellae uptake
As described above, about 15% of all coral species receives zooxanthellae from the parent colony, a process known as vertical transmission. The majority of all species, however, has to acquire these symbiotic dinoflagellates early in life each generation. This uptake occurs during the larval stage, or after metamorphosis at the primary polyp stage. Several species are not yet competent to take up zooxanthellae until after metamorphosis into a primary polyp, as their larvae do not develop an oral pore during the planktonic stage. This is true for the Caribbean Elkhorn coral, Acropora palmata, a species which is critically endangered. Primary polyps of this species can be inoculated by adding concentrated zooxanthellae cultures to the aquarium water, which have been previously isolated from coral tissue. They may also be able to take up zooxanthellae which have been expelled by other corals in mucus, a process which may occur in the wild. Knowledge of the onset of the coral-zooxanthellae symbiosis for each species is important to promote survival chances for larvae or primary polyps.
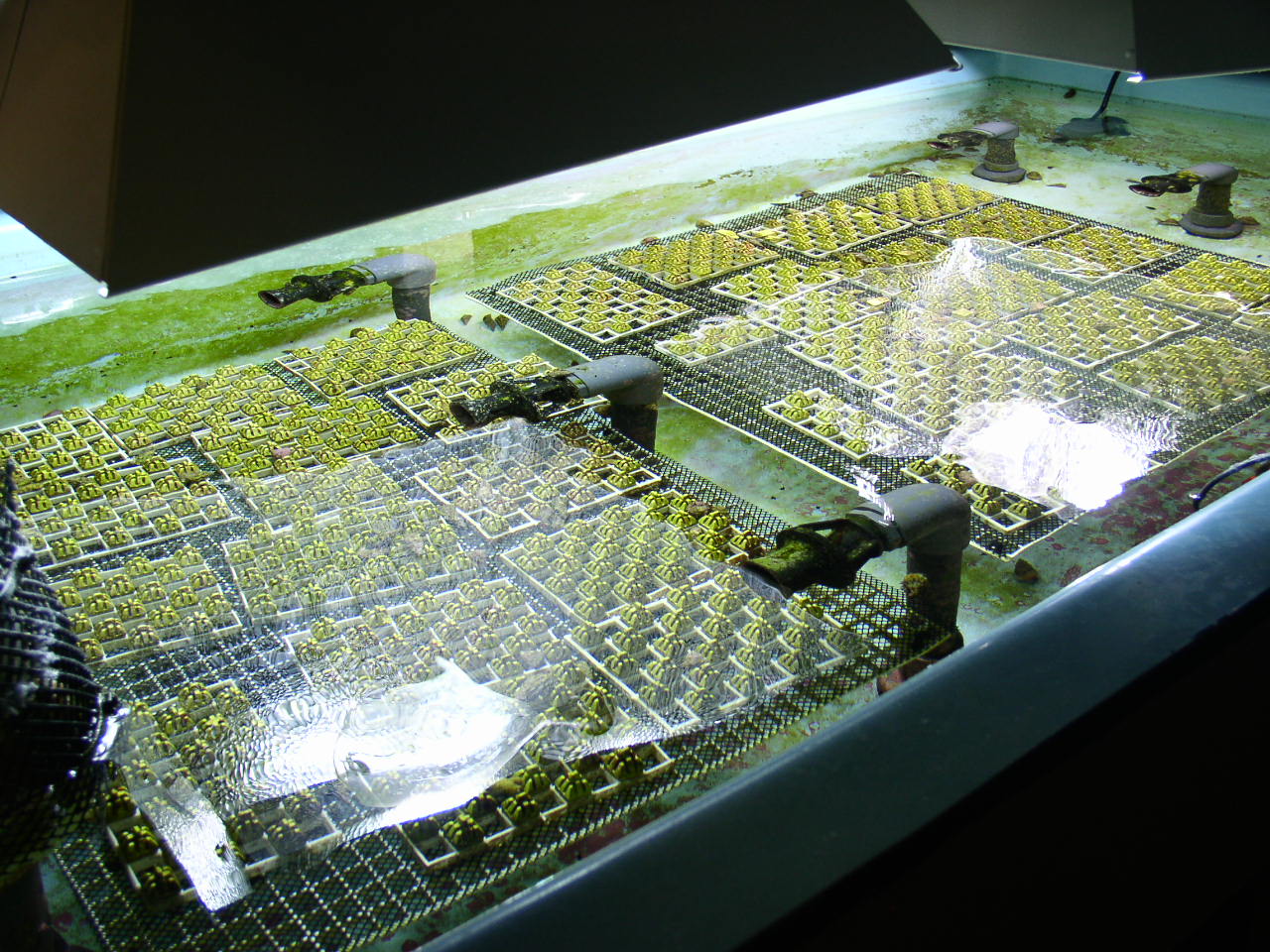
Setup for rearing young A. palmata colonies. Only about 10% of the larvae may settle onto a tile and metamorphose into a primary polyp. Young colonies from this species grow very slowly, and should be kept free of fouling organisms at all times (photograph: Mitch Carl, Omaha’s Henry Doorly Zoo, Omaha, USA).
Rearing offspring
Rearing young colonies can be a difficult task, as they are quite sensitive to overgrowth by algae and other organisms. Offspring should therefore be kept clean, by introducing snails, hermit crabs and herbivorous fishes, and by regular cleaning of the tiles.
Degrees of difficulty: an overview
Although sexual coral reproduction is not an easy task, several degrees of difficulty exist. This mostly depends on coral sexuality (parthenogenesis, hermaphroditism or gonochorism) and the mode in which ova are fertilized (broadcasting or brooding). The easiest species are parthenogenic and hermaphroditic brooders. For these species, only one colony is required, as in many cases selfing may occur. Egg cells are also internally fertilized and developed, which circumvents the problems of removal by mechanical filtration. Furthermore, larvae released by brooding species often display negative buoyancy and short competency periods, which again reduces filtration-related losses. When plankton nets are placed over brooding colonies, larvae can often be collected the following morning for rearing in separate aquaria.
“The development of a closed system aimed at large-scale sexual coral reproduction would be a major step in the field of marine aquaculture.”
The most difficult species are hermaphroditic and gonochoric broadcast spawners. These corals require environmental stimuli such as strong fluctuations in water temperature, (moon)light and possibly water movement. For gonochoric species, colonies of both sexes are also required. Finally, released gametes will have to be collected before they are trapped by filtration systems, at least when conventional aquarium systems are utilized. Table 1 provides an overview of the various categories and their degrees of difficulty.
Table 1: Coral species categorized in degrees of sexual reproduction difficulty. Only several species per group are presented (based on Riddle, 2008 and Fadlallah, 1983).
1: classification from A: easy to G: highly difficult
2: sufficient nutrition and light required for maintaining oogenesis
3: usage of plankton nets required if protein skimmers and biofilters are applied, to prevent mechanical removal of gametes and larvae
4: environmental cues such as fluctuations in water temperature, (moon)light and water current required
5: a minimum of two colonies required
6: two sexes required
The future
The field of coral aquaculture has made significant advances over the last decades, with the advent of protein skimmers, calcium reactors, powerful pumps, high quality synthetic sea salts and a thorough knowledge of water chemistry. The husbandry of exotic species always seems to follow three distinct phases; long-term survival, growth and finally sexual reproduction, and the same may be true for corals. The first two phases have been successfully achieved for many reef species, save for azooxanthellate corals such as Dendronephthya sp., feather stars, tunicates, many bivalves and sponges. However, when the final phase is considered, sexual reproduction, it becomes clear that coral aquaculture is still faced with important challenges.
Sexual reproduction of corals is a time-consuming endeavor which is not within reach of most aquarists. Nevertheless, for the average hobbyist, there still is room for much improvement. Providing environmental reproductive cues and using plankton-friendly filtration systems may yield new results. Zoos, public aquaria, universities and research institutes have made important contributions to sexual coral reproduction, with projects such as SECORE (www.secore.org) and CORALZOO (www.coralzoo.org). In Japan, much has been done in this field as well. For example, coral spawnings at the Okinawa Churaumi Aquarium occur regularly. Coral larvae have also been used around Okinawa to restore reef sections, by isolating large numbers within plankton nets placed over damaged parts of the reef.
Although impressive and pioneering, these efforts all depend on field work or open aquarium systems. The development of a closed system aimed at large-scale sexual reproduction would be a major step forward in the field of coral aquaculture. As science and technology continue to evolve, it is quite possible that sexual coral reproduction will become a standard practice in the future.
For a comprehensive list of stony corals and their reproduction methods, the reader is referred to the Coral Reproduction series by Dana Riddle in Advanced Aquarist (2008).
Acknowledgements
The author wishes to thank Dana Riddle for critically evaluating and improving the manuscript.
References
- Atkinson S. & M.J. Atkinson, 1992. Detection of estradiol-17ß during a mass coral spawn. Coral Reefs 11:33-35
- Elahi R. and P.J. Edmunds, 2007. Tissue Age Affects Calcification in the Scleractinian Coral Madracis mirabilis, Biol. Bull. 212:20-28
- Elyakova L.A., N.M. Shevchenko, S.M. Avaeva, 1981. A comparative study of carbohydrase activities in marine invertebrates, Comp. Biochem Physiol. 69B:905-908
- Fabricius K.E., G. Yahel & A. Genin, 1995. Herbivory in asymbiotic soft corals, Science 268:90-93
- Fadlallah Y.H., 1983. Sexual reproduction, development and larval biology in scleractinian corals, Coral Reefs 2:129-150
- Houlbrèque F., C. Ferrier-Pagès, 2009. Heterotrophy in tropical scleractinian corals, Biological Reviews 84:1-17
- Iguchi A., M. Morita, Y. Nakajima, A. Nishikawa and D. Miller, 2009. In vitro fertilization efficiency in coral Acropora digitifera, Zygote 17:225-227
- Jokiel P.L., R.Y. Ito and P.M. Liu, 1985. Night irradiance and synchronization of lunar release of planula larvae in the reef coral Pocillopora damicornis, Marine Biology 88:167-174
- Levy, O., L. Appelbaum, W. Leggat, Y. Gothlif, D.C. Hayward, D.J. Miller and O. Hoegh-Guldberg, 2007. Light-Responsive Cryptochromes from a Simple Multicellular Animal, the Coral Acropora millepora, Science 318:467-470
- Loya Y. and K. Sakai, 2008. Bidirectional sex change in mushroom stony corals, Proc. R. Soc. B. 275:2335-2343
- Okubo, N., T. Motokawa and M. Omori, 2007. When fragmented coral spawn? Effect of size and timing on survivorship and fecundity of fragmentation in Acropora formosa, Marine Biology 151:353-363
- Permata W.D. and M. Hidaka, 2005. Ontogenic changes in the capacity of the coral Pocillopora damicornis to originate branches, Zoological Science 22:1197-1203
- Petersen D., M. Laterveer and H. Schuhmacher, 2005. Innovative substrate tiles to spatially control larval settlement in coral culture, Marine Biology 146:937-942
- Riddle D., 2008. Feature Article: Coral Reproduction, Part Three: Stony Coral Sexuality, Reproduction Modes, Puberty Size, Sex Ratios and Life Spans, Advanced Aquarist’s Online Magazine (www.advancedaquarist.com) 7(9)
- Riddle, D., 2008. Feature Article: Coral Reproduction, Part Three: Stony Coral Sexuality, Reproduction Modes, Puberty Size, Sex Ratios and Life Spans, Advanced Aquarist’s Online Magazine (www.advancedaquarist.com), 7(9)
- Rinkevich B. and Y. Loya, Senescence and dying signals in a reef building coral, 1984. Experientia 42:320-322
- Rinkevich B., 2008, personal communication
- Rosenberg E., O. Koren, L. Reshef, R. Efrony and I. Zilber-Rosenberg, 2007. The role of microorganisms in coral health, disease and evolution, Nature Reviews Microbiology 5:355-362
- Stoddart, J.A., 1983. Asexual production of planulae in the coral Pocillopora darnicornis. Mar Biol 76:279-284
- Tchernov D., M.Y. Gorbunov, C. de Vargas, S.N. Yadav, A.J. Milligan, M. Häggblom and P.G. Falkowski, 2004. Membrane lipids of symbiotic algae are diagnostic of sensitivity to thermal bleaching in corals, Prod. Natl. Acad. Scienc. USA 101:13531-13535
- van Woesik R., 2008. Solar Insolation Drives Reproductive Schedules Of Reef Corals: A Long Road in The Search For Proximate And Ultimate Cues, 11th ICRS, Fort Lauderdale, USA
- Wabnitz C., M. Taylor, E. Green and T. Razak, 2003. From ocean to aquarium: The global trade in marine ornamental species, UNEP-WCMC Biodiversity Series No 17, pp 65, ISBN: 92-807-2363-4
- Weis V., 2008, personal communication
- Wijgerde T., F. Houlbrèque and C. Ferrier-Pagès, 2009. How corals feed, Coral Science (www.coralscience.org)
- Yakovleva I.M., A.H. Baird, H.H. Yamamoto, R. Bhagooli, M. Nonaka, M. Hidaka, 2009. Algal symbionts increase oxidative damage and death in coral larvae at high temperatures, Mar. Ecol. Prog. Ser. 378:105-112
0 Comments